10.5 Disorders of fluids, electrolytes and acid–base
Physiology
There are many physiological differences between adults and infants, and a few specific features that must be taken into account in managing fluid therapy. Infants have greater total body water, up to 70% of body weight compared with 60% in adults, the extra fluid being mostly extracellular, 30% of body weight compared with 20%1,2 (Fig. 10.5.1). The ionic composition of intracellular and extracellular fluid is shown in Table 10.5.1. Small children drink more to accommodate a higher metabolic rate and excrete a higher solute load, and thus urine volume is greater.
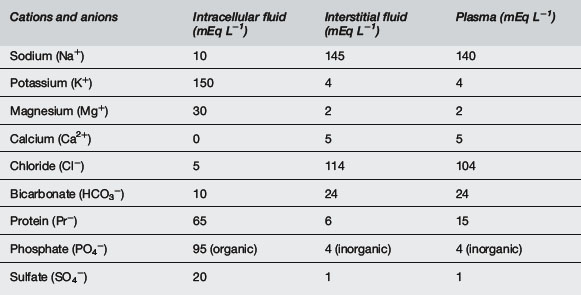
The following physiological differences apply to children:1–3
Clinical assessment
Capillary refill times were studied in 32 children, aged 1–26 weeks, admitted to hospital with dehydration.4 The authors recommended a cut-off value of 2 seconds, below which minimal or no dehydration exists. A capillary refill time of 2–3 seconds suggests a 50–100 mL kg−1 water deficit; 3–4 seconds 100–120 mL kg−1, and over 4 seconds >150 mL kg−1. However, ambient temperature affects capillary return.5
In children less than 4 years old clinicians overestimate the degree of dehydration by 3.2%.6 Other studies have suggested that the sensitivity of clinical examination for diagnosing dehydration is 74, 33 and 70% for mild, moderate and severe dehydration respectively.7 See Tables 10.5.2 and 10.5.3 for clinical signs associated with dehydration and electrolyte imbalance.8
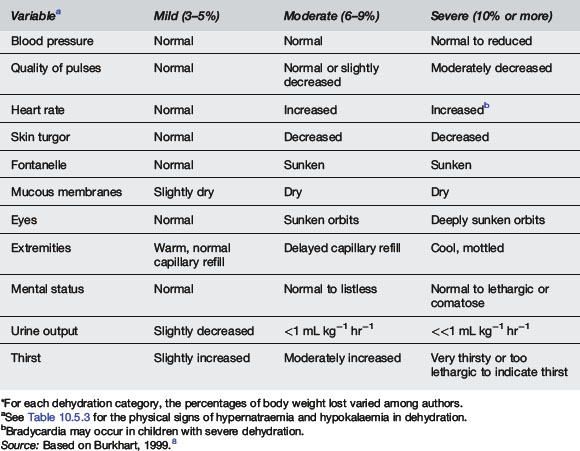
Hypernatraemia |
Cutaneous signs |
Warm, ‘doughy’ texture |
Possibly decreased skin-fold tenting in severe dehydration, thereby giving appearance of lower level of dehydration |
Neurological signs |
Hypertonia |
Hyperreflexia |
Lethargy common, but marked irritability |
when touched |
Hypokalaemia |
Weakness |
Ileus with abdominal distension |
Cardiac arrhythmias |
Source: Based on Burkhart, 1999.8
Plasma bicarbonate concentration may be the single most useful laboratory test; a level less than 17 mmol L−1 indicates moderate or severe dehydration. Addition of this to the clinical scale improves the sensitivity of diagnosing moderate and severe dehydration to 90 and 100% respectively. Plasma bicarbonate was a better predictor than plasma urea and creatinine.7
Haemorrhagic shock
Clinical signs are of value in assessing degree of haemorrhage.9 See Table 10.5.4. Hypotension is a preterminal sign.
Class of haemorrhage | Blood volume lost (%) | Signs |
---|---|---|
I | <15 | Minimal, slight tachycardia |
II | 15–30 | Tachycardia, tachypnoea, diminished pulse pressure, systolic BP unchanged, prolonged capillary refill, minimal decrease in urine output, anxiety |
III | 30–40 | Tachycardia, tachypnoea, decreased BP, decreased urine output, mental status changes |
IV | >40 | Hypotension, anuria, loss of consciousness |
Source: Based on Morgan and O’Neill,1998.9
Investigations
Plasma osmolality can be calculated as well as measured in order to detect an osmolar gap.
Treatment
Replacement of circulating volume
Also called volume resuscitation, this is an urgent priority in any cause of hypovolaemic shock, e.g. haemorrhage, sepsis, burns, anaphylaxis and dehydration. It requires isotonic fluids (Table 10.5.5). Hypotonic fluids are inappropriate. Crystalloids are inexpensive and readily available. Colloids have a theoretical advantage of increasing the colloid oncotic pressure of plasma, thus helping to maintain fluid in the vascular space. However, in a capillary leak syndrome such as septic shock, the colloid may pass into the interstitial space. In practice, albumin in saline solution has been tested against 0.9% saline in adult intensive care patients. There was no difference in mortality or intensive care unit (ICU) stay between the two therapies. More saline was administered than albumin (ratio 1.38:1), suggesting that albumin may be superior in patients where excessive water administration may be harmful, e.g. pulmonary oedema, pulmonary hypertension and encephalopathy. There was a suggestion that albumin may be superior in sepsis but inferior in traumatic brain injury.10 A problem with saline is that it has no bicarbonate and relatively high chloride, so it can lead to hyperchloraemic acidosis. Hartmann’s and Ringer’s solutions are more physiological, containing buffer and calcium.10–12
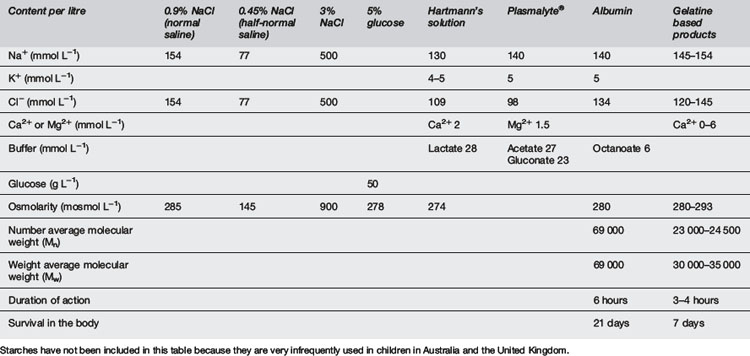
An initial bolus or boluses of 20 mL kg−1 to treat shock may be part of this initial fluid deficit.
How much fluid?
Thus a bolus dose of 20 mL kg−1 is a logical bolus dose of fluid with which to begin resuscitation.
Thus in dehydration repeated boluses may be necessary. Give a bolus, wait 10 minutes and reassess the patient again. Do not wait 10 minutes if it is clear that more than 20 mL kg−1 will be required.13–15
In severe sepsis such as meningococcaemia, 80–100 mL kg−1 may be required. Good evidence exists that early and vigorous resuscitation improves morbidity and mortality in paediatric sepsis and meningococcaemia.16 The volume and the rapidity of resuscitation seems to be more important than the type of fluid used.17–19
Investigation and management of fluids in different conditions
Oral rehydration either by mouth or nasogastric tube is the method of choice for rehydration unless shock exists. It is safe, cheap and effective. It can be effective even in the case of vomiting.21–26
Recommendations for oral and intravenous hydration in gastroenteritis as given by the American Academy of Pediatrics are contained in Table 10.5.7. Oral rehydration should occur with one of the recommended oral rehydration solutions (Table 10.5.8). No evidence exists to show that one formula is more effective than another.22 These fluids resemble 0.45% saline and 2.5% glucose with added potassium, a useful intravenous solution in this condition.8
∗ These recommendations cover children from 1 month to 5 years of age with no pre-existing comorbid conditions who live in developed countries. Specifically excluded are children who have diarrhoea lasting more than 10 days, diarrhoea associated with failure to thrive and/or vomiting in the absence of diarrhoea. If the physician is unsure of the dehydration category for a specific patient, the therapy for the more severe category should be used.
a The diet should emphasise complex carbohydrates, lean meats, yogurt, fruits and vegetables. Fatty foods, and foods and fluids that are high in simple sugars should be avoided.
b Replacement of ongoing fluid losses should include an amount for the estimated volume of emesis and 10 mL kg−1 for each diarrhoeal stool.
Source: Based on Burkhart, 1999.8
Clear liquids or soft drinks are not to be encouraged. These may be high in carbohydrate and osmolality and lead to osmotic diarrhoea. Hyponatraemia may occur due to their low sodium content. Chicken broth is inappropriate as it may lead to hypernatraemia8 (Table 10.5.9).
Some trials suggest frozen oral rehydration solution is better tolerated than the liquid form.27 One study suggested that children with a serum bicarbonate greater than 13 mmol L−1, and who were vomiting, could be treated with rapid intravenous rehydration (20–30 mL kg−1 of isotonic crystalloid over 1–2 hours) in the ED.28 They could then tolerate oral rehydration and be discharged home. Shorter stays in the ED were also enabled compared to times reported with oral rehydration solution.
Hypernatraemia and hypernatraemic dehydration
Hypernatraemia is usually caused by water depletion, or water plus sodium depletion in dehydration states where there is relatively more water depletion. Most causes of dehydration (see Table 10.5.5) can cause hypernatraemia. Rarely, hyperaldosteronism or salt poisoning cause sodium excess.
If salt poisoning is suspected, obtain gastric fluid via nasogastric tube for sodium analysis. Features of dehydration are usually absent and FENa is high, often >10%. If there is salt excess without dehydration, then give less than maintenance water with no sodium, aiming for a very slow correction of plasma sodium, <0.6 mmol L−1 hr−1. A diuretic may be considered and, in extreme cases, dialysis.29–31
Sepsis and meningococcal disease
There is no compelling argument for crystalloid or colloid.18–21 A study of septic shock14 found that fluid resuscitation in excess of 40 mL kg−1 in the first hour was associated with improved survival, decreased occurrence of persistent hypovolaemia and no increase in the risk of acute respiratory distress syndrome or pulmonary oedema. No restriction was placed on the type of resuscitation fluid used.
A study of severe meningococcaemia17 looked at 336 patients retrospectively and found a decreased mortality, both in the general population and in high-risk groups, when FFP was not used.
Haemorrhagic shock
FFP should be thawed if a large transfusion is expected, and platelets requested. FFP only comes in adult-sized bags and doses of 10 mL kg−1 are appropriate with repeating of coagulation times.9
Head injury
Vigorous treatment of shock should be undertaken to maintain cerebral perfusion pressure.32
Interest has been generated in the use of 7.5% saline in head-injured patients. A prospective randomised study of 35 patients with head injury compared Ringer’s lactate with 7.5% saline.33 Children treated with hypertonic saline had a statistically significant decrease in complications, intracranial pressure and ICU stay. There was no difference, however, in survival or duration of hospital stay. Neurological outcome was not well documented. More evidence is required.33–35
Burns
Originally, 50% of this was to be given in the first 8 hours and the rest over the remaining 16 hours. However, recent evidence suggests that restoration of urinary output and vital signs occurs earlier if 50% is given in the first 4 hours. Many burns patients require more fluid than is provided by the Parkland formula. Aim for urine output greater than 1 mL kg−1 hr−1.35
Some evidence also suggests that paediatric burns patients tolerate hypoalbuminaemia well and may not require albumin.36
Diabetic ketoacidosis
Rehydration over 48 hours is desirable so as to minimise the risk of cerebral oedema.
The fluid of choice is controversial. One article suggests that normal saline be used until restoration of glucose and then a change should be made to 0.45% saline. Potassium replacement should be started when the urinary output is adequate.37
Hyperkalaemia
Investigations should include a blood gas, creatine kinase (CK) and glucose.
Resonium, 1 g kg−1 every 4–6 hours oral or rectal, may eliminate 1–2 mmol kg−1 of potassium.
All cases of hyperkalaemia should be admitted with ECG monitoring.
Maintenance fluids
A common formula for calculating ‘maintenance’ water requirements in a healthy child is as follows:
Reduce this by one-third initially, or even one-half if there is risk of cerebral oedema, e.g. in meningitis or brain injury, especially if hyponatraemia already exists. Less than ‘maintenance’ water should be given when water retention is likely, such as in sepsis and severe respiratory disease (0.7 × ‘maintenance’), cardiac failure (0.5 × ‘maintenance’), renal failure or other oliguric states without hypovolaemia (0.3 × ‘maintenance’ plus urine output) (Table 10.5.10). Greater than ‘maintenance’ is often advocated when water losses are expected, e.g. spontaneous hyperventilation or fever, but it is probably more appropriate to increase fluids only if urine output falls to 0.5 mL kg−1 hr−1. The most appropriate maintenance IV fluid in the acutely ill child is 0.45% saline because at half-‘maintenance’ rates this provides 1–3 mmol kg−1 day−1 of sodium, but separate consideration should always be given to sodium and other electrolyte requirements. Do not include dehydration deficit in maintenance; consider this separately and use 0.9% saline or a buffered isotonic solution for replacement.
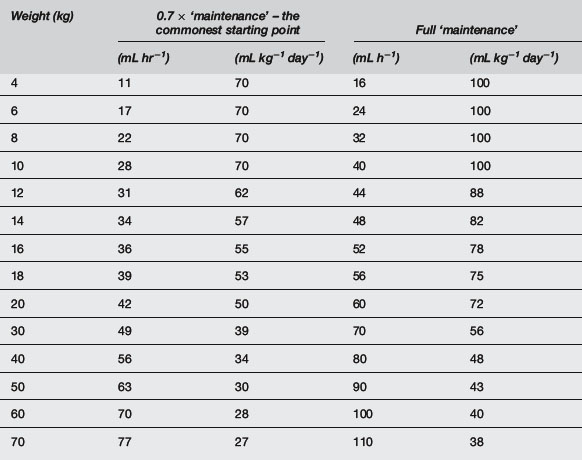
Acid–base disorders
Note that Mg2+ and Ca2+ are divalent and so are 2 × mmol L−1.
Metabolic acidosis
In metabolic acidosis, bicarbonate deficit (mmol kg−1) is given by:
First treat the underlying disease (Table 10.5.11), including general management of renal, hepatic failure, cardiac failure, hypoxia, shock and hypovolaemia. Alkali is not usually needed for mild acidosis (pH > 7.2) because the acidosis itself does not cause any compromise. Even extreme acidaemia (pH < 6.8) can be followed by full recovery, and bicarbonate therapy is only indicated if there is severe hyperkalaemia or tricyclic poisoning. Sudden changes in acid–base status should be avoided. Sodium bicarbonate has many adverse effects, especially when given rapidly, such as hypokalaemia, decreased plasma ionised calcium, sodium load, osmolar load, increased haemoglobin/oxygen affinity, exacerbation of effects of hypophosphataemia, and late metabolic alkalosis. There is no evidence for increased intracellular acidosis. Sodium bicarbonate is especially unhelpful in lactic acidosis, resulting in sodium overload with a metabolic alkalosis as the lactic acid is metabolised to bicarbonate during recovery.
Metabolic alkalosis
1 Henning R. Fluid resuscitation in children. Emerg Med. 1995:57-62. Second Australian Symposium on Fluid Replacement
2 Cullen P. Fluid resuscitation in infants and children. Curr Anaesth Crit Care. 1996;7:197-205.
3 Tobias J.D. Shock in children: The first 60 minutes. Pediatr Ann. 1996;25:330-338.
4 Saavedra J.M., Harris G.D., Li S., Finberg L. Capillary refilling (skin turgor) in the assessment of dehydration. Am J Dis Child. 1991;145:296-298.
5 Gorelick M.H., Shaw K.N., Baker N. Effect of ambient temperature on capillary refill in healthy children. Pediatrics. 1993;92:699-702.
6 Mackenzie A., Barnes G., Shann F. Clinical signs of dehydration in children. Lancet. 1989;ii:605-607.
7 Vega R.M., Avner J.R. A prospective study of the usefulness of clinical and laboratory parameters for predicting percentage of dehydration in children. Pediatr Emerg Care. 1997;13:179-182.
8 Burkhart D.M. Management of acute gastroenteritis in children. Am Fam Phys. 1999;60:2555-2563.
9 Morgan W.M., O’Neill J.A. Hemorrhagic and obstructive shock in pediatric patients. New Horiz. 1998;6:150-154.
10 The SAFE Study Investigators. A comparison of albumin and saline for fluid resuscitation in the Intensive Care Unit. N Engl J Med. 2004;350:2247-2256.
11 Schierhout G., Roberts I. Fluid resuscitation with colloid or crystalloid solutions in critically ill patients: A systematic review of randomised trials. Br Med J. 1998;316:961-964.
12 Emery E.F., Greenhough A., Gamsu H.R. Randomised controlled trial of colloid infusions in hypotensive preterm infants. Arch Dis Child. 1992;67:1185-1188.
13 Schexnayder S.P. Pediatric septic shock. Pediatr Rev. 1999;20:303-307.
14 Carcillo J.A., Davis A.L., Zaritsky A. Role of early fluid resuscitation in pediatric septic shock. JAMA. 1991;266:1242-1245.
15 Goh A.Y.T., Chan P.W.K., Lum L.C.S. Sepsis, severe sepsis and septic shock in paediatric multiple organ dysfunction syndrome. J Paediatr Child Health. 1999;35:488-492.
16 Pollard A.J., Britto J., Nadel S., et al. Emergency management of meningococcal disease. Arch Dis Child. 1999;80:290-296.
17 Busund R., Straume B., Revhaug A. Fatal course in severe meningococcemia: Clinical predictors and effect of transfusion therapy. Crit Care Med. 1993;21:1699-1705.
18 McClelland B. Human albumin administration in critically ill patients. Br Med J. 1998;317:882. [letter]
19 Cochrane Injuries Group Albumin Reviewers. Human albumin administration in critically ill patients: Systematic review of randomised controlled trials. Br Med J. 1998;317:235-240.
20 Nadel S., De Munter C., Britto J., et al. Albumin: Saint or sinner? Arch Dis Child. 1998;79:384-385.
21 Moyer V.A., Elliott E.J. Evidence-based pediatrics: The future is now. J Pediatr. 2000;136:282-284.
22 Mackenzie A., Barnes G. Randomised controlled trial comparing oral and intravenous rehydration therapy in children with diarrhoea. Br Med J. 1991;303:393-396.
23 Gremse D.A. Effectiveness of nasogastric rehydration in hospitalised children with acute diarrhea. J Pediatr Gastroenterol Nutr. 1995;21:145-148.
24 Barnes G.L. Oral rehydration solutions in gastroenteritis before and after admission to hospital. J Paediatr Child Health. 1996;32:16-17.
25 Eliason B.C., Lewan R.B. Gastroenteritis in children: Principles of diagnosis and treatment. Am Fam Physician. 1998;58:1769-1776.
26 Wittenberg D.F., Ramji S. Paediatric diarrhoea – rehydration therapy revisited. S Afr Med J. 1995;85:655-658.
27 Santucci K.A., Anderson A.C., Lewander W.J., Linakis J.G. Frozen oral hydration as an alternative to conventional enteral fluids. Arch Pediatr Adolesc Med. 1998;152:142-146.
28 Reid S.R., Bonadio W.A. Outpatient rapid intravenous rehydration to correct dehydration and resolve vomiting in children with acute gastroenteritis. Ann Emerg Med. 1996;28:318-323.
29 Ng P.C., Chan H.B., Fok T.F., et al. Early onset of hypernatraemic dehydration and fever in exclusively breast fed infants. J Paediatr Child Health. 1999;35:585-587.
30 Moritz M.L., Ayus J.C. The changing pattern of hypernatremia in hospitalised children. Paediatrics. 1999;104:435-439.
31 Dunn K., Butt W. Extreme sodium derangement in a paediatric inpatient population. J Paediatr Child Health. 1997;33:26-30.
32 Scalea T.M., Maltz S., Yelon J., et al. Resuscitation of multiple trauma and head injury: Role of crystalloid fluids and inotropes. Critl Care Med. 1994;22:1610-1615.
33 Simma B., Burger R., Falk M., et al. A prospective, randomised, and controlled study of fluid management in children with severe head injury: Lactated Ringer’s solution versus hypertonic saline. Pediatr Crit Care. 1998;26:1265-1270.
34 Sheikh A.A., Matsuoka T., Wisner D.H. Cerebral effects of resuscitation with hypertonic saline and a new low sodium hypertonic fluid in hemorrhagic shock and head injury. Crit Care Med. 1996;24:1226-1232.
35 Cocks A.J., O’Connell A., Martin H. Crystalloids, colloids and kids: A review of paediatric burns in intensive care. Burns. 1998;24:717-724.
36 Sheridan R.D., Prelack M.S., Cunningham J.J. Physiological hypoalbuminemia is well tolerated by severely burned children. J Trauma: Injury. Infect Crit Care. 1997;43:448-452.
37 Edge J.A. Management of diabetic ketoacidosis in childhood. Br J Hosp Med. 1996;55:508-512.
Arieff A.I. Postoperative hyponatraemic encephalopathy following elective surgery in children. Paediatr Anaesth. 1998;8:1-4. [editorial]
Arieff A.I., Ayus J.C. Treatment of symptomatic hyponatremia: Neither haste nor waste. Crit Care Med. 1991;19:748-751. [editorial]
Arieff A.I., Ayus J.C., Fraser C.L. Hyponatremia and death or permanent brain damage in healthy children. Br Med J. 1992;304:1218-1222.
Bickell W.H., Wall M.J., Pepe P.E., et al. Immediate versus delayed fluid resuscitation for hypotensive patients with penetrating torso injuries. N Engl J Med. 1994;331:1105-1109.
Bohn D. Problems associated with intravenous fluid administration in children: Do we have the right solution? Curr Opin Paediatr. 2000;12:217-221.
Brown W.D., Caruso J.M. Extrapontine myelinolysis with involvement of the hippocampus in three children with severe hypernatraemia. J Child Neurol. 1999;14:428-433.
Chan J.C.M., Gill J.R., editors. Kidney electrolyte disorders. New York: Churchill Livingstone, 1990.
Coulthard M.G., Haycock G.B. Distinguishing between salt poisoning and hypernatraemic dehydration in children. Br Med J. 2003;326:157-160.
Doyle J.A., Davis D.P., Hoyt D.B. The use of hypertonic saline in the treatment of traumatic brain injury. J Trauma. 2001;50:367-383.
Duke T., Molyneux E.M. Intravenous fluids for seriously ill children: Time to reconsider. Lancet. 2003;362:1320-1323.
Feld L.G. Hyponatremia in infants and children: A practical approach. J Nephrol. 1996;9:5-9.
Finberg L., Kravath R.E., Hellerstein S., editors. Water and electrolytes in pediatrics. Physiology, pathology and treatment, 2nd ed., Philadelphia: WB Saunders, 1993.
Fraser C.L., Arieff A.I. Epidemiology, pathophysiology and management of hyponatremic encephalopathy. Am J Med. 1997;102:67-77. [review]
Gerigk M., Gnehm H.P.E., Rascher W. Arginine vasopressin and renin in acutely ill children: Implication for fluid therapy. Acta Paediatr. 1996;85:550-553.
Halberthal M., Halperin M.L., Bohn D. Acute hyponatremia in children admitted to hospital: Retrospective analysis of factors contributing to its development and resolution. Br Med J. 2001;322:780-782.
Holliday M.A., Segar W.E. Reducing errors in fluid therapy management. Pediatrics. 2003;111:424-425. [Commentary]
Ichikawa I., Yoshioka T., editors. Paediatric textbook of fluids and electrolytes. Baltimore: Williams & Wilkins, 1990.
Kamel K.S., Wei C. Controversial issues in the treatment of hyperkalemia. Nephrol Dial Transpl. 2003;18:2215-2218.
Kellum J.A. Saline-induced hyperchloremic metabolic acidosis. Crit Care Med. 2002;30:259-261.
Kelly A., Moshang T. Disorders of water, sodium and potassium homeostasis. In: Nichols D.G., editor. Rogers’ textbook of pediatric intensive care. Baltimore: Lippincott Williams & Wilkins; 2008:1615-1634.
Laureno R., Karp B.I. Myelinolysis after correction of hyponatremia. Ann Intern Med. 1997;126:57-62.
McClure R.J., Prasad V.K., Brocklebank J.T. Treatment of hyperkalemia using intravenous and nebulised salbutamol. Arch Dis Child. 1994;70:126-128.
Meyers A. Fluid and electrolyte therapy for children. Curr Opin Paediatr. 1994;6:303-309.
Myers C.T. Minimal volume, hypotense resuscitation. Emerg Med. 1995:51-56. Second Australian Symposium on Fluid Replacement
Phin S.J., McCaskill M.E., Browne G.J., Lam L.T. Clinical pathway using rapid rehydration for children with gastroenteritis. J Paediatr Child Health. 2003;39:343-348.
Rodriguez-Soriano J. Potassium homeostasis and its disturbances in children. Paediatr Nephrol. 1995;9:364-374.
Ronco C., Bellomo R., Kellum J., editors. Critical care nephrology. Philadelphia: Saunders Elsevier, 2009.
Tuthill D.P., Hewson M., Wilson R. Paediatric resuscitation – by phone. J Paediatr Child Health. 1998;34:524-527.
Wilkins B. Fluid therapy in acute paediatrics: A physiological approach. Curr Paediatr. 1999;9:51-56.