CHAPTER 27 Congenital Percutaneous Interventions
ATRIAL SEPTAL DEFECT
Description and Special Anatomic Considerations
Secundum atrial septal defect (ASD), one of the more common congenital heart defects, represents 6% to 10% of all congenital anomalies, occurring in 1 in 1500 live births.1 Secundum ASDs are due to absence, perforation, or deficiency of the septum primum. This defect typically occurs sporadically but has been linked to genetic abnormalities such as Holt-Oram syndrome and mutations on chromosome 5p.
Device closure of an ASD was first performed in 1974 by King and Mills2 with a 24-gauge surgically placed femoral sheath and a double-sided disk device. Technology and technique have been modified and refined over the years; however, the procedure remains conceptually identical. A collapsible double-sided disk device with a metal frame and fabric patches is positioned antegrade through a long femoral sheath (6F to 10F) across the secundum ASD. On extrusion from the sheath, the device expands, creating a patch on both sides of the septum, clamping the rim of tissue surrounding the ASD. The endocardium grows in to cover the device to create a permanent seal. Because of the need for surrounding rim tissue, device closure is limited to secundum-type defects and is not applicable to either primum (no inferior posterior rim) or venosus (no superior rim) ASDs. With recent technologic advances, device closure has rapidly become the treatment of choice for secundum ASDs.
Indications
Device closure is indicated for any size secundum ASD with evidence on echocardiography of right ventricular volume overload. ASD should also be closed in patients with symptoms of exercise intolerance or history of cryptogenic stroke. There is mounting evidence that ASD closure, even in the elderly, can improve maximal oxygen consumption.3 ASDs have been closed by device in small children, including infants; however, the most common timing for elective closure appears to be between 2 and 4 years of age.
Outcomes and Complications
Concurrent controlled trials comparing surgical closure with device closure have shown efficacy rates of more than 96% with significantly lower complication rates and hospital stay.4 Most patients can be discharged on the day of the procedure, with return to full activity within 48 to 72 hours, significantly reducing costs and medical resources.5 Early complications have been minor and occur in fewer than 9% of patients; they consist primarily of transient arrhythmias, vascular injury, and asymptomatic device embolization. Serious complications have been rare but include thrombus formation on the device, heart block requiring pacing, and cardiac perforation.6
Imaging Findings
Preoperative Planning
When an ASD has been diagnosed, complete transthoracic echocardiography should be performed to evaluate the suitability for device closure. This includes specific attention to the pulmonary vein drainage as well as to the size and location of the defect, including tissue rims to the atrioventricular valves, inferior vena cava, right pulmonary veins, aortic valve, and roof of the atrium. If the transthoracic study is inadequate to delineate these structures, OmniPlane transesophageal echocardiography should be performed. Documentation of an adequate atrial septal rim circumferentially (>3 mm, especially at the posterior inferior inlet portion; Fig. 27-1) and evaluation for additional defects, tissue strands, or septal aneurysms with perforations are essential (Fig. 27-2). Identification of all pulmonary veins, particularly the right upper, is essential because of the association of partial anomalous pulmonary venous return with sinus venosus ASD.
There is current interest in the use of three-dimensional echocardiography as well as MRI for the preprocedure evaluation of ASD. Certainly, these modalities improve detection of an anomalous pulmonary vein and give a more complete understanding of the shape of the defect. This may permit more accurate measurement of the long-axis dimension of the defect, which is helpful for choosing the appropriate type and size of device. Although these modalities give additional information, their clinical advantage in typical ASDs is not proved, and they do add to cost and medical service use. In patients with unusual anatomy, they are invaluable (Fig. 27-3).
Procedural imaging for device implantation is a combination of echocardiography and biplane fluoroscopy. In my practice, I use surface echocardiography for implantation in children younger than 6 years (Fig. 27-4) and intracardiac echocardiography for older patients (Fig. 27-5). Transesophageal echocardiography is used in older patients in some institutions; however, it necessitates general anesthesia for the procedure. Balloon sizing of the defect is often done and can be useful to detect multiple defects (Fig. 27-6). There has been experimental animal work with active MRI for device implantation (Fig. 27-7), although there has been no human clinical application to date.
Postoperative Surveillance
The cornerstone of surveillance after implantation remains transthoracic echocardiography. Patients are seen 1, 6, and 12 months after implantation to ensure appropriate device position, to rule out thrombus formation, and to assess right ventricular size and function. In most patients with right ventricular dilation, ventricular size returns to normal by 12 months after implantation. Commonly used devices have different imaging properties by echocardiography and radiography (Fig. 27-8). There have been frame fractures after implantation in certain devices (STARFlex and HELEX); therefore, chest radiography or, if necessary, fluoroscopy is needed at 6 to 12 months of follow-up (Fig. 27-9). MRI (Fig. 27-10) and CT can be used to image septal devices after implantation but have not shown additional clinical utility to date.
PATENT FORAMEN OVALE
Description and Special Anatomic Considerations
Device closure of patent foramen ovale (PFO) was first described in 19877 for the prevention of recurrent stroke associated with paradoxical embolus.8 It has also been used to prevent right-to-left shunting causing desaturation in patients with orthodeoxia-platypnea syndrome.9 The foramen ovale is a flap valve in the atrial septum created by overlap of the superior anterior septum secundum on the inferior posterior septum primum (Fig. 27-11). It is present in all fetuses during development to direct oxygenated venous return from the placenta through the inferior vena cava across the atrial septum, bypassing the right ventricle and unexpanded lungs, to fill the left ventricle, allowing optimal cerebral perfusion. After birth, with redistribution of flow due to lung expansion resulting in an increased left atrial pressure, the PFO closes and seals permanently in 65% to 80% of people, age dependent.10 However, in 20% to 35% of the normal population, the foramen ovale does not fibrose closed and remains patent, allowing unidirectional flow from right to left if right atrial pressure exceeds left atrial pressure. This is physiologically insignificant for most people unless the amount of right-to-left shunting is significant, causing orthodeoxia-platypnea syndrome, or an embolus crosses right to left, resulting in a cryptogenic transient ischemic attack or stroke. Approximately 55% of patients who have had a stroke have a PFO,11 suggesting that it plays an important role in some of these patients.
During the last 14 years, interventional device closure of PFO has become an attractive alternative therapeutic strategy to surgical PFO closure or lifelong anticoagulation for stroke prevention. No controlled comparative studies with these other treatment strategies exist for PFO closure, although several active multicenter protocols in stroke patients are currently comparing device closure with medical therapy for prevention of recurrent stroke. Good comparative data from the ASD literature suggest that the efficacy of device closure of ASD is similar to that of surgical closure, with a significant reduction in complications, hospital stay, recovery time, and medical resource use.4
Outcomes and Complications
Procedural success with PFO device closure is 98% to 100%, with complete closure rates of 51% to 96% at 6 months on evaluation by saline contrast transesophageal echocardiography.12,13 Recurrent neurologic event risk after PFO device closure is 1% to 2% annually, with a 96% 1-year and a 90% to 94% 5-year event-free rate.12,13 These results are significantly influenced by selection of patients because some patients who undergo device closure may have recurrent strokes unrelated to either the PFO or the device. More definitive information about recurrent stroke risk will be available from controlled randomized trials now under way comparing device closure with medical therapy. Procedural complications are uncommon, occurring in fewer than 2% of patients; they include stroke, transient ischemic attack, transient myocardial ischemia (these three due to air or clot embolism with the large delivery sheaths in the left atrium), device malposition or embolization, cardiac perforation with tamponade, and local femoral vein injury.12 Late complications include atrial arrhythmias in 4% of patients, although most are mild and require no treatment,14 and thrombus formation on the device.
Imaging Findings
Preoperative Planning
Because most patients undergo PFO device closure for prevention of stroke recurrence, it is essential to evaluate the patient’s prior neurologic events and to ensure that they were cryptogenic and likely related to the PFO. Stroke associated with paradoxical embolism is a diagnosis of exclusion, so it is imperative to rule out other potential causes of stroke including cerebral aneurysm, carotid or vertebral vessel abnormalities, atrial arrhythmias, left atrial appendage thrombus, cardiomyopathy, and a hypercoagulable state. Standard pre–device closure evaluation includes head and neck MRI or MRA; carotid ultrasonography; saline contrast transesophageal echocardiography with Valsalva maneuver (Fig. 27-12); and hypercoagulable screen, including proteins C and S, antithrombin III, factor V Leiden, prothrombin 20210, methylenetetrahydrofolate reductase (MTHFR), anticardiolipin antibody, and homocysteine. This work-up is essential to help guide decisions about the appropriateness of implanting a device and the optimal medical strategy during the endocardialization process. If there is controversy about the presence or size of the PFO, a saline contrast transcranial Doppler study of the middle cerebral artery with Valsalva maneuver is sensitive,15 allowing quantification of the amount of right-to-left shunting. The test is not specific to a PFO; pulmonary arteriovenous malformations will also result in a positive test result. Because of a small incidence of atrial arrhythmias after device placement, a baseline electrocardiogram should also be obtained.
Procedural imaging includes fluoroscopy with or without echocardiography, most commonly intracardiac imaging. Unlike for an ASD, the angiogram must be performed in the right atrium to demonstrate the right-to-left shunting, best shown on profile in the lateral plane (75 degrees left anterior oblique and 5 degrees caudal; see Fig. 27-11).
PATENT DUCTUS ARTERIOSUS
Description and Special Anatomic Considerations
Patent ductus arteriosus (PDA) is the persistence of a normal fetal connection between the proximal descending aorta and the proximal left pulmonary artery that allows the right ventricle to bypass the lungs and pump deoxygenated blood through the descending aorta to the placenta for oxygenation. Normal ductal closure occurs within the first 12 hours after birth by contraction and cellular migration of the medial smooth muscle in the wall of the ductus, resulting in protrusion of the thickened intima into the lumen, causing functional closure. Final closure with creation of the ligamentum arteriosum is completed by 3 weeks of age; permanent sealing of the duct by infolding of the endothelium, disruption of the internal elastic lamina, and hemorrhage and necrosis in the subintimal region lead to replacement of muscle fibers with fibrosis. This process of closure is incomplete in 1 in 2000 live births and accounts for up to 10% of all congenital heart disease.16
PDA was one of the first congenital heart lesions treated by interventional techniques, first reported by Porstmann in 1967.17 There have been substantial refinements in devices and techniques during the last 35 years, but for the last 15 years, interventional catheter treatment has been the preferred therapy in most large centers worldwide. It is a particularly attractive technique in adults, in whom surgical ligation and division can be a problem because of calcified ductal tissue and increased surgical risks. The technique is simple, consisting of placement of a device or vascular occlusion coil in the PDA either antegrade from the femoral vein or retrograde from the femoral artery. Once it is implanted, the device physically occludes ductal flow, and during the first 6 to 8 weeks after implantation, endothelial overgrowth covers the device or coil from both the pulmonary artery and aorta, sealing the PDA permanently closed.
Several different closure devices are currently used because of the significant variability of ductal anatomy. The most common anatomic shape is conical with a large aortic ampulla that narrows at the pulmonary artery end; however, other distinct anatomic forms exist, including “tubular” without a narrowing at the pulmonary artery end, “complex” with narrowing at both the aortic and pulmonary ends, and a short “window” that is an anatomy commonly found in adults.18 Different closure tools and techniques may be needed to effectively address these less common PDA anatomic subtypes. The most commonly used technique for closure of PDAs less than 2.5 mm is retrograde placement of embolization coils. For large ducts, antegrade placement of an Amplatzer duct occluder device is the preferred method.
Outcomes and Complications
Transcatheter ductal closure procedural success has been extremely high, with rates of complete closure above 96%.19,20 The procedure takes approximately 2 hours, with discharge within 6 hours. Full activity may resume within 48 hours of the procedure. No anticoagulation or antiplatelet therapy is recommended after the coil closure procedure, although most centers recommend daily aspirin for 4 to 6 months after Amplatzer duct occluder or device closure. Procedural complications are uncommon, occurring in fewer than 5%.19,20 Hemolysis causing anemia may occur if a residual shunt is present after closure with either coils or a device and requires repeated catheterization with placement of additional embolization coils. The major complication associated with coil closure of the PDA is coil embolization to the lungs; however, this is a technical issue that occurs at or immediately after implantation, and the incidence significantly decreases with the operator’s experience. It is related to either undersizing of the coil or malposition on placement. In all but a very few patients, the coils can be snared from their embolized position in the pulmonary artery and removed from the body without sequela. Device embolization, thrombus, and ductal aneurysm have been reported in fewer than 1%.
Imaging Findings
Preoperative Planning
A complete physical examination and thoracic echocardiography are necessary to make the diagnosis before intervention. Transthoracic echocardiography will show an abnormal systolic left-to-right color flow jet into the main pulmonary artery or proximal left pulmonary artery directed inferiorly and anteriorly. The central pulmonary arteries will be dilated, as will the left atrium and left ventricle if the shunt is significant. The ductus itself can be imaged from the left parasternal area. Pulmonary artery pressure estimate can be obtained with the PDA Doppler flow velocity. The hemodynamic significance of the PDA can be assessed by measurement of retrograde flow in the descending aorta (Fig. 27-13). In the adult, in whom echocardiography imaging may be poor, MRI should be performed to demonstrate the PDA anatomy clearly. Unusual variations of the ductus arteriosus include origin from the inferior aspect of the transverse arch and from the proximal innominate or subclavian artery (Fig. 27-14). These are usually associated with more complex forms of congenital heart disease. The anatomy of the ductus can vary significantly; the most common is conical, with the narrowing at the pulmonary artery end (Fig. 27-15). The ductus in the adult tends to be short or window-like (Fig. 27-16) and may have significant calcification. These various ductal anatomies can all be closed interventionally but require a variety of closure devices (Fig. 27-17).
Postoperative Surveillance
The majority of patients can be discharged on the same day or the next morning after the procedure. Predischarge evaluation should include a physical examination to assess for recurrence of ductal murmur (it will have disappeared with successful closure) and sheath insertion site. If a murmur is present or there is concern of device or coil positioning at the time of closure, imaging of the ductal device is necessary, either chest radiography or echocardiography. Uncommon but significant complications associated with ductal closure include proximal left pulmonary artery stenosis and mild coarctation related to device protrusion, both of which can and should be evaluated by echocardiography. If left pulmonary artery stenosis is confirmed on echocardiography, a nuclear medicine pulmonary flow scan should be obtained to evaluate the physiologic significance of the obstruction. After device placement, endothelialization occurs during the first 6 to 12 weeks. Although it is uncommon, development of a small leak next to the coil (Fig. 27-18) or device can occur (<1%), so follow-up echocardiography at 4 to 6 months after closure is critical to ensure complete closure.
COARCTATION OF THE AORTA
Description and Special Anatomic Considerations
Balloon dilation and more recently stent repair are becoming the treatments of choice for coarctation of the aorta in many centers in older children and adults. Coarctation is most often a discrete narrowing of the proximal descending thoracic aorta just distal to the origin of the left subclavian artery at the site of the ductus ligamentum. It represents 7% of all patients with congenital heart disease and results in upper extremity hypertension, left ventricular hypertrophy, and eventually ventricular failure if it is left untreated. Hypoplasia of the distal transverse arch occurs in up to 10% of patients. Although it is much less common, coarctation of the distal thoracic aorta or abdominal aorta does occur, often in association with vasculitis or genetic syndromes such as Williams syndrome. It should be considered during the initial evaluation of systemic hypertension and can easily be diagnosed on physical examination by decreased femoral pulses with a delay compared with radial pulses and blood pressure differential between the arms and legs. A 2/6 systolic ejection murmur can often be heard at the left upper sternal border and over the left side of the back. The narrowing is due to thick intimal and medial ridges that protrude posteriorly and laterally into the aortic lumen.21 Intimal proliferation and elastic lamina disruption occur distal to the ridges because of the high-velocity jet impact on the distal aortic wall. Cystic medial necrosis with disarray and loss of medial elastic tissue occurs commonly in the adjacent aorta and may extend to the ascending aorta as well. It is this abnormality that may lead to late aneurysm formation. The body’s compensatory response to coarctation is the development of vessels that bypass the obstruction, collateral vessels from the innominate, carotid, and subclavian arteries that connect to the thoracic aorta below the level of the coarctation, often connecting through the intercostal arteries. Enlargement of the intercostal arteries due to this collateral flow is the mechanism for rib notching seen on chest radiography in older patients with severe native coarctation.
Contraindications
Patients with coarctation gradients of less than 20 mm Hg with no evidence of collateral flow, hypertension, left ventricular hypertrophy, or abnormal blood pressure response to exercise do not need treatment. Patients with significant hypoplasia and obstruction of the transverse aortic arch in the area of the origin of the carotids should be excluded. Stent repair with jailing of the carotids may be appropriate in the rare patient at extremely high surgical risk; however, for the majority of patients with this lesion, surgical repair should be performed. Any patient with an existing aneurysm should also be cautiously considered. Covered thoracic stents may have a role in this setting, although there are limited data at present. Aortic wall dissection, aneurysm, and rupture associated with stent implantation are uncommon but increase in frequency with the age of the patient and the degree of aortic calcification associated with the coarctation.22 These patients must be considered carefully, and covered stent implantation versus surgery should be considered.
Outcomes and Complications
Balloon dilation for treatment of coarctation was first performed in the early 1980s in children with good success in both native and postoperative recoarctation.23 Its efficacy in adults was found to be similar to that in children24; however, there remained a small but significant failure rate with residual gradient of more than 20 mm Hg in approximately 15% of patients treated. Stent implantation for repair of coarctation was performed sporadically in the early 1990s in children; it was first reported in adults in 1995 with very promising results.25 Since that time, stent repair has become the treatment of choice for coarctation in many centers because of the improved success rate and low restenosis rate, although controlled trials are not available. Procedural success has been reported in more than 95% of patients, with residual obstruction of less than 20 mm Hg. Recurrent stenosis has been extremely rare, occurring in fewer than 5%, usually in younger patients, and is generally mild. Complications have been reported in up to 20% and include aneurysm, perforation, stroke, and death in fewer than 3%.26 In addition, femoral artery complications including arteriovenous fistula and pseudoaneurysm have been reported in association with the larger arterial sheaths required for the procedure.
Imaging Findings
Preoperative Planning
Echocardiography is useful to confirm the diagnosis and to evaluate location and severity of the obstruction. However, spatial resolution is limited, particularly in older adolescents and adults, so to determine exact anatomic dimensions, either thoracic CT or MRI (Fig. 27-19) is needed. MRI gives the added benefit of assessment of the severity of obstruction as well as the significance of collateral flow (Fig. 27-20). Special attention should be paid to the surrounding aortic diameter, including the distal transverse arch, the coarctation diameter, and the location relative to the carotid and left subclavian arteries, as well as to the presence of existing aneurysm, poststenotic dilation, and calcification. Final assessment of the coarctation is performed during the procedure with biplane angiography, including during and after balloon dilation or stent implantation (Fig. 27-21).
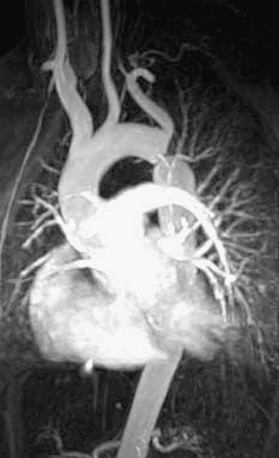
FIGURE 27-19 Three-dimensional reconstruction of MR angiogram in a patient with severe discrete native coarctation of the aorta.
Postoperative Surveillance
Most patients are discharged on the day after the implantation or dilation, and chest radiography should be performed before discharge to ensure stable stent placement. Because late aneurysms may occur in up to 7% as late as 10 years, ongoing imaging surveillance is required. Current recommendations are for CT or MRI scan 6 to 12 months after implantation (Fig. 27-22). If an aneurysm is detected, annual evaluations are required. If no aneurysm is detected, repeated imaging every 4 to 5 years is recommended.
PULMONARY ARTERY STENOSIS
Outcomes and Complications
Balloon dilation of branch pulmonary artery stenosis was initially described in 1983 by Lock and colleagues27; however, only 50% of lesions responded, with a significant restenosis rate. With the availability of larger peripheral stents in the early 1990s and their application to pulmonary artery branch stenosis,28 stent placement has rapidly become the treatment of choice in school-age children and adults because of improved success and low restenosis rates. Shaffer and coworkers29 reported results in more than 130 children and adults with postoperative branch pulmonary artery stenosis; in more than 65%, stent implantation increased lesion diameter by more than 100%, with a median gradient reduction from 46 to 10 mm Hg and right ventricle–to–systemic pressure ratio reduction from 60% to 40%. Long-term results have been excellent, with restenosis rates below 5%. Complications are rare, occurring in fewer than 4% of cases overall, and include hemoptysis, aneurysm, perforation, refractory ventilation-perfusion mismatch, and death. Technical issues, such as device malposition and embolization, have been reported in less than 2% and are quite rare with recent improvements in balloon and stent technology. Recent use of cutting balloons has further improved results to more than 90% success for segmental pulmonary artery stenosis resistant to standard dilation and stenting techniques.30
Imaging Findings
Preoperative Planning
Echocardiography allows accurate assessment of tricuspid regurgitation, right ventricular hypertrophy and dilation, and pulmonary insufficiency before the procedure. It is limited in the evaluation of proximal pulmonary artery stenosis and poor in the evaluation of segmental branch stenosis. MRI, CT, and angiography (Fig. 27-23) remain the best imaging for anatomic stenosis information in the pulmonary arteries. In addition to anatomic information, physiologic information of relative flow to pulmonary segments is crucial before the procedure for interventional decision-making. This should be assessed with either nuclear medicine pulmonary flow scan (Fig. 27-24) or MRA. These flow data are critical and must be coupled with anatomic and pressure data at the procedure to optimize clinical decision-making.
Postoperative Surveillance
Postoperative type and frequency depend on the type and location of treatment (angioplasty alone versus stent implantation) as well as on the presence of vessel injury or aneurysm after treatment. For proximal pulmonary artery lesions treated with balloon angioplasty alone, echocardiography coupled with pulmonary flow scan evaluation at 4 months, then yearly thereafter, is adequate. For more distal stenosis with stent implantation, CT angiography is optimal for imaging, although cardiac MRI (Fig. 27-25) can be used (artifact will depend on stent type—currently both stainless steel and nitinol stents are commonly being used). Nuclear medicine pulmonary flow scan evaluation yearly is needed if flow data cannot be accurately obtained by MRI. If an acute aneurysm develops with the procedure early (Fig. 27-26), more frequent CT or MRI is required during the first year to ensure no progression.
PULMONARY INSUFFICIENCY
Description and Special Anatomic Considerations
Pulmonary valve implantation for pulmonary insufficiency is an active area of development in percutaneous treatment of congenital heart disease. Pulmonary insufficiency with associated right-sided heart dilation and dysfunction is a common occurrence late after repair of tetralogy of Fallot and truncus arteriosus. Although it is tolerated well in childhood, recent information shows that chronic pulmonary insufficiency limits right ventricular function and shortens life span. Surgical repair requires tissue valve implantation, either a homograft or stented autograft. Although quite effective, these valves last only 15 to 20 years, necessitating multiple repeated operations over a lifetime. In 2000, Bonhoeffer and colleagues31 reported the first percutaneous pulmonary valve implantation using a bovine jugular valve sewn inside a balloon-expandable platinum stent. Since that time, more than 450 patients have been treated. Commercial valves are now available in Europe and are currently under investigation in the United States.
Contraindications
Current limitations to percutaneous pulmonary valve implantation are due to both the large sheath size required for the implant and the stented valve maximum diameter (Fig. 27-27). The procedure currently requires a sheath larger than 20F; therefore, most operators restrict use to patients weighing 25 kg or more. Commercially available implants are limited to a maximum diameter of 24 mm, limiting their use in the RVOT or main pulmonary artery to lesions no larger than 22 mm in diameter. This limitation will be relaxed as newer devices are developed to expand to larger diameters. The RVOT must be more than 14 mm in diameter to ensure proper functioning of the valve with expansion. In addition, the location of the coronary arteries relative to the RVOT and main pulmonary artery area is crucial for determination of eligibility. Although uncommon, there are patients after surgical repair of tetralogy of Fallot or truncus arteriosus whose left or right coronary artery courses directly behind the obstructive RVOT. Placement of a stent in these patients will result in coronary compromise and myocardial infarction and can cause death.
Outcomes and Complications
The procedure is successful in 95% of implantations, with significant reduction in right ventricular systolic pressures (63 mm Hg to 45 mm Hg) and of RVOT gradients (37 mm Hg to 17 mm Hg).32 Pulmonary insufficiency, severe in all patients before implantation, was absent in more than 60% and trivial or mild in 38% after 18 months of follow-up. Procedural complications included acute surgery for RVOT and existing homograft dissection in 5%, with no associated mortality. Late complications included endocarditis and reobstruction due to sternal compression or stent fracture. Repeated stent dilation or stent implantation inside the existing stent is needed in up to 12% because of late stenosis or fracture. Freedom from reoperation is 84% at 5 years. Percutaneous valve implantation results in resolution of right ventricular dilation (142 to 91 mL/m2) and improved submaximal exercise tolerance at 1 year.33
Imaging Findings
Preoperative Planning
Preoperative imaging is crucial to determination of eligibility for this procedure as well as for planning. CT or MRI is required to adequately assess the right ventricle, RVOT, main pulmonary artery, and branch pulmonary artery anatomy to determine dimensions. MRI gives the added benefit of pulmonary regurgitant fraction assessment (Fig. 27-28) as well as relative branch pulmonary flows. Specific attention to coronary artery anatomy in the area of the RVOT to assess for large coronary branches immediately posterior to the RVOT is crucial. If coronary artery anatomy is not clear from CT or MRI or there are significant concerns, selective coronary angiography with simultaneous test RVOT balloon expansion is required during the procedure (Fig. 27-29). Angiography in the main pulmonary artery is performed after implantation to assess valve positioning and function (Fig. 27-30). Procedural echocardiography, either transesophageal echocardiography or intracardiac, is useful as well for assessing residual stenosis and insufficiency immediately after implantation to determine the need for additional dilation or stenting.
Postoperative Surveillance
Before discharge, echocardiography and chest radiography are repeated to assess residual stenosis, insufficiency, and stent integrity. Repeated echocardiographic evaluation (Fig. 27-31) is appropriate every 6 months with CT or MRI evaluation annually to assess right ventricle size, in-stent restenosis, stent integrity, and pulmonary regurgitant fraction. If the patient is stable with a competent valve, these assessments can be lengthened to every 2 to 3 years.
KEY POINTS



1 Samánek M. Children with congenital heart disease: probability of natural survival. Pediatr Cardiol. 1992;13:152-158.
2 King TD, Mills NL. Nonoperative closure of atrial septal defects. Surgery. 1974;75:383-388.
3 Suchoń E, Podolec P, Tomkiewicz-Pajak L, et al. Cardiopulmonary exercise capacity in adults with atrial septal defect. Acta Cardiol. 2002;57:75-76.
4 Du ZD, Hijazi ZM, Kleinman CS, et al. Comparison between transcatheter and surgical closure of secundum atrial septal defect in children and adults: results of a multicenter nonrandomized trial. J Am Coll Cardiol. 2002;39:1836-1844.
5 Hughes ML, Maskell G, Goh TH, Wilkinson JL. Prospective comparison of costs and short term health outcomes of surgical versus device closure of atrial septal defect in children. Heart. 2002;88:67-70.
6 Chessa M, Carminati M, Butera G, et al. Early and late complications associated with transcatheter occlusion of secundum atrial septal defect. J Am Coll Cardiol. 2002;39:1061-1065.
7 Lock JE, Cockerham JT, Keane JF, et al. Transcatheter umbrella closure of congenital heart defects. Circulation. 1987;75:593-599.
8 Bridges ND, Hellenbrand W, Latson L, et al. Transcatheter closure of patent foramen ovale after presumed paradoxical embolism. Circulation. 1992;86:1902-1908.
9 Landzberg MJ, Sloss LJ, Faherty CE, et al. Orthodeoxia-platypnea due to intracardiac shunting—relief with transcatheter double umbrella closure. Cathet Cardiovasc Diagn. 1995;36:247-250.
10 Hagen PT, Scholz DG, Edwards WD. Incidence and size of patent foramen ovale during the first 10 decades of life: an autopsy study of 965 normal hearts. Mayo Clin Proc. 1984;59:17-20.
11 Lechat P, Mas JL, Lascault G, et al. Prevalence of patent foramen ovale in patients with stroke. N Engl J Med. 1988;318:1148-1152.
12 Martín F, Sánchez PL, Doherty E, et al. Percutaneous transcatheter closure of patent foramen ovale in patients with paradoxical embolism. Circulation. 2002;106:1121-1126.
13 Sievert H, Horvath K, Zadan E, et al. Patent foramen ovale closure in patients with transient ischemia attack/stroke. J Interv Cardiol. 2001;14:261-266.
14 Beitzke A, Schuchlenz H, Gamillscheg A, et al. Catheter closure of the persistent foramen ovale: mid-term results in 162 patients. J Interv Cardiol. 2001;14:223-229.
15 Telman G, Yalonetsky S, Kouperberg E, et al. Size of PFO and amount of microembolic signals in patients with ischaemic stroke or TIA. Eur J Neurol. 2008;15:969-972.
16 Mitchell SC, Korones SB, Berendes HW. Congenital heart disease in 56,109 births: incidence and natural history. Circulation. 1971;43:323-332.
17 Porstmann W, Wierny L, Warnke H. Closure of persistent ductus arteriosus without thoracotomy. Ger Med Mon. 1967;12:259-261.
18 Krichenko A, Benson LN, Burrows P, et al. Angiographic classification of the isolated, persistently patent ductus arteriosus and implications for percutaneous catheter occlusion. Am J Cardiol. 1989;63:877-880.
19 Wang JK, Liau CS, Huang JJ, et al. Transcatheter closure of patent ductus arteriosus using Gianturco coils in adolescents and adults. Catheter Cardiovasc Interv. 2002;55:513-518.
20 Bilkis AA, Alwi M, Hasri S, et al. The Amplatzer duct occluder: experience in 209 patients. J Am Coll Cardiol. 2001;37:258-261.
21 Edwards JE, Christensen NA, Clagett OT, et al. Pathologic considerations in coarctation of the aorta. Mayo Clin Proc. 1948;23:324-332.
22 Forbes TJ, Garekar S, Amin Z, et al. Procedural results and acute complications in stenting native and recurrent coarctation of the aorta in patients over 4 years of age: a multi-institutional study. Catheter Cardiovasc Interv. 2007;70:276-285.
23 Lock JE, Bass JC, Amplatz K, et al. Balloon dilation angioplasty of aortic coarctations in infants and children. Circulation. 1983;68:109-116.
24 Tynan M, Finley JP, Fontes V, et al. Balloon angioplasty for the treatment of native coarctation: results of Valvuloplasty and Angioplasty of Congenital Anomalies Registry. Am J Cardiol. 1990;65:790-792.
25 Diethrich EB, Heuser RR, Cardenas JR, et al. Endovascular techniques in adult aortic coarctation: the use of stents for native and recurrent coarctation repair. J Endovasc Surg. 1995;2:183-188.
26 Marshall AC, Perry SB, Keane JF, Lock JE. Early results and medium-term follow-up of stent implantation for mild residual or recurrent aortic coarctation. Am Heart J. 2000;139:1054-1060.
27 Lock JE, Castaneda-Zuniga WR, Fuhrman BP, Bass JL. Balloon dilation angioplasty of hypoplastic and stenotic pulmonary arteries. Circulation. 1983;67:962-967.
28 O’Laughlin MP, Perry SB, Lock JE, Mullins CE. Use of endovascular stents in congenital heart disease. Circulation. 1991;83:1923-1939.
29 Shaffer KM, Mullins CE, Grifka RG, et al. Intravascular stents in congenital heart disease: short- and long-term results from a large single-center experience. J Am Coll Cardiol. 1998;31:661-667.
30 Bergersen LJ, Perry SB, Lock JE. Effect of cutting balloon angioplasty on resistant pulmonary artery stenosis. Am J Cardiol. 2003;91:185-189.
31 Bonhoeffer P, Boudjemline Y, Saliba Z, et al. Percutaneous replacement of pulmonary valve in a right-ventricle to pulmonary-artery prosthetic conduit with valve dysfunction. Lancet. 2000;356:1403-1405.
32 Lurz P, Coats L, Khambadkone S, et al. Percutaneous pulmonary valve implantation: impact of evolving technology and learning curve on clinical outcome. Circulation. 2008;117:1964-1972.
33 Frigiola A, Tsang V, Bull C, et al. Biventricular response after pulmonary valve replacement for right ventricular outflow tract dysfunction: is age a predictor of outcome? Circulation. 2008;118(Suppl):S182-S190.