CHAPTER 32 Connective Tissues
Animals use different proportions of matrix macromolecules to construct connective tissues with a range of mechanical properties to support their organs. Bone is a stiff, hard solid; blood vessel walls are flexible and elastic; and the vitreous body of the eye is a watery gel. Plant cell walls are conceptually similar to the animal extracellular matrix but are composed of completely different molecules. This chapter begins with a discussion of simple connective tissues but concentrates on cartilage, bone, development of the skeleton, and the mechanisms that repair wounds, finishing with a discussion of the plant cell wall.
Loose Connective Tissue
Loose connective tissue consists of a sparse extracellular matrix of hyaluronan and proteoglycans supported by a few collagen fibrils and elastic fibrils. In addition to fibroblasts, the cell population is heterogeneous, including both indigenous and emigrant connective tissue cells (see Fig. 28-3). The loose connective tissue underlying the epithelium in the gastrointestinal tract is a good example of this heterogeneity (Fig. 32-1A), with lymphocytes, plasma cells, macrophages, eosinophils, neutrophils, and mast cells, as well as fibroblasts and occasional fat cells (see Chapter 28 for details on these cells). This variety of defensive cells is appropriate for a location near the lumen of the intestine, which contains microorganisms and potentially toxic materials from the outside world. Loose connective tissue is also found in and around other organs. The optically transparent vitreous body of the eye is an extremely simple loose connective tissue in which fibroblasts produce a highly hydrated gel of hyaluronan and proteoglycans, supported by a loose network of type II collagen. Few defensive cells are required, as the interior of the eye is sterile.
Dense Connective Tissue
Collagen fibers, with or without elastic fibers, predominate over cells in dense connective tissue (Fig. 32-1B). Fibroblasts are present to manufacture extracellular matrix but are relatively sparse. Other connective tissue cells are even rarer, as these tissues are not usually exposed to microorganisms. Collagen fibers can be arranged precisely, as in tendons or cornea (see Fig. 29-3), or less so, as in the wall of the intestine or the skin. Tendons consist nearly exclusively of type I collagen fibers, all aligned along the length of the tendon to provide the tensile strength that is required to transmit forces from muscle to bone. The cornea that forms the transparent front surface of the eye is also well organized into orthogonal layers of collagen fibrils.
Dense connective tissues can also be elastic. For example, the walls of arteries (see Fig. 29-8) and the dermal layer of skin consist of both collagen and elastic fibers. Energy from each heartbeat stretches the elastic fibers in the walls of arteries. Recoil of these elastic fibers propels blood between heartbeats.
Cartilage
Cartilage (Fig. 32-2) is tough, resilient connective tissue that is well suited for a variety of mechanical roles. It covers the articular surfaces of joints and supports large airways, such as the trachea, and skeletal appendages, such as the nose and ears. Cartilage also forms the entire skeleton of sharks and the embryonic precursors of many bones in higher vertebrates. The mechanical properties of cartilage are attributable to abundant extracellular matrix consisting of fine collagen fibrils and high concentrations of glycosaminoglycans and proteoglycans (Fig. 32-3).
Chondrocytes synthesize and secrete macromolecules for the cartilage matrix, which eventually surrounds them completely. Chondrocytes replenish the matrix as the macromolecules turn over slowly, but their ability to remodel and repair the matrix is limited. No blood vessels penetrate cartilage, owing to production of several inhibitors of endothelial cell growth by chondrocytes. Thus, all nutrients must diffuse into cartilage from the nearest blood vessel in the perichondrium, a dense capsule of fibrous connective tissue that covers the surface of cartilage. This capsule contains mesenchymal stem cells (see Box 41-1) that are capable of differentiating into chondrocytes.
Glycosaminoglycans, including hyaluronan, constitute the second major class of matrix macromolecules. Molecules of the proteoglycan aggrecan attach to a hyaluronan backbone like the bristles of a test tube brush, forming so-called megacomplexes (see Fig. 29-14). Aggrecan also binds type II collagen. Highly charged glycosaminoglycans fill the extracellular space and attract water, the most abundant component of the matrix.
A hydrostatic mechanism allows cartilage to resist deformation (Fig. 32-3). Collagen fibrils provide tensile strength (i.e., resistance to stretching) but do not resist compression or bending. Glycosaminoglycans strongly attract water, resulting in an internal swelling pressure that pushes outward against collagen fibrils aligned parallel to the surface of the cartilage. The force of internal hydrostatic swelling pressure is balanced by the force produced by tension on the collagen fibrils. Remarkably, this internally stressed material can resist strong external forces such as those on the articular surfaces of joints. A macroscopic analog is a thin-walled plastic bottle filled with water. One can stand on the bottle provided that it is sealed, whereas neither the empty bottle nor the water could separately support any weight.
Differentiation and Growth of Cartilage
Many growth factors cooperate to influence the differentiation of precursor cells into chondrocytes, the proliferation of chondrocytes, and the production of cartilage matrix molecules. These include Indian hedgehog (Ihh), members of transforming growth factor-b family (TGF-b and bone morphogenetic factors), fibroblast growth factors (FGFs), parathyroid hormone–related protein (PTHrP), and insulin-like growth factors (IGF-I and IGF-II). Chondrocytes produce some of these growth factors (TGF-b, FGFs, and IGFs). During development, adjacent tissues can induce cartilage formation by secreting TGF-b and FGF. SOX9 is the key transcription factor mediating expression of cartilage-specific genes.
Diseases Involving Cartilage
Cartilage fails in common human diseases, including arthritis and ruptured intervertebral disks. Mutations in the genes for cartilage proteins and growth factors cause human disease (Appendix 32-1). Chondrocytes fail to proliferate in the absence of PTHrP or certain receptors for FGF, causing severe deformities of the skeleton. More than 25 different mutations of the human gene for type II collagen cause disorders of cartilage, ranging in severity from death in utero to dwarfism or osteoarthritis. Mutations in genes for minor cartilage collagens cause a variety of symptoms, including degenerative joint disease. A premature stop codon in chicken aggrecan causes lethal skeletal malformations.
Bone
For most vertebrates, bones provide mechanical support and serve as a storage site for calcium. The great strength and light weight of bones are attributable both to the mechanical properties of the extracellular matrix and to efficient overall design, including tubular form and lamination (Fig. 32-4). A superficial layer of compact bone surrounds a medullary cavity that is filled with marrow, fat, or both and is supported by struts of bone arranged precisely along lines of mechanical stress. External surfaces of bones are covered either by dense connective tissue, called periosteum, or by cartilage at joint surfaces. A monolayer of bone-forming cells called osteoblasts line the internal surfaces. Blood vessels supply the medullary cavity and penetrate compact bone through a network of channels. Although bone is durable and strong, continuous remodeling makes bone much more dynamic than it appears.
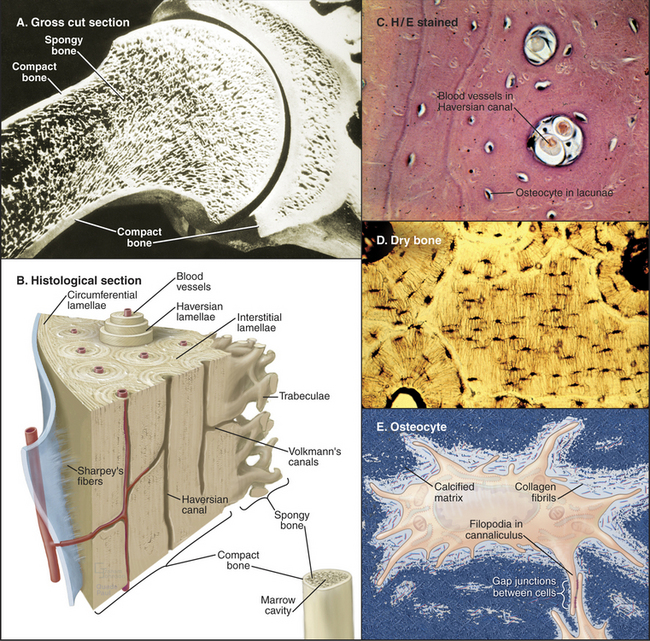
Figure 32-4 organization of long bones. A, Longitudinal section of a shoulder joint of a dried bone specimen. Struts of trabecular spongy bone reinforce compact bone in the cortex.B, A wedge of long bone. Circumferential lamellae form the outer layer just beneath the periosteum (blue) covering the surface. Osteons (Haversian systems) consist of concentric lamellae of calcified matrix and osteocytes arranged around a channel containing one or two capillaries or venules. Interstitial lamellae are fragments of osteons that remain after remodeling (Fig. 32-10). Radial vascular channels connect longitudinal vascular channels to the medullary cavity or periosteum. C, Light micrograph of a cross section stained with hematoxylin-eosin showing circumferential lamellae on the left and two Haversian canals. D, Light micrograph of a cross section of dried bone showing a central interstitial lamella surrounded by three osteons. Narrow canaliculi connect the lacunae housing osteocytes. E, An osteocyte surrounded by calcified matrix and extending filopodia into canaliculi.
(Micrographs courtesy of D. W. Fawcett, Harvard Medical School, Boston, Massachusetts.)
Extracellular Matrix of Bone
Bone is a composite material consisting of collagen fibrils (providing tensile strength) embedded in a matrix of calcium phosphate crystals (providing rigidity) (Fig. 32-4E). Macroscopic analogs of the bone matrix are concrete reinforced by steel rods and fiberglass consisting of a brittle plastic reinforced by glass fibers. Each of these composites is stronger than its separate components. Simple extraction experiments illustrate the contributions of the two components. After removal of calcium phosphate with a calcium chelator, bone is so rubbery that it bends easily. After destruction of collagen by heating, bone is hard but brittle.
Fibrils of type I collagen, the dominant organic component of the matrix (Table 32-1), are arranged in sheets or a meshwork. Covalent cross-links between the collagen molecules in fibrils make them inextensible. The matrix contains more than 100 minor proteins, including growth factors and adhesive glycoproteins, but few proteoglycans.
Name | Content | Functions |
---|---|---|
Bone morphogenic proteins | Minor | TGF-β homologs; cartilage stimulation and bone development and repair |
Collagen type I | 90% | Forms fibrils in the bone matrix |
Osteocalcin | 1%–2% | Network of aspartic acid and γ-carboxylated glutamic acid side chains bind hydroxyapatite; promotes calcification; attracts osteoclasts and osteoblasts |
Osteonectin | 2% | Synthesized in developing and regenerating bone; binds collagen and hydroxyapatite; may nucleate hydroxyapatite crystallization in bone matrix |
Osteopontin | Minor | RGD sequence; binds osteoclast integrins to bone surface |
Proteoglycans | Minor | Decorin, biglycan, osteoadherin; may bind TGF-β |
Sialoproteins | 2% | RGD sequence; binds osteoclast integrins to bone surface |
Bone Cells
Bone is an active tissue that is maintained by a balance of cellular activities. Osteoblasts and osteocytes produce extracellular matrix and establish conditions for its calcification. Osteoclasts resorb bone, as is required for growth and remodeling. An imbalance of these opposing cellular activities causes human diseases. Osteoblasts arise from the same mesenchymal stem cells that give rise to fibroblasts and chondrocytes (see Fig. 28-3). Osteoclasts form by fusion of blood monocytes.
A monolayer of osteoblasts on the surface of growing bone tissue uses a well-developed secretory pathway to synthesize and secrete organic components of the matrix (Fig. 32-5). Unmineralized bone matrix consists largely of type I collagen but includes factors that promote crystallization of calcium phosphate on the surface of these fibrils. Osteoblasts also control the differentiation, but not the activity, of osteoclasts (see Fig. 32-6).