Chapter 29 Craniovertebral Junction
A Reappraisal
• Atlantoaxial joint opening, manipulation, and direction fixation form important components of treatment of craniovertebral instability.
• Lateral masses of C1 and C2 are firm and largely cortical in nature and provide a solid ground for screw fixation.
• For atlantoaxial dislocation, atlantoaxial fixation should be done.
• Reduction of basilar invagination can be performed by distraction of the facets of the atlas and axis in a specific group of patients.
• Syringomyelia is always a secondary phenomenon. The primary cause should be identified and appropriately treated. Direct manipulation of the syrinx can be harmful.
Atlantoaxial Dislocation
Mobile and Reducible Atlantoaxial Dislocation
In the year 2001, we analyzed 160 cases with mobile and reducible AAD treated in our department during the years 1988 to 2001.1,2 In the series, the etiological factor for the dislocation included Morquio’s disease (9 cases), Down syndrome (8 cases), and craniosynostosis (5 cases). Although trauma leads to mechanical disruption of ligaments of the region, in “congenital” dislocation and dislocation related to known syndromes, laxity or incompetence of the ligaments is more often the cause. In several cases, the exact differentiation between a congenital and an acquired cause is difficult to decipher. The range of mobility of the dislocation is in general significantly higher in cases with congenital dislocation than in cases with trauma-related dislocation.
Surgical Treatment
The aim of surgery in general is to restore normal or the best possible alignment and to achieve stability of the atlantoaxial joint. The techniques of fixation for atlantoaxial dislocation can be divided into midline procedures that involve fixation of the arch of the atlas with the lamina of the axis and lateral mass fixation procedures. Midline methods of fixation include use of interlaminar clamps, Gallie’s posterior C1-C2 sublaminar wiring,3 Brooks-Jenkins fusion,4 and the Sonntag technique of sublaminar wiring.5 Lateral mass fixation procedures include Goel’s C1 lateral mass and C2 pedicle/pars screw fixation (interarticular)1,2 and the Magerl’s C1-C2 transarticular technique.6
Metal implants and fixation methods provide an initial period of fixation of the region, facilitating bony fusion. Bony fusion takes about 3 months and the metal implants should be strong enough to hold the region for that period. It ultimately depends on bony fusion to provide stability to the region. Bone harvested from the patient’s own iliac crest has been identified by several authors to be superior to any other form of bone graft or artificial material. Compromise on using the type of bone graft can lead to inadequate fusion, a phenomenon that can mar the entire operative procedure. Multiple pieces of corticocancellous bone graft should be placed in the craniovertebral region after elaborately preparing the host bone. The movements in the atlantoaxial region occur in the atlantoaxial joint. Any method of stabilization that directly fixes the joint and is segmental in nature has superiority over methods that employ fixation remote to the site of maximum movements and those that attempt to fix multiple bone segments. The lateral mass fixation techniques7 have been identified to be philosophically superior and biomechanically stronger to the techniques that involve fixation of the midline structures such as lamina and arch of atlas.
Craniovertebral Junction and Related Anatomy
The vertebral artery adopts a serpentine course in relation to the craniovertebral region. The artery has multiple loops and an intimate relationship with the atlas and axis bones. We observed a wide variability of the course of the artery in our specimens. The shape, size, and location of the vertebral artery groove on the inferior aspect of the superior articular facet of the C2 and over the posterior arch of the atlas have wide variations. The vertebral artery during its entire course is covered with a large plexus of veins. The venous plexuses are the largest in the region lateral to the C1-C2 joint. After a relatively linear ascent of the vertebral artery in the foramen transversarium of C6 to C3, the artery makes a loop medially toward an anteriorly placed superior articular facet of the C2 vertebra, making a deep groove on its inferior surface. The extent of medial extension of the loop varies. The distance of the artery from the midline of the vertebral body of C2 as would be observed during a transoral surgical procedure is an average of 12 mm.8 The vertebral artery loops away from the midline underneath the superior articular facet of the C2.
The dens or the odontoid process is flanked by two large, superior facets, extending laterally onto the adjoining pars interarticularis and articulating with the inferior atlantal facets. The superior facet of the C2 vertebra differs from the facets of all other vertebrae in two important characteristics. First, the superior facet of C2 is present in proximity to the body when compared to other facets, which are located in proximity to the lamina. Second, the vertebral artery foramen is present partially or completely in the inferior aspect of the superior facet of C2, but in other cervical vertebrae, the vertebral artery foramen is located entirely in relation to the transverse process. Unlike superior facets of all other vertebrae, they do not form a pillar with the inferior facets, being considerably anterior to them. The pedicle of the C2 vertebra is relatively small. The course of the vertebral artery in relation to the inferior aspect of the superior articular facet of the C2 makes it susceptible to injury during transarticular and interarticular screw implantation techniques. The average distance of the artery from the ganglion is 7.5 mm. This finding suggests that the dissection around the lateral end of the ganglion should be carefully done.
Lateral Mass Plate (or Rod) and Screw (Monoaxial or Polyaxial) Fixation (Figs. 29.1 to 29.6)1,2
Cervical traction is set up prior to induction of anesthesia and the weights are progressively increased to approximately 5 to 8 kg or one sixth of the total body weight. The patient is placed prone with the head end of the table elevated to about 35 degrees (see Fig. 29.2). Cervical traction stabilizes the head in an optimally reduced extension position and prevents any rotation. The traction also ensures that the weight of the head is directed superiorly toward the direction of the traction and pressure over the face or eyeball by the headrest is avoided. The head is in a “hanging” position, the headrest being placed only for additional or minimal support. Elevation of the head end of the table, which acts as a countertraction, helps in reducing venous engorgement in the operative field. The suboccipital region and the upper cervical spine are exposed through an approximately 8-cm longitudinal midline skin incision centered on the spinous process of the axis. The spinous process of the axis is identified, and the attachment of paraspinal muscles to it is sharply sectioned. The large second cervical ganglion is closely related to the vertebral artery on its lateral aspect (see Fig. 29.3). It is first exposed widely and then sectioned sharply. This procedure provides a wide exposure of the lateral masses of the atlas and axis. Bleeding from the large venous sinuses in the region and in the extradural space can be troublesome. Packing of the region with Surgicel and Gelfoam can assist in the control of venous bleeding. The joint capsule is cut sharply, and the articular surfaces of the joint are exposed. The adjacent synovial articular surfaces of the atlantoaxial joint are decorticated widely with a microdrill, and pieces of bone harvested from the iliac crest are stuffed into the joint space. The lateral aspect of the lamina and a part of the pars of the axis are drilled to make the posterior surface of the lateral mass of the axis relatively flat so that the metal plate can be placed snugly and parallel to the bone. Drilling also helps in reducing the length of the plate and in placing the screw more superiorly and almost directly into the lateral mass of the axis. Actual vertebral artery exposure is unnecessary either lateral to the pars of the axis or superior to the arch of the atlas.
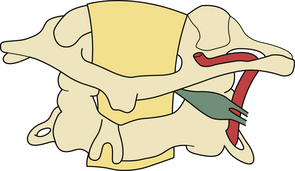
FIGURE 29.3 Drawing showing the relationship of the C2 ganglion, vertebral artery, and atlantoaxial joint.
Screws are implanted into the previously created guide holes in the lateral mass of the atlas and axis through a two- holed (approximately 2 cm in length) metal (stainless steel or titanium) plate. First, a screw is placed into the atlas. It is directed at an angle of approximately 15 degrees medial to the sagittal plane and 15 degrees superior to the axial plane. The preferred site of screw insertion is at the center of the posterior surface of the lateral mass, 1 to 2 mm above the articular surface. Whenever necessary, careful drilling of the inferior surface of the lateral aspect of the posterior arch of the atlas in relation to its lateral mass can provide additional space for the placement of the plate and screw implantation. The screw may even be implanted by choosing an insertion point on the articular surface of the lateral mass of the atlas. Such a site is useful more frequently in children than in adult patients. Screws can also be implanted into the facet of the atlas through the lateral aspect of the posterior arch of the atlas. Owing to the intimacy of vertebral artery relationships, screw implantation in the axis is relatively unsafe and needs to be precise. It was observed that the screw implantation in the superior facet of the C2 vertebra has to be sharply medial and directed toward the anterior tubercle of the C1 for transarticular fixation and toward the vertebral body of C2 for interarticular fixation. The pars interarticularis can be divided into nine quadrants;9 the superior and medial compartments can be used for the interarticular technique of screw implantation. Neuronavigation can be of assistance in identifying the best and the safest trajectory of the screw. The direction of screw implantation must be sharply medial and superior and should be toward the superior aspect of the body of the axis vertebra toward the midline. The medial surface of the pedicle of the axis is identified before the implantation of the screw. The screw is directed at an angle approximately 25 degrees medial to the sagittal plane and 15 degrees superior to the axial plane. The angle of screw insertion varies, depending on the local anatomy and the size of the bones. The quality of cancellous bone in the lateral masses of the atlas and axis in the proposed trajectory of screw implantation is generally good, providing an excellent purchase of the screw, and avoids the vertebral artery. The screws used are 2.9 mm in diameter in adult patients and 2.7 mm in diameter in pediatric patients. The length of the required screw is calculated on the basis of the size of the lateral masses observed on the preoperative radiological studies. The approximate lengths of the atlas screws are 26 to 28 mm in adults and 22 to 26 mm in children. The screws in the atlas and axis are almost similar in their length. The lateral masses of the atlas and axis are firm and cortical in nature, and, although preferable, it is not mandatory that the screws engage both the posterior and anterior cortices. If the screw traverses beyond the anterior cortex, it will lie harmlessly in the anteriorly displaced soft tissue. Injury to the critical neurovascular and pharyngeal wall is possible in such a situation but is extremely rare. Intraoperative fluoroscopic control and navigation were found to be helpful but not essential in determining the state of the screws. Large pieces of corticocancellous bone graft from the iliac bone are then placed over the adequately prepared posterior elements of atlas and axis. After the wound is closed, cervical traction is discontinued. The patients are mobilized as soon as possible and advised to wear a hard cervical collar for 3 months.
Double Insurance Fixation10
An alternative method of atlantoaxial fixation that combines the transarticular method of fixation and the interarticular fixation technique can be used. The technique combines the biomechanical strengths of both of the more commonly used techniques of fixation and provides maximal stability to the implants (see Figs. 29.4 and 29.5).
Basilar Invagination
Basilar invagination forms a prominent component of the craniovertebral anomalies. Chiari malformation and syringomyelia are commonly associated with basilar invagination and are the soft tissue components of the dysgenesis. Plain radiological and tomographic parameters have been principally used to diagnose basilar invagination for many years. There has been a renewed interest in the normal anatomy and pathological lesions of the craniovertebral junction with the development of imaging by high-resolution CT scan and MRI. Dynamic MRI and CT scans have helped in the evaluation of the pathology of basilar invagination, in the assessment of biomechanics of the joints, and in the formulation of a rationale for the surgical strategy. Despite the clarity of imaging, controversy regarding the management of basilar invagination continues. Even the natural history has not been clearly elucidated in the literature. The surgical indications for a given approach together with the timing of the surgical stages are still under discussion.
Pathogenesis
Several theories have been suggested to elucidate the probable cause and origin of basilar invagination. Most of these theories point toward embryological dysgenesis, genetic abnormalities, and viral infections.13–16 Several authors, for over a century, have thought that deformation had a mechanical cause and therefore applied the name: “impressio baseos cranii” (Berg and Retzius, 1855, cited by Virchow, 1876),17 or basilar impression.18 Grawitz (1880)19 believed that basilar invagination was often a result of under- or maldevelopment of the craniovertebral transition region. A range of malformations is frequently associated with anomalies of the atlas and axis, some of which may be quite atypical, and with fissures or defects and bone projections from the spinal column in the craniovertebral transition zone. The latter anomalies were grouped together by von Torklus and Gehle (1970) as suboccipital dysplasias.18 Basilar invagination can be secondary to abnormally inclined alignment of the facets of the atlas and axis.20 The progressive slippage of the atlas over the axis secondary to this malalignment, a process similar to spondylolisthesis in the lumbosacral spine, results in invagination of the odontoid process into the craniocervical cord.20,21 Short neck, low hairline, web-shaped neck muscles, torticollis, reduction in the range of neck movements, and several such physical variations have been described as hallmarks of basilar invagination for over a century. A number of bone fusion deformities and platybasia have also been recorded. Neck pain, muscle spasms, and restriction of neck movements are frequently noted and suggest instability of the region. Degeneration of the atlantoaxial joint and primary or secondary destruction of the facets of atlas or axis can lead to superior and posterior migration of the odontoid process and result in basilar invagination and fixed or irreducible atlantoaxial dislocation. Such involvement of the lateral masses can result in laxity of the posterior atlantoaxial or retro-odontoid ligaments that result in retro-odontoid pannus or “tumor-like” or “osteophyte-like” formation.
Classification into Groups I and II
In 1997, we presented a classification system for basilar invagination that divided it into two discrete categories. This classification helped in clarifying the understanding of the pathology and pathogenesis of the anomaly, in the selection of the surgical treatment, and in prediction of the outcome.22 Based on a single criterion of the absence or presence of Chiari malformation, the anomaly was classified into groups I and II, respectively.
Essentially, group I included cases in which there was invagination of the odontoid process into the foramen magnum and indented into the brainstem. The tip of the odontoid process distanced itself from the anterior arch of the atlas or the inferior aspect of the clivus. The distancing of the odontoid process from the anterior arch suggested the presence of instability in the region and atlantoaxial dislocation. The angle of the clivus and the posterior cranial fossa volume were essentially unaffected in these cases. In group II, on the other hand, the assembly of the odontoid process, anterior arch of the atlas, and the clivus migrated superiorly in unison, resulting in reduction of the posterior cranial fossa volume, which was the primary pathology in these cases. The Chiari malformation or herniation of the cerebellar tonsil was considered to be a result of reduction in the posterior cranial fossa volume. In 1997, Goel first defined the clinical implication of association of small posterior cranial fossa volume and Chiari malformation.22
Classification into Groups A and B
In our 2004 study, we identified a subgroup of patients in whom there was clear radiological evidence of instability of the region that was manifested by distancing of the odontoid process away from the anterior arch of the atlas, and the radiological features matching those of group I cases. Considering this current evaluation we have proposed a new classification for basilar invagination into two groups based on parameters that determined an alternative treatment strategy.23,24 In group A basilar invagination there was a “fixed” atlantoaxial dislocation and the tip of the odontoid process “invaginated” into the foramen magnum and was above the Chamberlain line,25 McRae line of the foramen magnum,26 and Wackenheim’s clival line.27 The definition of basilar invagination of prolapse of the cervical spine into the base of the skull, as suggested by von Torklus,18 was suitable for this group of patients (Fig. 29.7