Development of the brain
Neural tube formation
The central nervous system is derived from the neural tube, which appears during the fourth week after fertilization. At this early stage the embryo takes the form of a trilaminar germ disc, lying in the floor of the amniotic sac (Fig. 2.1). The germ disc is composed of three layers of tissue from which all the structures of the body originate:
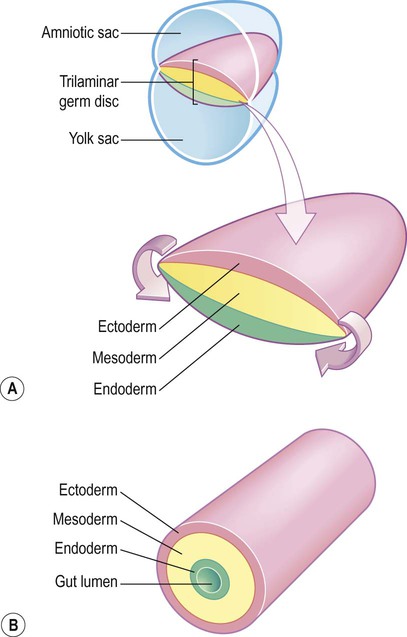
(A) The germ disc is composed of three primitive germ layers from which all body tissues are derived. A complex process of folding (not illustrated) transforms the germ disc so that the ectoderm comes to lie on the outside and the endoderm lines the gut lumen; (B) The body can be represented in cartoon form as a hollow cylinder with the mouth at one end and the anus at the other.
The ectoderm (Greek: ektos, outside) contributes mainly to the skin, but also gives rise to the central and peripheral nervous systems.
The mesoderm (Greek: misos, middle) is the origin of the cardiovascular, musculoskeletal, urinary and reproductive systems.
The endoderm (Greek: endon, within) contributes chiefly to the respiratory and gastrointestinal tracts, including the liver, gallbladder and pancreas.
The process by which the embryonic ectoderm gives rise to the neural tube is called primary neurulation (Fig. 2.2). It is initiated by the notochord, a rod-like mesodermal structure that helps to define the longitudinal axis of the embryo. The notochord releases soluble mediators including cell adhesion molecules and trophic factors, which influence the overlying ectoderm. This process is termed neural induction.
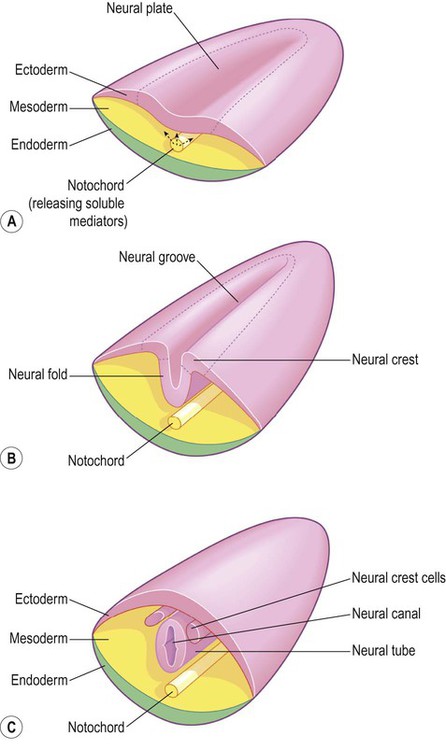
(A) The mesodermal notochord releases soluble mediators that induce neural tube formation in the overlying ectoderm (neural induction); (B) The neural tube forms from the paired neural folds which flank the neural groove; (C) The neural crest cells detach from the dorsolateral margins of the neural tube and will give rise to much of the peripheral nervous system, including the spinal and autonomic ganglia.
Ultrasound studies show that in humans the neural tube begins to form at around 21-23 days after fertilization, when the embryo is just 2–3 mm in length. The first change (which occurs at about day 18) is the appearance of the neural plate, a broad area of thickening in the dorsal ectoderm. A shallow longitudinal depression termed the neural groove separates the neural plate into paired neural folds which gradually roll up to form a cylinder. The neural folds ultimately meet in the midline and unite to create the neural tube and neural canal. Fusion begins in the presumptive cervical region and proceeds both rostrally and caudally in a ‘zipper-like’ fashion. The open ends of the neural tube are called the cranial and caudal neuropores, which have normally closed by the beginning of week five. Disorders resulting from faulty neural tube closure are discussed in Clinical Box 2.1.
Origin of neurons and glial cells
The wall of the neural tube can be divided into three concentric zones (Fig. 2.3). The ventricular zone is closest to the fluid-filled neural canal (which will become the cerebral ventricles) and is composed of proliferating neural progenitor cells. These include neuroblasts (neuronal precursors) and glioblasts (glial precursors) that give rise to most of the specialized cells of the central nervous system. Microglia are the resident phagocytes of the brain, but originate from the bone marrow and are of mesodermal rather than ectodermal lineage.
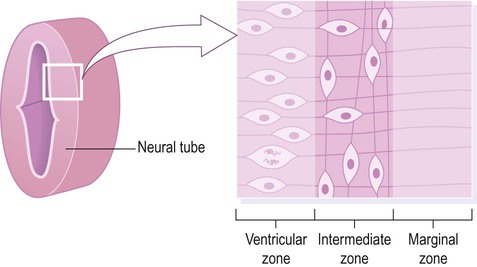
Cell division (mitosis) occurs in the ventricular zone, giving rise to neurons and glia.
Formation of the cerebral cortex
In the cerebral hemispheres the majority of neurons ultimately vacate the intermediate zone or undergo programmed cell death (see Ch. 8) and this region eventually becomes the subcortical white matter. Up to 50% of neurons produced in the developing brain fail to (i) reach their intended targets or (ii) make appropriate functional connections and are consequently deleted by programmed cell death.
Sensory and motor areas
The neural tube has two functional divisions, separated by the sulcus limitans (Fig. 2.4A). The basal plate occupies the ventral portion of the neural tube (anterior to the sulcus limitans) and is predominantly a motor structure; the alar plate is located dorsally and is sensory. This dorsal–ventral division between sensory and motor areas is reflected in the adult spinal cord, with sensory fibres entering via the dorsal roots and motor fibres emerging in the ventral roots (Fig. 2.4B). It is echoed throughout the central nervous system so that motor structures (e.g. cortical areas, tracts, nuclei) tend to be anterior to sensory structures.
The neural crest
The peripheral nervous system is mainly derived from the neural crest. This is a population of cells that detaches from the lateral margins of the neural plate during neurulation (see Fig. 2.2). Neural crest cells that come to lie dorsolateral to the neural tube ultimately become the primary sensory neurons of the dorsal root ganglia. These neurons are initially bipolar but their central and peripheral processes fuse at a common T-shaped extension of the cell body to form a single continuous axon. For this reason they are described as pseudounipolar (Greek: pseudo-, false). The central processes of the dorsal root ganglion cells innervate the alar (sensory) plate of the neural tube, whereas the peripheral processes enter the spinal nerves at each segmental level (see Fig. 2.4B).