Chapter 78 Diabetic Ketoacidosis
Box 78–1 DKA Treatment in Children and Adolescents
Modified from Glaser NS: Pediatric diabetic ketoacidosis and hyperglycemic hyperosmolar state. Pediatr Clin North Am 52:1611-1635, 2005.
Fluids
Insulin
Potassium and other electrolyte replacement
Monitoring
Etiology, Definition, and Presentation
In a child with new onset of type 1 diabetes, declining insulin production results from autoimmune destruction of pancreatic beta cells. The concentration of insulin is decreased relative to glucagon causing excess hepatic glucose production and decreased peripheral glucose uptake in muscle and adipose tissue.1, 2 When the serum glucose concentration rises above approximately 180 to 200 mg/dL, the renal threshold for glucose reabsorption is exceeded causing glycosuria, which leads to osmotic diuresis and compensatory polydipsia. Low insulin concentrations also stimulate the release of free fatty acids (FFA) from adipose tissue to fuel ketogenesis.3 This, in combination with activation of the hepatic β-oxidative enzyme sequence resulting from relative excess of glucagon in relation to insulin, results in markedly increased hepatic ketone production.2–4
Progressive dehydration and increasing acidosis eventually stimulate additional release of the counterregulatory (“stress”) hormones, cortisol, catecholamines, and growth hormone, which accelerate hepatic glucose output and ketone production.5,6 Infection or other illness or injury can likewise contribute to this process by stimulating release of counterregulatory hormones. Elevated cortisol concentrations augment FFA release from adipose tissue and decrease peripheral glucose uptake. Increased epinephrine concentrations directly increase glycogenolysis and stimulate release of gluconeogenic precursors from muscle.7,8 Both epinephrine and norepinephrine also stimulate lipolysis and β-oxidation of FFAs.9,10 Catecholamines may also directly inhibit insulin secretion, thereby accelerating DKA in those with endogenous insulin capacity, such as a new diagnosis of type 1 diabetes or those with type 2 diabetes.11,12 Growth hormone also decreases peripheral glucose uptake, and enhances ketone production by increasing FFA release.13 Elevated concentrations of counterregulatory hormones thus result in increased acidosis, hyperglycemia, and dehydration. This in turn stimulates further counterregulatory hormone release thereby creating a “vicious cycle” resulting in rapid worsening of DKA (Figure 78-1).
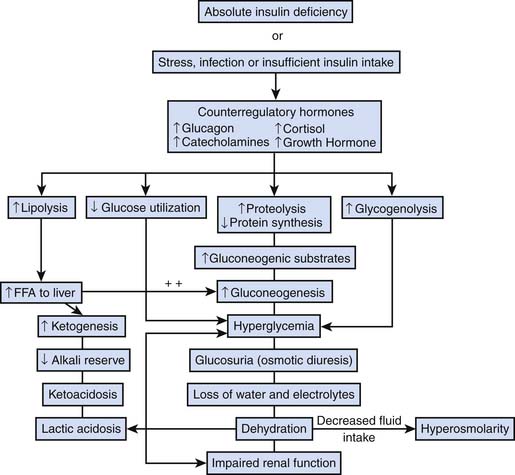
Figure 78–1 Pathophysiology of DKA.
(From Wolfsdorf J, Glaser N, Sperling MA: Diabetic ketoacidosis in infants, children, and adolescents: a consensus statement from the American Diabetes Association, Diabetes Care 29[5]:1150-1159, 2006.)
During DKA, intestinal ileus results from potassium depletion, acidosis, and diminished splanchnic perfusion, causing abdominal pain and vomiting and thereby limiting fluid intake. Progressive dehydration eventually leads to diminished tissue perfusion sufficient to cause accumulation of lactic acid, enhancing acidosis.14 In addition, poor perfusion may result in diminished renal function, limiting the capacity for clearance of glucose and ketones. Ongoing osmotic diuresis and ketonuria in the setting of acidosis also results in urinary losses of electrolytes (potassium, sodium, chloride, calcium, phosphate, and magnesium).
Classical symptoms of DKA include polyuria, polydipsia, polyphagia, weight loss, abdominal pain, nausea, and vomiting. Abdominal tenderness, absence of bowel sounds and guarding are frequent and may even mimic an acute abdomen.15 Tachycardia and signs of hypoperfusion, such as delayed capillary refill time and cool extremities, are also common as well as dry mucous membranes, absence of tears, and poor skin turgor. Despite substantial volume depletion, however, hypotension is unusual in children with DKA and occasional children may present with hypertension. Kussmaul breathing and tachypnea are the result of metabolic acidosis and respiratory compensatory mechanisms. Fruity breath odor (acetone) may be present. Hypothermia has also been described.16
Although hyperglycemia is part of the definition of DKA, in rare cases, the serum glucose concentration may be nearly normal, so called “euglycemic DKA.” This situation has been reported most frequently in pregnant women.17–19 Normal glucose concentrations or even hypoglycemia despite ketosis may also occur in children with known diabetes who administer insulin to treat DKA prior to arrival in the emergency department. In general, however, the persistence and severity of hyperglycemia reflects the severity of dehydration. In the absence of preexisting renal disease or unusually high carbohydrate intake just before presentation, blood glucose concentrations in excess of 500 to 600 mg/dl imply that dehydration is of sufficient severity to diminish the glomerular filtration rate and thereby diminish the capacity for renal clearance of excess glucose.20
Concentrations of ketone bodies (beta-hydroxybutyrate [βOHB] and acetoacetate [AcAc]) are elevated in DKA resulting in acidosis. Hyperchloremic acidosis frequently coexists with increased anion-gap acidosis, and the anion gap reflects the combination of these processes.21 The ratio of βOHB:AcAc (typically 1:1 in the normal state) is increased during DKA and may be as high as 10:1.22 During treatment, this ratio returns to normal. The nitroprusside reaction used to test urine ketone concentrations detects only AcAc and not βOHB. As a result, nitroprusside urine testing cannot be relied on to determine DKA severity or treatment response. Bedside blood ketone meters provide a rapid means for measuring βOHB, and may be useful in place of or in addition to urine testing particularly in patients with anuria or oligoria who produce insufficient amounts of urine for ketone testing.23 Blood ketone measurements are also useful for determining the timing of transition from intravenous to subcutaneous insulin administration. Urine ketones may be present even when blood ketones have normalized as a result of urine stagnating in the bladder.
Hyperglycemia results in fluid shifts from the extravascular to the intravascular space and a decrease in the serum sodium concentration. This decrease can be calculated as an approximately 1.6 mEq/L decrease in sodium concentration for every 100 mg/dl increase in serum glucose higher than 100 mg/dl or Na corrected = Naactual + (glucose in mmol/L – 5.5 mmol/L)}.24,25 Hyperlipidemia may also contribute to a decrease in measured serum sodium concentrations.26 Typically, serum potassium at presentation is in the high-normal range as a result of redistribution of potassium ions from the intracellular to the extracellular space. Several processes are responsible for intracellular potassium depletion including direct effects of low insulin concentrations, intracellular protein and phosphate depletion, and buffering of hydrogen ions in the intracellular compartment.27 Intracellular potassium stores may be profoundly depleted and the serum potassium concentration typically declines rapidly with insulin treatment. Serum phosphate concentrations similarly decrease during treatment.
Leukocytosis is frequent in children with DKA, likely resulting from elevated concentrations of catecholamines and pro-inflammatory cytokines. In children, new onset of type 1 diabetes or insulin omission are far more common causes of DKA than infection. 28 Therefore, an elevated or left-shifted white blood cell count need not prompt a search for an infectious process in the absence of fever or other symptoms or signs of infection. However, in the presence of fever, careful history, physical examination, and laboratory evaluation to assess for infection is prudent.
Epidemiology
Frequency of Diabetic Ketoacidosis at Diagnosis
The frequency of DKA at diagnosis varies widely by geographic region, with an overall estimated frequency of approximately 20% to 67%. In the population-based US study, SEARCH for Diabetes in Youth, data were collected from self-reported health questionnaires and medical record review. In this study, 25.5% of children and adolescents presented at onset of diabetes in DKA.29 A similar frequency was observed in Germany, with 26.3% of children under the age of 15 presenting in DKA at diagnosis of diabetes. In a collaborative study from Germany and Austria, the Diabetes Patienten Verlausdokumentation (an electronic documentation system for children with diabetes), the frequency of DKA at presentation was 21.1%.30 Younger age (<5 years) and female sex were associated with higher likelihood of presenting in DKA.31,32 A delay in diagnosis is associated with a higher likelihood of DKA, and two factors contributing to this are patient age and provider experience. Regions with a higher prevalence of type 1 diabetes generally have a lower frequency of DKA,33 attributed to heightened awareness in providers and thus earlier detection. The non-specific nature of individual symptoms, such as polyuria, tachypnea, and altered mental status, may cause such symptoms to be misconstrued as urinary tract infection, pneumonia, or meningitis, respectively.34 Mallare et al.35 reported a frequency of DKA of 33% in children and adolescents at the initial visit, and almost double (59%) in those in whom the diagnosis of diabetes was missed at the initial visit. The diagnosis of diabetes was more likely to be missed in very young children (34% of children ≤5 years of age compared to 8.5% in those greater than 10 years of age),35 particularly when these very young children are evaluated by family practitioners rather than pediatricians.36
Frequency of Diabetic Ketoacidosis in Children and Adolescents After Diagnosis
Although there are several population-based studies reporting the frequency of DKA at presentation, fewer data are available describing the incidence of DKA in children and adolescents with established diabetes. Reported frequencies range from 1 to 10 per 100 patient years.37 In the Diabetes Control and Complications Trial, the incidence of DKA in adolescents treated with intensive management regimens was 2.8 per 100 patient-years, significantly lower than the incidence in those treated conventionally (4.7 per 100 patient years). Although this is an older study, it was a time and resource-intensive study, and represents a more idealized situation than often encountered in the overall pediatric diabetes population.38 In a more recent study from the Barbara Davis Center for Childhood Diabetes in Denver, Colorado, the overall incidence of DKA was 8 per 100 person-years. In that study, factors associated with higher incidence included older age, higher HbA1C (relative risk [RR] of 1.68 per 1% increase in HbA1C in younger children, RR of 1.43 in older children), higher reported insulin dose, DSM4 psychiatric diagnoses, and “underinsurance” reflecting lower socioeconomic status.39 DKA is also observed more often in children and adolescents on continuous subcutaneous insulin infusion therapy (CSII) than on subcutaneous injections, particularly in the first year of initiation of CSII.40 However, lower rates of DKA are achievable for those on CSII with adequate training and resources.
Over the past two decades T2DM has been occurring with increasing frequency in older children and adolescents. Certain racial/ethnic groups in the United States are disproportionately affected including Native Americans, Hispanics, and African Americans. DKA can be the clinical presentation for T2DM in youth estimated at 5% to 10%.41 Youth with T2DM may also present with hyperglycemic hyperosmolar state (HHS), also referred to hyperosmotic hyperglycemic non-ketotic coma (HHNK, described more fully in the following section).
Morbidity and Mortality Associated with Diabetic Ketoacidosis
Mortality in children presenting with DKA is approximately 0.25% to 0.30%.37 Most of the mortality in DKA occurs in children with cerebral edema, accounting for 57% to 87% of deaths. Neurologic sequelae of DKA are described in the section on DKA-associated complications. Other causes of morbidity and mortality include sepsis and secondary infection, electrolyte abnormalities (e.g., hypokalemia), arrhythmias, rhabdomyolysis, thrombosis, pneumomediastinum, subcutaneous emphysema, and pulmonary edema.
Management Guidelines
Fluids
Restoration of adequate peripheral perfusion and hemodynamic stability with bolus administration of intravenous fluids (0.9% saline or other isotonic fluids) should begin as soon as possible. Typical patients require an initial fluid bolus of 10 ml/kg that may be repeated if ongoing hemodynamic instability is present. Studies have shown that clinical assessments of dehydration severity in children with DKA tend to be inaccurate (Figure 78-2).42 The average degree of dehydration for most patients is approximately 7% to 9% of body weight and this figure should be used as a basis for determining the total volume of fluids to be replaced.42,43