Chapter 4 Complement
• Complement is central to the development of inflammatory reactions and forms one of the major immune defense systems of the body. The complement system serves as one of the links between the innate and adaptive arms of the immune system.
• Complement activation pathways have evolved to label pathogens for elimination. The classical pathway links to the adaptive immune system through antibody. The alternative and lectin pathways provide antibody-independent ‘innate’ immunity, and the alternative pathway is linked to and amplifies the classical pathway.
• The complement system is carefully controlled to protect the body from excessive or inappropriate inflammatory responses. C1 inhibitor controls the classical and lectin pathways. C3 and C5 convertase activity are controlled by decay and enzymatic degradation. Membrane attack is inhibited on host cells by CD59.
• The membrane attack pathway results in the formation of a lytic transmembrane pore. Regulation of the membrane attack pathway by CD59 reduces the risk of ‘bystander’ damage to adjacent host cells.
• Many cells express one or more membrane receptors for complement products. Receptors for fragments of C3 are widely distributed on different leukocyte populations. Receptors for C1q are present on phagocytes, mast cells, and platelets. C5 fragment receptors are present on many cell types. The plasma complement regulator fH binds leukocyte surfaces.
• Complement has a variety of functions. Its principal functions include opsonization, chemotaxis and cell activation, lysis of target cells, and priming of the adaptive immune response.
• Complement deficiencies illustrate the homeostatic roles of complement. Classical pathway deficiencies result in tissue inflammation. Deficiencies of mannan-binding lectin (MBL) are associated with infections in infants and the immunosuppressed. Alternative pathway and C3 deficiencies are associated with bacterial infections. Terminal pathway deficiencies predispose to Gram-negative bacterial infections. C1 inhibitor deficiency leads to hereditary angioedema. Deficiencies in alternative pathway regulators produce a secondary loss of C3.
Complement and inflammation
Complement is now known to comprise some 16 plasma proteins, together constituting nearly 10% of the total serum proteins, and forming one of the major immune defense systems of the body (Fig. 4.1). In addition to serving as a key component of the innate immune system, complement also interfaces with and enhances adaptive immune responses. More than a dozen regulatory proteins are present in plasma and on cells to control complement. The functions of the complement system include:
• triggering and amplification of inflammatory reactions;
• attraction of phagocytes by chemotaxis;
• clearance of immune complexes and apoptotic cells;
• cellular activation for microbial killing;
• direct microbial killing; and
• an important role in the efficient development of antibody responses.
Complement activation pathways
Moreover, there are several different ways to activate the complement system, so providing a large degree of flexibility in response (Fig. 4.2).
All three pathways – classical, alternative, and lectin pathways:
• involve the activation of C3, which is the most abundant and most important of the complement proteins;
• comprise a proteolytic cascade in which complexes of complement proteins create enzymes that cleave other complement proteins in an ordered manner to create new enzymes, thereby amplifying and perpetuating the activation cascade.
Thus a small initial stimulus can rapidly generate a large effect. Figure 4.3 summarizes how each of the pathways is activated.
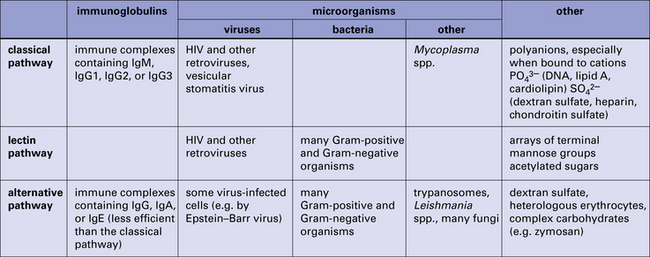
Fig. 4.3 Summary of the activators of the classical, lectin, and alternative pathways
Summary of the activators of the classical, lectin, and alternative complement activation pathways.
• the small chemotactic and proinflammatory fragments C3a and C5a;
The details of complement activation, the nomenclature, and the ways in which the pathways are controlled are shown in Figure 4.4.
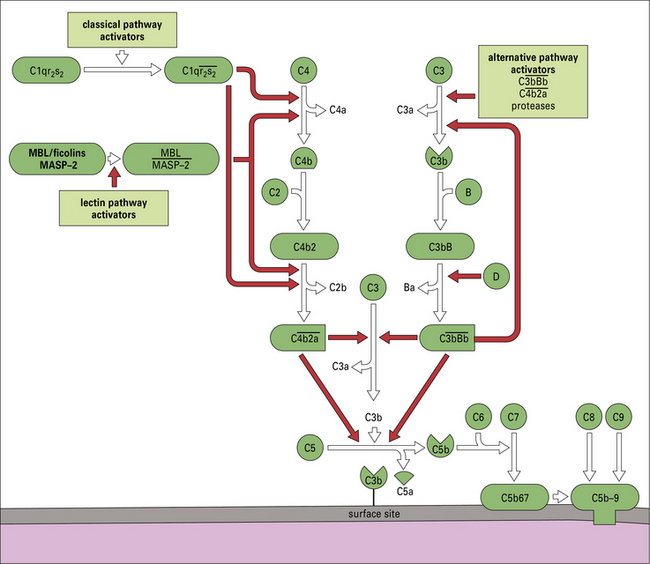
Fig. 4.4 Overview of the complement activation pathways
The proteins of the classical and alternative pathways are assigned numbers (e.g. C1, C2). Many of these are zymogens (i.e. pro-enzymes that require proteolytic cleavage to become active). The cleavage products of complement proteins are distinguished from parent molecules by suffix letters (e.g. C3a, C3b). The proteins of the alternative pathway are called ‘factors’ and are identified by single letters (e.g. factor B, which may be abbreviated to fB or just ‘B’). Components are shown in green, conversion steps as white arrows, and activation/cleavage steps as red arrows. The classical pathway is activated by the cleavage of C1r and C1s following association of C1qr2s2 with classical pathway activators (see Fig. 4.3), including immune complexes. Activated C1s cleaves C4 and C2 to form the classical pathway C3 convertase . Cleavage of C4 and C2 can also be effected via MASP-2 of the lectin pathway, which is associated with mannan-binding lectin (MBL) or ficolin. The alternative pathway is activated by the cleavage of C3 to C3b, which associates with factor B and is cleaved by factor D to generate the alternative pathway C3 convertase
. The initial activation of C3 happens to some extent spontaneously, but this step can also be affected by classical or alternative pathway C3 convertases or a number of other serum or microbial proteases. Note that C3b generated in the alternative pathway can bind more factor B and generate a positive feedback loop to amplify activation on the surface. Note also that the activation pathways are functionally analogous, and the diagram emphasizes these similarities. For example, C3 and C4 are homologous, as are C2 and factor B. MASP-2 is homologous to C1r and C1s. Either the classical or alternative pathway C3 convertases may associate with C3b bound on a cell surface to form C5 convertases,
, or
, which split C5. The larger fragment C5b associates with C6 and C7, which can then bind to plasma membranes. The complex of C5b67 assembles C8 and a number of molecules of C9 to form a membrane attack complex (MAC), C5b–9.
The classical pathway links to the adaptive immune system
The classical pathway is activated by antibody bound to antigen and requires Ca2+
• IgM is the most efficient activator, but unbound IgM in plasma does not activate complement;
• among IgG subclasses, IgG1 and IgG3 are strong complement activators, whereas IgG4 does not activate because it is unable to bind the first component of the classical pathway.
The first component of the pathway, C1, is a complex molecule comprising a large, 6-headed recognition unit termed C1q and two molecules each of C1r and C1s, the enzymatic units of the complex (Fig. 4.5). Assembly of the C1 complex is Ca2+-dependent, and the classical pathway is therefore inactive if Ca2+ ions are absent.
C1s enzyme cleaves C4 and C2
• releasing a small fragment, C4a; and
• exposing a labile thioester group in the large fragment C4b.
Through the highly reactive thioester, C4b becomes covalently linked to the activating surface (Fig. 4.6).
is the classical pathway C3 convertase
• releasing a small fragment, C3a; and
• exposing a labile thioester group in the large fragment C3b.
As described above for C4b, C3b covalently binds the activating surface.
is the classical pathway C5 convertase
• a small fragment, C5a, is released; and
• the large fragment, C5b, remains associated with the complex.
Cleavage of C5 is the final enzymatic step in the classical pathway.
The ability of C4b and C3b to bind surfaces is fundamental to complement function
When either C3 or C4 is cleaved by the convertase enzyme, a conformational change takes place that exposes the internal thioester bond in C3b and C4b, making it very unstable and highly susceptible to attack by nucleophiles such as hydroxyl groups (-OH) and amine groups (-NH2) in membrane proteins and carbohydrates. This reaction creates a covalent bond between the complement fragment and the membrane ligand, locking C3b and C4b onto the surface (see Fig. 4.6).
The alternative and lectin pathways provide antibody-independent ‘innate’ immunity
The lectin pathway is activated by microbial carbohydrates
C1q and MBL are members of the collectin family of proteins characterized by globular head regions with binding activities and long collagenous tail regions with diverse roles (see Fig. 6.w3). Ficolins are structurally similar but the head regions comprise fibrinogen-like domains.
The
complex is the C3 convertase of the alternative pathway
The complex is the C3 cleaving enzyme (C3 convertase) of the alternative pathway. C3b generated by this convertase can be fed back into the pathway to create more C3 convertases, thus forming a positive feedback amplification loop (Fig. 4.7). Activation may occur in plasma or, more efficiently, on surfaces.
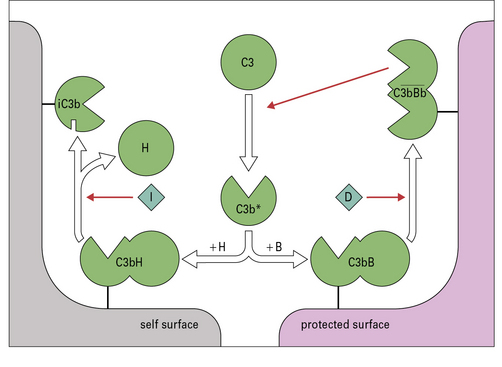
Fig. 4.7 Regulation of the amplification loop
Alternative pathway activation depends on the presence of activator surfaces. C3b bound to an activator surface recruits factor B, which is cleaved by factor D to produce the alternative pathway C3 convertase , which drives the amplification loop, by cleaving more C3. However, on self surfaces the binding of factor H is favored and C3b is inactivated by factor I. Thus the binding of factor B or factor H controls the development of the alternative pathway reactions. In addition, proteins such as membrane cofactor protein (MCP) and decay accelerating factor (DAF) also limit complement activation on self cell membranes (see Fig. 4.9).
On an activating surface such as a bacterial membrane, amplification will occur unimpeded and the surface will rapidly become coated with C3b (see Fig. 4.7). In a manner analogous to that seen in the classical pathway, C3b molecules binding to the C3 convertase will change the substrate specificity of the complex, creating a C5 cleaving enzyme, .
Complement protection systems
C1 inhibitor controls the classical and lectin pathways
In the activation pathways, the regulators target the enzymes that drive amplification:
• activated C1 is controlled by a plasma serine protease inhibitor (serpin) termed C1 inhibitor (C1inh), which removes C1r and C1s from the complex, switching off classical pathway activation;