Chapter 6 Congenitally Corrected Transposition of the Great Arteries
More than a century elapsed before Karl von Rokitansky1 applied the term corrected to a hitherto undescribed form of transposition of the great arteries: “The left atrioventricular valve and the left-sided ventricle resembled the usual right atrioventricular valve and right ventricle. The aorta is positioned somewhat left and anterior…. The right-sided ventricle … is finely trabeculated, as usually seen in the left-sided ventricle. The venous atrioventricular ostium has a bivalve. From the right-sided ventricle arises a somewhat right and posteriorly positioned pulmonary artery…. The atria are normal, a right caval atrium and a left pulmonary venous atrium.”
At the end of the 18th century, Mathew Baille2 described a singular malformation characterized by discordant origins of the arterial trunks from the ventricular mass. In 1957, Anderson and coworkers described the clinical manifestations of Rokitansky’s singular malformation; and 4 years later, Schiebler and coworkers3 changed the term corrected to congenitally corrected to clarify that the correction was a gift of God and not a gift of the surgeon. The reader is referred to three seminal publications.4–6
Transposition of the great arteries is characterized by chambers that are joined concordantly at the atrioventricular junction but discordantly at the ventriculo-great arterial junction (see Chapter 27).7,8 The pulmonary artery arises from a morphologic left ventricle, and the aorta arises from a morphologic right ventricle. The circulations are in parallel rather than in series. Congenitally corrected transposition is characterized by chambers that are joined discordantly at the atrioventricular junction and ventricles that are joined discordantly at the ventriculo-great arterial junction; atrioventricular alignments and ventriculoarterial alignments are both discordant (Figures 6-1 and 6-2).3,4,9 The double discordance—atrioventricular and ventriculoarterial—physiologically corrects the discordance intrinsic to each (see Figure 6-1). Blood from a morphologic right atrium reaches the pulmonary artery by traversing a morphologic mitral valve and a morphologic left ventricle, and blood from a morphologic left atrium reaches the aorta by traversing a morphologic tricuspid valve and a morphologic right ventricle (Figures 6-1 and 6-3).3,4,9 The terms atrioventricular discordance, l-transposition, ventricular inversion, and congenitally corrected transposition are used interchangeably. Atrioventricular discordance requires the presence of two morphologically distinct atria and two morphologically distinct ventricles. Hearts in which two morphologically distinct atria are aligned with one ventricle (univentricular atrioventricular connection) are the subject of Chapter 26.
The terms used in this chapter were defined in Chapter 3 but are repeated here for the reader’s convenience.
Congenitally corrected transposition of the great arteries typically occurs in situs solitus. The estimated prevalence rate is 0.5% of clinically diagnosed congenital malformations of the heart or approximately 1 in 13,000 live births.16,17 Virtually all patients have coexisting cardiac malformations—ventricular septal defect, pulmonary stenosis, abnormalities of the left atrioventricular (AV) valve, and conduction defects.
The coronary artery arrangement is an important morphologic aspect of congenitally corrected transposition.7 The Leiden Convention proposed a means of relating the origins of the coronary arteries to the aortic sinuses from which they originate.7,18 The coronary arteries almost always arise from both, or one or the other, aortic sinuses that are adjacent to the pulmonary trunk7 and are morphologically concordant with the ventricles (i.e., the right coronary artery perfuses the morphologic right ventricle and the left coronary artery perfuses the morphologic left ventricle; Figure 6-4; see Chapter 32).3,4,19–22 Epicardial distribution is a guide to ventricular inversion because the course of the anterior descending artery establishes the location of the ventricular septum. Coronary artery abnormalities are common,22 especially a single coronary artery (see Chapter 32).20
In congenitally corrected transposition of the great arteries, the anterior and leftward ascending aorta and the posterior and rightward pulmonary trunk are parallel and do not cross as in the normal heart3 (see Figures 6-1 and 6-2). The aorta is either convex to the left or ascends vertically but is not border forming on the right. A subaortic conus is responsible for the anterior and leftward position of the ascending aorta and the posterior and medial position of the pulmonary trunk.23 Anatomically corrected malposition refers to an anomaly in which the ascending aorta lies anterior and to the left of the pulmonary trunk in the presence of atrioventricular and ventriculoarterial concordance.23,24
The physiologic consequences of congenitally corrected transposition depend on the functional adequacy of a subaortic morphologic right ventricle and on coexisting congenital malformations.4,16,25 The thick-walled subaortic right ventricle is concordant with a right coronary artery that is designed to perfuse a thin-walled low-resistance right ventricle. A normal subpulmonary right ventricle is designed to serve the low-resistance pulmonary circulation, and its geometry remains unchanged when it is inverted to the subaortic position. Regional strain, twist, and radial wall motion in a subaortic right ventricle differ considerably from a subaortic left ventricle.26 An inverted subaortic right ventricle has a high prevalence of myocardial perfusion defects and abnormalities of regional wall motion.27 A normal subpulmonary right ventricle has a relatively high end-diastolic volume, so normal stroke volumes are achieved with ejection fractions of 35% to 45%.16,25 Importantly, the low ejection fraction does not increase when a morphologic right ventricle is inverted into the subaortic position, so the ejection fraction is considerably less than that of a normal subaortic left ventricle16,25 and response to supine exercise is similar to the response of a noninverted right ventricle.16,25 Ventricular septal defect, pulmonary stenosis, abnormalities of the left AV valve, and conduction defects have a considerable impact on the function of an inherently inadequate inverted right ventricle.3,4
A ventricular septal defect is present in 78% of necropsy cases (Figure 6-5), is usually nonrestrictive perimembranous, and typically extends into the inlet and trabecular septum.4 The inlet septum is poorly aligned with the atrial septum, which results in a malalignment gap that is sometimes filled by tissue from the membranous septum. Pulmonary stenosis or atresia occurs in 50% of cases and represents obstruction to outflow of the morphologic left ventricle.4 The stenosis is isolated in about 20% of cases and occurs with a ventricular septal defect in the remaining 80%. Fixed subpulmonary stenosis4 takes several forms: (1) a fibrous subpulmonary diaphragm attached to the mitral valve, analogous to fixed subaortic stenosis in hearts with noninverted ventricles; (2) aneurysms or fibrous tissue tags that originate from the relatively large membranous septum; and (3) accessory mitral leaflet tissue.3 Subaortic stenosis (obstruction to outflow of the morphologic right ventricle) is caused by anterior deviation of the infundibulum septum or by hypertrophied infundibular muscle bundles.1
Abnormalities of the inverted left atrioventricular valve are present in more than 90% of cases.4 The malformed valve usually functions normally in early life, but an age-related increase in regurgitation is seen. The abnormalities resemble those of Ebstein’s anomaly of a right-sided tricuspid valve in hearts without ventricular inversion (Figure 6-6A),3,4,28 but the anterior leaflet of the inverted Ebstein’s valve is usually small and malformed28 and the atrialized portion of the inverted right ventricle is poorly developed.28 Left atrioventricular valve incompetence is not necessarily caused by an Ebstein’s-like malformation, and the valve is occasionally stenotic rather than incompetent (see Chapter 13). Neonates with severe regurgitation of the inverted atrioventricular valve have an increased incidence of hypoplastic aortic arch, aortic atresia, and aortic coarctation.29 Abnormalities of the right-sided inverted mitral valve have been reported in more than half of necropsy specimens and consist of multiple cusps, multiple or compound papillary muscles, anomalous chordal attachments, and a cleft valve or a common valve.30
History
The male:female ratio is approximately 1.5:1.3 The occurrence of congenitally corrected transposition and complete transposition among first-degree relatives in different families is believed to represent monogenic transmission and implies a pathogenetic link between the two malformations.31,32 Symptoms and clinical course depend chiefly on the presence and degree of coexisting malformations (see previous discussion), but longevity principally hinges on the vulnerability of the subaortic morphologic right ventricle, even with no coexisting malformations.33–35 Infant mortality is related to congestive heart failure. Survival is then relatively constant, with an attrition rate of approximately 1% to 2% year.36 Young patients with isolated congenitally corrected transposition are often overlooked because symptoms are absent and clinical signs are subtle.3 The diagnosis may come to light because of abnormalities in an x-ray (Figure 6-7) or an electrocardiogram (Figure 6-20) or because of symptomatic complete heart block (Figure 6-8).3,37,38 High-degree heart block rarely occurs in utero but is occasionally present shortly after birth (see Figure 6-8),39,40 or may announce itself later as a Stokes-Adams attack or sudden death.39,41 The age-related risk of development of complete heart block is about 2% per year.39 Left atrioventricular valve regurgitation is closely coupled to long-term survival.36,38 The regurgitation is usually occult in infants, so late appearance prompts a mistaken diagnosis of acquired mitral regurgitation.
Survival to the sixth or seventh decade is infrequent,3,17,21,33,42,43 but two patients reached their eighth decade.36,44 In isolated congenitally corrected transposition, failure of the subaortic right ventricle is uncommon but not rare and may occur during pregnancy in previously asymptomatic women.45 Myocardial perfusion defects are prevalent.27 Angina pectoris is attributed to a supply-demand imbalance between a thick-walled systemic right ventricle and its blood supply from a morphologic right coronary artery (see previous discussion).
The ventricular septal defect that accompanies congenitally corrected transposition is typically nonrestrictive with a clinical course analogous to a ventricular septal defect of analogous size in normally formed hearts (see Chapter 17).3 Pulmonary stenosis exerts a protective effect by curtailing excessive pulmonary blood flow. An inverted subpulmonary left ventricle adapts to the systemic systolic pressure incurred by a nonrestrictive ventricular septal defect. Isolated pulmonary stenosis varies from mild to severe and has a clinical course analogous to equivalent pulmonary stenosis in hearts without ventricular inversion (see Chapter 11).
Jugular venous pulse
PR interval prolongation is recognized by an increase in the interval between the jugular A wave and the carotid pulse, two-to-one heart block is recognized by A waves that occur with twice the frequency of the carotid pulse, and complete heart block is recognized by independent jugular A waves and random cannon A waves (see Chapter 4).46
Pulmonary stenosis is associated with A wave amplitudes that vary with the severity, as in isolated pulmonary stenosis in hearts without ventricular inversion (see Chapter 11). Pulmonary stenosis with a nonrestrictive ventricular septal defect is accompanied by a normal jugular venous pulse as in Fallot’s tetralogy (see Chapter 18).
Precordial movement and palpation
Precordial movements are influenced by the spatial orientation of the ventricular septum and by the anterior and leftward position of the ascending aorta. The plane of the ventricular septum faces forward, and a vertical interventricular sulcus is closely aligned with the left sternal border (Figure 6-6B).3,47 Accordingly, an inverted right ventricle occupies an anterior and leftward position, with its medial border adjacent to the left sternal edge and its lateral border at the apex (see Figure 6-2B), an arrangement that accounts for the large topographic area occupied by the right ventricular impulse, which is accentuated by left atrioventricular valve regurgitation and systolic expansion of the left atrium that causes anterior displacement of the heart (see Figure 6-6A). No retraction exists medial to the interventricular sulcus because the sulcus lies too close to the left sternal border (see Figure 6-6B). The inverted left ventricle occupies a posterior and rightward position behind the sternum3 and cannot be palpated even in the presence of pulmonary hypertension or pulmonary stenosis (see Figures 6-2A and 6-6B). The ascending aorta is palpated when dilated and convex to the left (see Figure 6-5). The aortic component of the second heart sound is palpated because it is accentuated by the anterior position of the aortic root (Figures 6-5 and 6-9).
Auscultation
A soft first heart sound reflects the PR interval prolongation of first-degree heart block, and variation in intensity is a sign of complete heart block (see Chapter 4).46 An ejection sound at the left base (Figure 6-13B) originates in the anterior aorta, especially when the aortic root is dilated (see Figure 6-5). A short soft basal midsystolic murmur originates in the anterior aorta but may also originate in the posterior pulmonary trunk across the deeply wedged outflow tract of the morphologic left ventricle. The second heart sound is loud because the aortic valve is anterior (see section Palpation; see Figure 6-13B).3,48 The loudness and location of the sound invite the mistaken diagnosis of pulmonary hypertension. The pulmonary component is attenuated by the posterior position of the pulmonary trunk, which makes splitting difficult to detect. When splitting is detected, it is heard in the second left interspace, despite the rightward position of the posterior pulmonary valve.49 A soft, high-frequency, early diastolic murmur at the mid left sternal border is more likely to be a Graham Steell murmur (Figure 6-10) than an early diastolic murmur of aortic regurgitation. The ventricular septal defect murmur is holosystolic (see Figure 6-10) or decrescendo (Figure 6-11), is absent when the shunt is reversed, and is analogous in location, configuration, and quality to the murmur of ventricular septal defect in hearts without ventricular inversion (see Chapter 17). A mid-diastolic murmur is generated by increased flow across the left atrioventricular valve when the left-to-right shunt is large (see Figure 6-11). A pulmonary ejection sound originates in a dilated hypertensive posterior pulmonary trunk (see Figures 6-5 and 6-10A). The intensity of an inherently loud aortic component of the second heart sound is augmented by pulmonary hypertension because the increased pulmonary component is synchronous with the prominent component from the anterior aortic valve (see Figures 6-10 and 6-11).
The murmur of pulmonary stenosis is maximal in the third left intercostal space because the stenosis is usually subpulmonary (Figure 6-12). It is relatively soft for a given degree of obstruction because of the attenuating effect of the anterior aorta. Radiation of the murmur is upward and to the right because the pulmonary trunk is oriented upward and to the right3 (see Figure 6-2A). The loud second heart sound at the left base is aortic not pulmonary (see Figure 6-12).
Left atrioventricular valve regurgitation generates a systolic murmur analogous to mitral regurgitation in hearts without ventricular inversion (Figure 6-13).13 Radiation tends to be toward the left sternal edge rather than into the axilla because the malformed tricuspid leaflets direct the jet medially within the left atrium. The first heart sound is not loud because in left-sided Ebstein’s anomaly with congenitally corrected transposition, the malformed anterior tricuspid leaflet is small and poorly mobile.28
Electrocardiogram
In 1913, Monckeberg50 described an anterior node that was responsible for atrioventricular conduction in congenitally corrected transposition of the great arteries. Walmsley51 in 1931 and Yater, Lyon, and Mcnabb52 in 1933 established that the bundle branches were inverted. Elegant studies by Lev and Anderson53 were major steps forward in advancing our knowledge of conduction tissues in hearts with ventricular inversion.
Because the atrial septum is malaligned with the inlet ventricular septum, the regular AV node does not make contact with infranodal right and left bundle branches. Instead, an anomalous anterior AV node with a bundle penetrates the atrioventricular fibrous annulus and descends onto the anterior aspect of the ventricular septum.54 The penetrating atrioventricular bundle descends for a long distance down the septal surface before branching. The long penetrating atrioventricular bundle is well-formed in hearts of young children, but beginning in adolescence, the conduction fibers are replaced with fibrous tissue, which is responsible for atrioventricular block. Electrophysiologic studies have identified multiple levels of conduction defects that include the AV node, the penetrating bundle, and the bundle branches.41 Complete heart block that is present at birth (see Figure 6-8)54 results from discontinuity between the anterior AV node and the ventricular septum. A cordlike right bundle branch extends leftward to the morphologic right ventricle, and a left bundle branch descends down the septal surface of the morphologic left ventricle. The right bundle branch is concordant with the morphologic right ventricle, and the left bundle branch is concordant with the morphologic left ventricle. Ebstein’s anomaly of left atrioventricular valve with left-sided accessory pathways provides the substrate for preexcitation between the morphologic left atrium and the morphologic right ventricle.55 The arrhythmogenic atrialized morphologic right ventricle resides in the left side of the heart.
The scalar electrocardiogram exhibits three major features: 1, disturbances in conduction and rhythm; 2, QRS and T wave patterns that reflect ventricular inversion; and 3, modifications of the P wave, QRS, ST segment, and T wave caused by coexisting congenital heart disease. The P wave is normal in direction and configuration because the sinoatrial node is in its normal location, so the atria are normally activated. Broad notched left atrial P waves occur when the left atrium is volume overloaded by incompetence of the left atrioventricular valve or by a large left-to-right shunt through a ventricular septal defect (Figure 6-14). Tall peaked right atrial P waves occur with pulmonary hypertension (Figure 6-15) or pulmonary stenosis (Figure 6-16).
Atrioventricular conduction ranges from PR interval prolongation of first-degree heart block (see Figure 6-16) to complete heart block (Figures 6-8 and 6-17). More than 75% of patients with congenitally corrected transposition exhibit varying degrees of AV block when all ages are included, and the overall incidence rate of complete heart block is about 30%.40,54,56 Normal conduction in the surface electrocardiogram coincides with normal conduction in intracardiac electrophysiologic studies and vice versa. The His electrogram does not necessarily localize the site of AV block because the long course of the nonbranching bundle precludes distinction between a distal AV nodal lesion and a proximal His bundle lesion.54 Second-degree AV block is almost always 2/1. The degree of block varies from time to time in the same patient, and first-degree block can change to intermittent 2/1 block to complete heart block. Complete heart block is associated with a normal sequence of ventricular activation and a normal QRS duration (see Figures 6-8 and 6-17),41 as is the case in isolated congenital complete heart block (see Chapter 4).
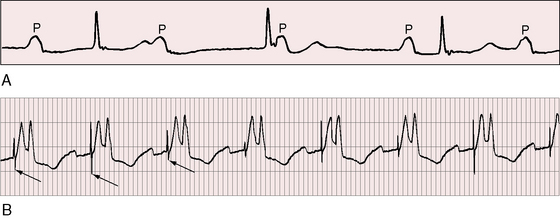
Figure 6-17 Monitor leads from a 41-year-old man with congenitally corrected transposition of the great arteries whose coronary arteriograms are shown in Figure 6-4. A, The rhythm strip identifies complete disassociation between P waves and QRS complexes that are of normal duration. B, Rhythm strip after insertion of a pacemaker into the subpulmonary left ventricle. Arrows identify the ventricular pacemaker spikes.
Accelerated conduction through bypass tracts occurs in congenitally corrected transposition with Ebstein’s anomaly of the left AV valve. Delta waves in lead V1 indicate left bypass tracts,54,55 and fusion beats indicate dual antegrade conduction via a bypass tract and an anterior AV node.55 When discontinuity exists beyond the anterior AV node, antegrade conduction is occasionally achieved via an accessory pathway.55 Supraventricular tachycardia, atrial fibrillation, and atrial flutter with left-sided Ebstein’s anomaly do not necessarily coincide with the presence of Wolff-Parkinson-White accessory pathways.54 Chronic atrial fibrillation occurs with chronic severe incompetence of the left atrioventricular valve.
QRS and T wave patterns are to be considered in light of the inverted right and left bundle branches and the coexisting cardiac malformations.57 Activation of the ventricular septum in the normal heart proceeds from left to right, so Q waves appear in left precordial leads and an initial R wave appears in right precordial leads. In congenitally corrected transposition, the opposite is the case. Inversion of the right and left bundle branches results in reversed septal activation that proceeds from right to left. Q waves appear in right precordial leads (Figures 6-16 and 6-18 through 6-20) and are absent in left precordial leads (see Figures 6-14, 6-15, and 6-18 through 6-20). Absence of left precordial Q waves stands out in bold relief in the presence of volume overload of the systemic ventricle (see Figures 6-14 and 6-15). Presence or absence of left precordial Q waves can be confirmed with recording leads beyond the V6 position (see Figure 6-14). Q waves in leads contiguous to lead V1 can be detected in leads V3R and V4R. When supplementary lead placements are used, reversed septal activation is found in virtually all patients. An occasional R wave in lead V1 (see Figures 6-14 and 6-15) should not detract from the importance of consistent absence of Q waves in left precordial leads (see Figures 6-16 and 6-18 through 6-20). Septal activation in a superior direction is responsible for Q waves in leads 3 and aVF and for almost uniform absence of Q waves in leads 1 and aVL (see Figures 6-15 and 6-18 through 6-20).3 Q waves are consistently deeper in lead 3 than in lead aVF and not uncommonly are very deep (see Figures 6-15 and 6-18 through 6-20).
Left axis deviation is diagnostically important (see Figures 6-18 through 6-20).48,57 The mechanism responsible for the left superior axis is unsettled but cannot be the result of left anterior fascicular block because the left bundle branch is in the right side of the heart. In most cases, the atrioventricular conduction axis originates from an anomalous atrioventricular node rather than the normal atrioventricular node located at the apex of the triangle of Koch.58 Left axis deviation is most likely when systolic pressure in the subpulmonary left ventricle is not elevated (see Figures 6-18 through 6-20) and is least likely when systolic pressure is high (see Figures 6-14 and 6-15).48,57 A left superior axis is occasionally associated with striking Q waves in leads 2, 3, and aVF and in Q waves in most if not all precordial leads (Figures 6-18, 6-20, and 6-21).
In more than 80% of cases, T waves are positive in all six precordial leads (see Figures 6-19, 6-20, and 6-21), a distinctive feature attributed to the side-by-side relationship of the inverted ventricles (see Figure 6-6). T waves are often taller in right precordial leads (see Figures 6-14, 6-17, 6-20, and 6-21).
Severe pulmonary stenosis with intact ventricular septum may produce a qR complex in lead V1 and an rS complex in lead V6.57 Nonrestrictive ventricular septal defects with large left-to-right shunts are associated with large equidiphasic RS complexes in mid-precordial leads and with increased R waves without Q waves in left precordial leads (see Figures 6-14 and 6-15).57
X-ray
The normal heart in a posteroanterior chest x-ray is characterized by a distinctive triad of contours that consists of the ascending aorta on the right and the aortic knuckle and pulmonary trunk on the left. In congenitally corrected transposition, this triad is lost because the aorta does not ascend on the right and the pulmonary trunk is not border forming on the left (see Figure 6-2).59 The most common relationship of the great arteries is side by side with the ascending aorta anterior and to the left of a medially and posteriorly positioned pulmonary trunk (see Figure 6-2). These relationships can usually be recognized even when subtle. The ascending aorta at the left base varies from absent (Figures 6-22 and 6-23) to straight (see Figure 6-7) to gently concave (see Figure 6-22) to moderately convex (Figure 6-24) to strikingly convex (Figure 6-9).9,59 Less commonly, the ascending aorta rises vertically and anterior to the posterior pulmonary trunk, so neither great artery is border forming (see Figure 6-23).
The posterior and rightward pulmonary trunk tilts the right branch upward and the left branch downward, so that the two branches are at the same level (see Figure 6-6B).48,59 A dilated posterior pulmonary trunk can displace the superior vena cava to the right, forming a right basal shadow (see Figure 6-24)59 or may project as a right basal convexity that can be mistaken for the ascending aorta (Figure 6-25).9 The leftward position of the aortic arch and proximal descending aorta produce a relatively bold indentation on the left side of the esophagus (see Figure 6-7).59
The silhouette of the morphologic right ventricle has two distinctive features: (1) a hump-shaped appearance caused by a prominent inverted infundibulum (see Figures 6-2B, 6-22, and 6-23);59 and (2) a septal notch, which is a subtle indentation just above the left hemidiaphragm corresponding to the apical position of the interventricular groove (see Figures 6-22 and 6-24). The hump-shaped infundibular shadow occupies the site of the left atrial appendage (see Figures 6-2 and 6-23),3 so left atrial enlargement is best identified in a lateral projection. A giant left atrium is presented as a huge ball suspended below a narrow vascular pedicle (Figure 6-26).
Echocardiogram
Echocardiography is used to identify atrioventricular and ventriculoarterial discordance, the morphologic right ventricle equipped with a tricuspid valve, the morphologic left ventricle equipped with a mitral valve, and the spatial relationships of the great arteries and their ventricles of origin, in addition to coexisting congenital heart disease. Echocardiographic criteria for determination of the morphology of a ventricular chamber and its atrioventricular valve include the level of attachment of each valve to the ventricular septum, valve leaflet configuration (bileaflet or trileaflet), papillary muscle arrangements, the presence of chordal attachments to the septum (tricuspid valve) or to the ventricular free wall (mitral valve), the type of ventricular trabeculations, the ventricular shape, and the presence or absence of fibrous continuity between mitral valve and a great artery.60 A morphologic left ventricle is recognized by its ovoid or ellipsoid shape and its fine trabecular architecture (Figure 6-27), by an AV valve that inserts into the ventricular septum at a level more proximal than the septal insertion of the contralateral AV valve, by a bicommissural valve with a fish mouth appearance in diastole, by paired papillary muscles, by chordae tendineae that insert only into the ventricular free wall, and by continuity between an AV valve and a great artery. A morphologic right ventricle is recognized by its crescent shape and its coarse trabecular architecture, by a moderator band, by relatively distal insertion of the AV valve into the ventricular septum, by a tricommissural valve, by multiple irregular papillary muscles with chordal attachments to the ventricular septum, and by discontinuity between an AV valve and a great artery.
The crossed or spiral great arterial relationships of the normal heart (see Figure 6-1) are imaged in the short axis as a posterior circle, which is the aorta, and an anterior sausage, which is the right ventricular outflow tract and main pulmonary artery transected tangentially. The transposed great arteries of congenitally corrected transposition do not cross as in the normal heart (see Figure 6-1) and are imaged in the short axis as double circles that represent cross sections of the anterolateral aorta and poteromedial pulmonary trunk (Figure 6-28). Long-axis views image the aortic root and pulmonary trunk parallel to each other. The pulmonary trunk is identified by its bifurcation into right and left branches (Figure 6-29 and Videos 6-1 and 6-2), and the aorta is identified by its brachocephalic branches.
A ventricular septal defect is typically nonrestrictive and perimembranous with extension into the inlet septum (Figure 6-29; see Chapter 17). When the defect is large, the relative levels of AV valve attachments to the ventricular septum are unreliable guides to ventricular inversion. Malformations of the left atrioventricular valve include Ebstein’s anomaly (Figure 6-30 and Video 6-2) and multiple short chordae tendineae that attach to the septum and apex (see Chapter 13). Color flow imaging is used to determine the degree of left AV valve regurgitation.61 Echocardiography with Doppler interrogation characterizes obstruction to outflow of the subpulmonary left ventricle.4 Subpulmonary stenosis is caused by a fixed fibrous subpulmonary diaphragm, fibrous tags, a membranous septal aneurysm, or accessory mitral valve tissue.4
Echocardiography defines the different types of criss-cross hearts and superoinferior ventricles (Figures 6-31 and 6-32).10 Atrioventricular discordance and ventriculoarterial discordance result in the physiology of congenitally corrected transposition.10,15 Atrioventricular concordance with ventriculoarterial discordance and isolated ventricular inversion result in the physiology of complete transposition (see Figures 6-31 and 6-32).
1 Rokitansky K.F. Die Defekte der Scheidewande des Herzens. Vienna: W. Braumuller; 1875.
2 Baille M. The morbid anatomy of the most important parts of the human body, 2nd ed. London: Johnson and Nicol; 1797.
3 Schiebler G.L., Edwards J.E., Burchell H.B., Dushane J.W., Ongley P.A., Wood E.H. Congenital corrected transposition of the great vessels: a study of 33 cases. Pediatrics. 1961;27((5)Suppl):849-888.
4 Allwork S.P., Bentall H.H., Becker A.E., et al. Congenitally corrected transposition of the great arteries: morphologic study of 32 cases. Am J Cardiol. 1976;38:910-923.
5 Becker A.E., Anderson R.H. Conditions with discordant atrioventricular connections. In: Anderson R.H., Shinebourne E.A., editors. Pediatric cardiology. London: Churchill Livingstone, 1978.
6 Kurosawa H., Imai Y., Becker A.E. Congenitally corrected transposition with normally positioned atria, straddling mitral valve, and isolated posterior atrioventricular node and bundle. J Thorac Cardiovasc Surg. 1990;99:312-313.
7 Anderson R.H. Transposition—introduction. Cardiol Young. 2005;15(Suppl 1):72-75.
8 Anderson R.H., Weinberg P.M. The clinical anatomy of transposition. Cardiol Young. 2005;15(Suppl 1):76-87.
9 Lester R.G., Anderson R.C., Amplatz K., Adams P. Roentgenologic diagnosis of congenital corrected transposition of great vessels. Am J Roentgenol. 1960;83:985-997.
10 Pasquini L., Sanders S.P., Parness I., et al. Echocardiographic and anatomic findings in atrioventricular discordance with ventriculoarterial concordance. Am J Cardiol. 1988;62:1256-1262.
11 Quero-Jimenez M., Raposo-Sonnenfeld I. Isolated ventricular inversion with situs solitus. Br Heart J. 1975;37:293-304.
12 Foran R.B., Belcourt C., Nanton M.A., et al. Isolated infundibuloarterial inversion (S,D,I): a newly recognized form of congenital heart disease. Am Heart J. 1988;116:1337-1350.
13 Perloff J.K., Roberts W.C. The mitral apparatus. Functional anatomy of mitral regurgitation. Circulation. 1972;46:227-239.
14 Anderson R.H., Shinebourne E.A., Gerlis L.M. Criss-cross atrioventricular relationships producing paradoxical atrioventricular concordance or discordance. Their significance to nomenclature of congenital heart disease. Circulation. 1974;50:176-180.
15 Hery E., Jimenez M., Didier D., et al. Echocardiographic and angiographic findings in superior-inferior cardiac ventricles. Am J Cardiol. 1989;63:1385-1389.
16 Benson L.N., Burns R., Schwaiger M., et al. Radionuclide angiographic evaluation of ventricular function in isolated congenitally corrected transposition of the great arteries. Am J Cardiol. 1986;58:319-324.
17 Ikeda U., Furuse M., Suzuki O., Kimura K., Sekiguchi H., Shimada K. Long-term survival in aged patients with corrected transposition of the great arteries. Chest. 1992;101:1382-1385.
18 Gittenberger-De Groot A.C., Sauer U., Quaegebeur J. Aortic intramural coronary artery in three hearts with transposition of the great arteries. J Thorac Cardiovasc Surg. 1986;91:566-571.
19 Mckay R., Anderson R.H., Smith A. The coronary arteries in hearts with discordant atrioventricular connections. J Thorac Cardiovasc Surg. 1996;111:988-997.
20 Dabizzi R.P., Barletta G.A., Caprioli G., Baldrighi G., Baldrighi V. Coronary artery anatomy in corrected transposition of the great arteries. J Am Coll Cardiol. 1988;12:486-491.
21 Schwartz H.A., Wagner P.I. Corrected transposition of the great vessels in a 55-year-old woman; diagnosis by coronary angiography. Chest. 1974;66:190-192.
22 Ismat F.A., Baldwin H.S., Karl T.R., Weinberg P.M. Coronary anatomy in congenitally corrected transposition of the great arteries. Int J Cardiol. 2002;86:207-216.
23 Colli A.M., De Leval M., Somerville J. Anatomically corrected malposition of the great arteries: diagnostic difficulties and surgical repair of associated lesions. Am J Cardiol. 1985;55:1367-1372.
24 Chen M.-R. Anatomically corrected malposition of the great arteries. Pediatr Cardiol. 2008;29:467-468.
25 Graham T.P.Jr, Parrish M.D., Boucek R.J.Jr, et al. Assessment of ventricular size and function in congenitally corrected transposition of the great arteries. Am J Cardiol. 1983;51:244-251.
26 Fogel M.A., Weinberg P.M., Fellows K.E., Hoffman E.A. A study in ventricular-ventricular interaction. Single right ventricles compared with systemic right ventricles in a dual-chamber circulation. Circulation. 1995;92:219-230.
27 Hornung T.S., Bernard E.J., Jaeggi E.T., Howman-Giles R.B., Celermajer D.S., Hawker R.E. Myocardial perfusion defects and associated systemic ventricular dysfunction in congenitally corrected transposition of the great arteries. Heart. 1998;80:322-326.
28 Anderson K.R., Zuberbuhler J.R., Anderson R.H., Becker A.E., Lie J.T. Morphologic spectrum of Ebstein’s anomaly of the heart: a review. Mayo Clin Proc. 1979;54:174-180.
29 Celermajer D.S., Cullen S., Deanfield J.E., Sullivan I.D. Congenitally corrected transposition and Ebstein’s anomaly of the systemic atrioventricular valve: association with aortic arch obstruction. J Am Coll Cardiol. 1991;18:1056-1058.
30 Gerlis L.M., Wilson N., Dickinson D.F. Abnormalities of the mitral valve in congenitally corrected transposition (discordant atrioventricular and ventriculoarterial connections). Br Heart J. 1986;55:475-479.
31 Digilio M.C., Casey B., Toscano A., et al. Complete transposition of the great arteries: patterns of congenital heart disease in familial precurrence. Circulation. 2001;104:2809-2814.
32 Piacentini G., Digilio M.C., Capolino R., et al. Familial recurrence of heart defects in subjects with congenitally corrected transposition of the great arteries. Am J Med Genet. 2005;137:176-180. Part A
33 Graham T.P.Jr, Bernard Y.D., Mellen B.G., et al. Long-term outcome in congenitally corrected transposition of the great arteries: a multi-institutional study. J Am Coll Cardiol. 2000;36:255-261.
34 Skinner J., Hornung T., Rumball E. Transposition of the great arteries: from fetus to adult. Heart. 2008;94:1227-1235.
35 Warnes C.A. Transposition of the great arteries. Circulation. 2006;114:2699-2709.
36 Huhta J.C., Danielson G.K., Ritter D.G., Ilstrup D.M. Survival in atrioventricular discordance. Pediatr Cardiol. 1985;6:57-60.
37 Berman D.A., Adicoff A. Corrected transposition of the great arteries causing complete heart block in an adult. Treatment with an artificial pacemaker. Am J Cardiol. 1969;24:125-129.
38 Beauchesne L.M., Warnes C.A., Connolly H.M., Ammash N.M., Tajik A.J., Danielson G.K. Outcome of the unoperated adult who presents with congenitally corrected transposition of the great arteries. J Am Coll Cardiol. 2002;40:285-290.
39 Huhta J.C., Maloney J.D., Ritter D.G., Ilstrup D.M., Feldt R.H. Complete atrioventricular block in patients with atrioventricular discordance. Circulation. 1983;67:1374-1377.
40 Walker W.J., Cooley D.A., Mc N.D., Moser R.H. Corrected transposition of the great vessels, atrioventricular heart block, and ventricular septal defect: a clinical triad. Circulation. 1958;17:249-254.
41 Gillette P.C., Busch U., Mullins C.E., Mcnamara D.G. Electrophysiologic studies in patients with ventricular inversion and “corrected transposition”. Circulation. 1979;60:939-945.
42 Benchimol A., Sundararajan V. Congenital corrected transposition of the great vessels in a 58-year-old man. Chest. 1971;59:634-638.
43 Milici C., Bovelli D., Forlani D., et al. Images in cardiovascular medicine. An unusual case of congenitally corrected transposition of the great arteries in the elderly. Circulation. 2008;117:e485-e489.
44 Lieberson A.D., Schumacher R.R., Childress R.H., Genovese P.D. Corrected transposition of the great vessels in a 73-year-old man. Circulation. 1969;39:96-100.
45 Connolly H.M., Grogan M., Warnes C.A. Pregnancy among women with congenitally corrected transposition of great arteries. J Am Coll Cardiol. 1999;33:1692-1695.
46 Perloff J.K. Physical examination of the heart and circulation, 4th ed. Shelton, Connecticut: People’s Medical Publishing House; 2009.
47 Kraus Y., Yahini J.H., Shem-Tov A., Neufeld H.N. Precordial pulsations in corrected transposition of the great vessels. Diagnostic value of the electromechanical interval. Am J Cardiol. 1969;23:684-689.
48 Cumming G.R. Congenital corrected transposition of the great vessels without associated intracardiac anomalies. A clinical, hemodynamic and angiographic study. Am J Cardiol. 1962;10:605-614.
49 Gasul B.M., Graettinger J.S., Bucheleres G. Corrected transposition of the great vessels; demonstration of a new phonocardiographic sign of this malformation. J Pediatr. 1959;55:180-188.
50 Monckeberg J.G. Zur Entwicklungsgeschichte des Atrioventrikular-systems. Verh Dtsch Pathol Ges. 1913;16:228.
51 Walmsley T. Transposition of the ventricles and the arterial stems. J Anat. 1931;65:528-540.
52 Yater W.M., Lyon J.A., Mcnabb P. Congenital heart block: review and report of the second case of complete heart block studied by serial sections through the conduction system. JAMA. 1933;100:1831.
53 Anderson R.H., Becker A.E., Arnold R., Wilkinson J.L. The conducting tissues in congenitally corrected transposition. Circulation. 1974;50:911-923.
54 Daliento L., Corrado D., Buja G., John N., Nava A., Thiene G. Rhythm and conduction disturbances in isolated, congenitally corrected transposition of the great arteries. Am J Cardiol. 1986;58:314-318.
55 Bharati S., Rosen K., Steinfield L., Miller R.A., Lev M. The anatomic substrate for preexcitation in corrected transposition. Circulation. 1980;62:831-842.
56 Marino B., Sanders S.P., Parness I.A., Colan S.D. Obstruction of right ventricular inflow and outflow in corrected transposition of the great arteries (S,L,L): two-dimensional echocardiographic diagnosis. J Am Coll Cardiol. 1986;8:407-411.
57 Victorica B.E., Miller B.L., Gessner I.H. Electrocardiogram and vectorcardiogram in ventricular inversion (corrected transposition). Am Heart J. 1973;86:733-744.
58 Anderson R.H. The conduction tissues in congenitally corrected transposition. Ann Thorac Surg. 2004;77:1881-1882.
59 Carey L.S., Ruttenberg H.D. Roentgenographic features of congenital corrected transposition of the great vessels: a comparative study of 33 cases with a roentgenographic classification based on the associated malformations and hemodynamic states. Am J Roentgenol Radium Ther Nucl Med. 1964;92:623-651.
60 Meissner M.D., Panidis I.P., Eshaghpour E., Mintz G.S., Ross J. Corrected transposition of the great arteries: evaluation by two-dimensional and Doppler echocardiography. Am Heart J. 1986;111:599-601.
61 Lynch K.P.3rd, Yan D.C., Sharma S., Dhar P.K., Fyfe D.A. Serial echocardiographic assessment of left atrioventricular valve function in young children with ventricular inversion. Am Heart J. 1998;136:94-98.