17 Coagulation Monitoring
CPB itself induces a “whole-body inflammatory response” because of contact of blood and cellular elements with the extracorporeal circuit. The resultant alterations include leukocyte activation, release of inflammatory mediators, free radical formation, complement activation, kallikrein release, platelet activation, and stimulation of the coagulation and fibrinolytic cascades. This complex interplay of systems induces a coagulopathy characterized by microvascular coagulation, platelet dysfunction, and enhanced fibrinolysis.1,2 The homeostatic perturbations incurred during CPB are a major cause for the postbypass coagulopathy that is seen even after the effects of heparin are reversed with protamine.
The need to monitor anticoagulation during and after surgery is the reason that the cardiac surgical arena has evolved into a major site for the evaluation and use of hemostasis monitors. The rapid and accurate identification of abnormal hemostasis has been the major impetus toward the development of point-of-care tests that can be performed at the bedside or in the operating room. The detection and treatment of specific coagulation disorders in a timely and cost-efficient manner are major goals in hemostasis monitoring for the cardiac surgical patient. This chapter discusses the mechanisms of normal coagulation and how they are affected by CPB. The latter portion discusses the laboratory and point-of-care tests available for monitoring the coagulation system. A comprehensive overview of hemostasis, transfusion medicine, and the management of coagulopathy and bleeding disorders after CPB is provided in Chapters 30 and 31.
Hemostasis

Anticoagulation for Cardiopulmonary Bypass
Heparin acts as an antithrombin III (AT III) agonist and accelerates AT III binding to thrombin.3–5 In the absence of AT III, heparin is clinically ineffective as an anticoagulant; adequate AT III activity is necessary in patients about to undergo heparinization for cardiac surgical procedures.6–9
Monitoring heparin effect
The first clotting time to be used to measure heparin’s effect was the whole-blood clotting time (WBCT) or the Lee–White WBCT. This simply requires whole blood to be placed in a glass tube, maintained at 37°C, and manually tilted until blood fluidity is no longer detected. This test fell out of favor for monitoring the cardiac surgical patient because it was so labor intensive and required the undivided attention of the person performing the test for periods up to 30 minutes. Although the glass surface of the test tube acts as an activator of factor XII, the heparin doses used for cardiac surgery prolong the WBCT to such a profound degree that the test is impractical as a monitor of the effect of heparin during cardiac surgery.10 To speed the clotting time so that the test was appropriate for clinical use, activators were added to the test tubes, and the activated coagulation time (ACT) was introduced into practice.11

Activated Coagulation Time
The ACT was first introduced by Hattersley in 1966 and is still the most widely used monitor of heparin effect during cardiac surgery. Whole blood is added to a test tube containing an activator, diatomaceous earth (celite) or kaolin. The presence of activator augments the contact activation phase of coagulation, which stimulates the intrinsic coagulation pathway. ACT can be performed manually, whereby the operator measures the time interval from when blood is injected into the test tube to when clot is seen along the sides of the tube. More commonly, the ACT is automated as it is in the Hemochron and Hemotec systems. In the automated system, the test tube is placed in a device that warms the sample to 37°C. The Hemochron device (International Technidyne, Edison, NJ) rotates the test tube, which contains celite activator and a small iron cylinder, to which 2 mL whole blood is added. Before clot forms, the cylinder rolls along the bottom of the rotating test tube. When clot forms, the cylinder is pulled away from a magnetic detector, interrupts a magnetic field, and signals the end of the clotting time. Normal ACT values range from 80 to 120 seconds. The Hemochron ACT also can be performed using kaolin as the activator in a similar manner (Figure 17-1).
The Hemotec ACT device (Medtronic Hemotec, Parker, CO) is a cartridge with two chambers that contain kaolin activator and is housed in a heat block. Blood (0.4 mL) is placed into each chamber, and a daisy-shaped plunger is raised and passively falls into the chamber. The formation of clot will slow the rate of descent of the plunger, and this decrease in velocity of the plunger is detected by a photo-optical system that signals the end of the ACT test. The Hemochron and Hemotec ACTs have been compared in a number of investigations and have been found to differ significantly at low heparin concentrations.12 However, differences in heparin concentration, activator concentration, and the measurement technique make comparison of these tests difficult and have led to the realization that the Hemochron ACT result and the Hemotec ACT result are not interchangeable. In adult patients given 300 U/kg heparin for CPB, the Hemochron and Hemotec (Hepcon) ACTs were both therapeutic at all time points; however, at two points, the Hemochron ACT was statistically longer13 (Figure 17-2). This difference was even more pronounced in pediatric patients, who have greater heparin consumption rates (Figure 17-3). The apparent “overestimation” of ACT by the Hemochron device during hypothermic CPB may be because of the different volumes of blood that each assay warms to 37°C.
The ACT test can be modified by the addition of heparinase. With this modification, the coagulation status of the patient can be monitored during CPB while the anticoagulant effects of heparin are eliminated. Because this test is a side-by-side comparison of the untreated ACT to the heparinase ACT, it also has the advantage of being a rapid test for the assessment of a circulating heparin-like substance or for residual heparinization after CPB.14
With the introduction of ACT monitoring into the cardiac surgical arena, clinicians have been able to more accurately titrate heparin and protamine dosages.11,15 As a result, many investigators report reductions in blood loss and transfusion requirements, although many of these studies used retrospective analyses.16 The improvements in postoperative hemostasis documented with ACT monitoring are potentially attributable to better intraoperative suppression of microvascular coagulation and improved monitoring of heparin reversal with protamine.17
ACT monitoring of heparinization is not without pitfalls, and its use has been criticized because of the extreme variability of the ACT and the absence of a correlation with plasma heparin levels (Figure 17-4). Many factors have been suggested to alter the ACT, and these factors are prevalent during cardiac surgical procedures. When the extracorporeal circuit prime is added to the patient’s blood volume, hemodilution occurs and may theoretically increase ACT. Evidence suggests that this degree of hemodilution alone is not enough to actually alter ACT. Hypothermia increases ACT in a “dose-related” fashion. It has been shown by Culliford et al18 that, although hemodilution and hypothermia significantly increase the ACT of a heparinized blood sample, similar increases do not occur in the absence of added heparin. The effects of platelet alterations are a bit more problematic. At mild-to-moderate degrees of thrombocytopenia, the baseline and heparinized ACT are not affected. It is not until platelet counts are reduced to less than 30,000 to 50,000/μL that ACT may be prolonged.19 Patients treated with platelet inhibitors such as prostacyclin, aspirin, or platelet-membrane-receptor antagonists have a prolonged heparinized ACT compared with patients not treated with platelet inhibitors.20 This ACT prolongation is not related exclusively to decreased levels of platelet factor 4 (PF4) (PF4 is a heparin-neutralizing substance) because it also occurs when blood is anticoagulated with substances that are not neutralized by PF4. Platelet lysis, however, significantly shortens the ACT because of the release of PF4 and other platelet membrane components, which may have heparin-neutralizing activities.21 Gravlee et al22 showed that anesthesia and surgery decrease the ACT and create a hypercoagulable state, possibly by creating a thromboplastic response or through activation of platelets.
During CPB, heparin decay varies substantially and its measurement is problematic because hemodilution and hypothermia alter the metabolism of heparin. In a CPB study, Mabry et al23 found that the consumption of heparin varied from 0.01 to 3.86 U/kg/min and there was no correlation between the initial sensitivity to heparin and the rate of heparin decay.23 In the pediatric population, the consumption of heparin is increased to more than that of adult levels. The heparin administration protocol for pediatric patients undergoing CPB should account for a large volume of distribution, increased consumption, and a shorter elimination half-life. In monitoring the effects of heparin in pediatric patients, the minimum acceptable ACT value should be increased or an additional monitor should be used. The discrepancy between the Hemochron ACT and the Hemotec ACT that is demonstrated in Figure 17-2 is even more pronounced in pediatric patients (see Figure 17-3). Some investigators recommend the maintenance of heparin concentrations in addition to ACT during pediatric congenital heart surgery to ensure that optimal anticoagulation is being achieved.24,25

Cascade POC (Point of Care) System
A completely different technology for measuring the effect of heparin is used by the Cascade POC analyzer (Helena, Beaumont, TX; Formerly Rapid Point Coagulation Analyzer Bayer Diagnostics, Tarrytown, NY). This test system contains disposable cards with celite activator for the measurement of heparin activity. This variation of the ACT is called the “heparin management test” (HMT). This card contains paramagnetic iron oxide particles that move in response to an oscillating magnetic field within the device. When clot formation occurs, movement of the iron oxide particles is decreased and the end of the test is signaled. This system is capable of measuring prothrombin time (PT) and activated partial thromboplastin time (aPTT), which is discussed later. The suitability of this platform for the monitoring of ACT during cardiac surgery has been demonstrated in a variety of clinical studies.26,27 Suitability for monitoring heparinization in the interventional cardiology laboratory also has been reported. HMT correlates well with anti-Xa heparin activity in CPB patients and is less variable than standard ACT measures. In a comparison with ACT, the coefficients of variation were similar between the tests at baseline but were three times greater for the ACT during heparinization. This degree of agreement with plasma anti-Xa measurements has not been demonstrated universally when studying blood from patients undergoing CPB.28

Heparin Resistance
Heparin resistance is documented by an inability to increase the ACT of blood to expected levels despite an adequate dose and plasma concentration of heparin. In many clinical situations, especially when heparin desensitization or a heparin inhibitor is suspected, heparin resistance can be treated by administering increased doses of heparin in a competitive fashion. If an adequately prolonged clotting time is ultimately achieved using greater than expected doses of heparin, a better term than heparin resistance would be heparin tachyphylaxis of “altered heparin responsiveness.” During cardiac surgical procedures, the belief that a safe minimum ACT value of 300 to 400 seconds is required for CPB is based on a few clinical studies and a relative paucity of scientific data. However, inability to attain this degree of anticoagulation in the heparin-resistant patient engenders the fear among cardiac surgical providers that the patient will experience a microvascular consumptive coagulopathy or that clots will form in the extracorporeal circuit. This potential for fibrin formation in the extracorporeal circuit was reported by Young et al,29 who found increased production of fibrin monomer and consumption of fibrinogen and platelets in six of nine rhesus monkeys when the ACT declined to less than 400 seconds. However, Metz and Keats30 reported no adverse effects of thrombosis or excessive bleeding in 51 patients undergoing CPB whose ACT was less than 400 seconds. In a porcine model, the group whose ACT was maintained between 250 and 300 seconds did not have excessive consumption of coagulation factors, increases in fibrin monomer formation, or changes in oxygenator performance compared with the group whose ACT was maintained at greater than 450 seconds.31
Many clinical conditions are associated with heparin resistance.32 Sepsis, liver disease, and pharmacologic agents represent just a few33,34 (Table 17-1). Many investigators have documented decreased levels of AT III secondary to heparin pretreatment,35 whereas others have not found decreased AT III levels.36 Esposito et al37 measured coagulation factor levels in patients receiving preoperative heparin infusions and found that a lower baseline ACT was the only risk factor for predicting heparin resistance compared with patients not receiving preoperative heparin.
TABLE 17-1 Disease States Associated with Heparin Resistance
Disease State | Comment |
---|---|
Newborn | Decreased AT III levels until 6 months of age |
Venous thromboembolism | May have increased factor VIII level |
Accelerated clearance of heparin | |
Pulmonary embolism | Accelerated clearance of heparin |
Congenital AT III deficiency | 40% to 60% of normal AT III concentration |
Type I | Reduced synthesis of normal/abnormal AT III |
Type II | Molecular defect within the AT III molecule |
Acquired AT III deficiency | < 25% of normal AT III concentration |
Preeclampsia | Levels unchanged in normal pregnancy |
Cirrhosis | Decreased protein synthesis |
Nephrotic syndrome | Increased urinary excretion of AT III |
DIC | Increased consumption of AT III |
Heparin pretreatment | 85% of normal AT III concentration because of accelerated clearance |
Estrogen therapy | |
Cytotoxic drug therapy (l-asparaginase) | Decreased protein synthesis |
ATIII, antithrombin III; DIC, disseminated intravascular coagulation.
Patients receiving preoperative heparin therapy traditionally require larger heparin doses to achieve a given level of anticoagulation when that anticoagulation is measured by the ACT. Presumably, this “heparin resistance” is due to deficiencies in the level or activity of AT III.34,37–40 Other possible causes include enhanced factor VIII activity and platelet dysfunction causing a decrease in ACT response to heparin. Levy et al40 have shown that the in vitro addition of AT III enhances the ACT response to heparin. Lemmer and Despotis39 demonstrated that this heparin resistance, as measured by the ACT, does not correlate with preoperative AT III levels. It is unclear that these patients have increased heparin requirements during CPB because the ideal ACT and monitoring techniques have yet to be elucidated. Nicholson et al41 demonstrated that the temporal courses of ACT and AT III concentration do not parallel each other, further suggesting that AT III depletion is not the sole cause of heparin resistance during CPB. Lower ACTs (lower than 480s) were tolerated in this study with no adverse outcome. AT III concentrate is available as a heat-treated human product or in recombinant form and represents a reasonable method of treating patients with documented AT III deficiency (Box 17-1). Heparin responsiveness in the form of increased ACT levels is documented both in vitro and in vivo when heparin-resistant patients are treated with AT III.
BOX 17-1. Heparin Resistance
In a multicenter, randomized, placebo-controlled trial using 75 U/kg recombinant AT III, AT III–treated patients received less FFP to augment ACT levels and also had evidence of less hemostatic activation while on CPB.42 There was a trend toward increased blood loss in the AT III group, which is potentially a dose effect that requires further investigation.43,44

Heparin-Induced Thrombocytopenia
The options for treating these patients are few. If the clinician has the luxury of being able to discontinue heparin for a few weeks, often the antibody disappears and allows a brief period of heparinization for CPB without complication.45,46 Changing the tissue source of heparin was an option when bovine heparin was predominantly in use. Some types of low-molecular-weight heparin have been administered to patients with HIT, but reactivity of the particular low-molecular-weight heparin with the patient’s platelets should be confirmed in vitro. Supplementing heparin administration with pharmacologic platelet inhibition using prostacyclin, iloprost, aspirin, or aspirin and dipyridamole have been reported, all with favorable outcomes. The use of tirofiban with unfractionated heparin has been used in this clinical circumstance. Plasmapheresis may be used to reduce antibody levels. The use of heparin could be avoided altogether through anticoagulation with direct thrombin inhibitors such as argatroban, hirudin, or bivalirudin. These thrombin inhibitors have become standard of care in the management of the patient with HIT (Box 17-2). Bivalirudin has been studied in multicenter trials in HIT patients who must undergo CPB.47 Monitoring the direct thrombin inhibitors during CPB is discussed later in this chapter.

Measurement of Heparin Sensitivity
Even in the absence of heparin resistance, patient response to an intravenous bolus of heparin is extremely variable.48 The variability stems from different concentrations of various endogenous heparin-binding proteins such as vitronectin and PF4. This variability exists whether measuring heparin concentration or the ACT; however, variability seems to be greater when measuring the ACT. Because of the large interpatient variation in heparin responsiveness and the potential for heparin resistance, it is critical that a functional monitor of heparin anticoagulation (with or without a measure of heparin concentration) be used in the cardiac surgical patient. Bull et al49 documented a threefold range of ACT response to a 200-U/kg heparin dose and similar discrepancy in heparin decay rates and, thus, recommended the use of individual patient dose–response curves to determine the optimal heparin dose. This is the concept on which point-of-care individual heparin dose–response (HDR) tests are based.
An HDR curve can be generated manually using the baseline ACT and the ACT response to an in vivo or in vitro dose of heparin. Extrapolation to the desired ACT provides the additional heparin dose required for that ACT. Once the actual ACT response to the heparin dose is plotted, further dose–response calculations are made based on the average of the target ACT and the actual ACT (Figure 17-5). This methodology was first described by Bull et al49 and forms the scientific basis for the automated dose–response systems manufactured by Hemochron and Hemotec. The Hemochron RxDx system uses the heparin-response test, which is an ACT with a known quantity of in vitro heparin (3 IU/mL). A dose–response curve is generated that enables calculation of the heparin dose required to attain the target ACT using an algorithm that incorporates the patient’s baseline ACT, estimated blood volume, and heparin-response test. The patient’s heparin sensitivity can be calculated in seconds per international units per milliliter (sec/IU/mL) by dividing the heparin-response test by 3 IU/mL.
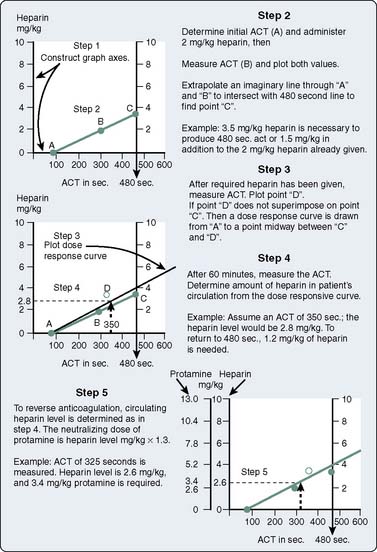
Figure 17-5 Construction of a dose–response curve for heparin. ACT, activated coagulation time.
(From Bull BS, Huse WM, Brauer FS, et al: Heparin therapy during extracorporeal circulation: II. The use of a dose-response curve to individualize heparin and protamine dosage. J Thorac Cardiovasc Surg 69:685–689, 1975.)
The RxDx system also provides an individualized protamine dose using the protamine-response test (PRT). This is an ACT with one of two specific quantities of protamine, depending on the amount of circulating heparin suspected (2 or 3 IU/mL). The protamine dose needed to return the ACT to baseline can be calculated on the basis of a protamine–response curve using the patient’s heparinized ACT, the PRT, and an estimate of the patient’s blood volume. Jobes et al50 reported that the heparin dose directed by the RxDx system resulted in ACT values far greater than the target ACT. In their patients, in vivo heparin sensitivity was higher than in vitro sensitivity. RxDx also resulted in lower protamine doses, lower postoperative mediastinal tube losses, and reduced transfusion requirements compared with a ratio-based system of heparin/protamine administration. In a larger study that standardized the treatment of heparin rebound, the reduced protamine dose was confirmed; however, the reductions in bleeding were not substantiated.51 The use of a protamine dose–response curve has been shown to successfully reduce the protamine dose in vascular surgery compared with standard weight-based protamine dosing.52
The Hepcon HMS system uses the HDR cartridge in the Hepcon instrument (Figure 17-6). Each cartridge houses six chambers. Chambers 1 and 2 contain heparin at a concentration of 2.5 IU/mL, chambers 3 and 4 contain heparin at a concentration of 1.5 IU/mL, and chambers 5 and 6 do not contain heparin. Once information regarding patient weight, height, and CPB prime volume is entered, the information that can be obtained from this test includes the baseline ACT (chambers 5 and 6) and an HDR slope. The dose–response slope, which is the increase in ACT from 1.5 to 2.5 IU/mL heparin, is extrapolated to the desired target ACT or target heparin concentration and the heparin dose is calculated.32,53

Heparin Concentration
Proponents of ACT measurement to guide anticoagulation for CPB argue that a functional assessment of the anticoagulant effect of heparin is mandatory and that the variability in ACT represents a true variability in the coagulation status of the patient. Opponents argue that during CPB, the sensitivity of the ACT to heparin is altered and ACT does not correlate with heparin concentration or with anti–factor Xa activity measurement. Heparin concentration can be measured using the Hepcon HMS system (Medtronic Hemotec), which uses an automated protamine titration technique. With a cartridge with four or six chambers containing tissue thromboplastin and a series of known protamine concentrations, 0.2 mL whole blood is automatically dispensed into the chambers. The first channel to clot is the channel whose protamine concentration most accurately neutralizes the heparin without a heparin or a protamine excess. Because protamine neutralizes heparin in the ratio of 1 mg protamine per 100 units heparin, the concentration of heparin in the blood sample can be calculated. A cartridge that monitors heparin concentration over a wide range can be used first, followed by another cartridge that can measure heparin concentrations within a more narrow range. The maintenance of a stable heparin concentration rather than a specific ACT level usually results in greater doses of heparin being administered because the hemodilution and hypothermia on CPB increase the sensitivity of the ACT to heparin. The measure of heparin concentration has been shown to correlate more closely with anti–factor Xa activity measurements than the ACT during CPB54 (Figure 17-7), although the precision and bias of the test may not prove to be acceptable for exclusive use clinically (Figure 17-8).
In a small, prospective, randomized study comparing ACT and heparin concentration monitoring, Gravlee et al55 demonstrated increased mediastinal tube drainage after surgery in the heparin concentration group, a finding that was initially attributed to greater total heparin doses. However, heparin rebound was not systematically assessed. In a follow-up study, the authors found a greater incidence of heparin rebound in the heparin concentration group, which, when treated, resulted in no difference in bleeding between the ACT and heparin concentration groups.56 In a prospective, randomized trial, Despotis et al57 demonstrated that by using a transfusion algorithm in association with Hepcon-based heparin management, chest tube drainage was minimally reduced and transfusion of non–red blood cell products could be significantly reduced relative to a group of patients who had ACT-based heparin management. They attributed their results to better preservation of the coagulation system by high heparin doses because the doses of heparin administered in the Hepcon group were nearly twice the doses used in the ACT management group. However, Gravlee et al55 were unable to confirm suppression of ongoing coagulation using Hepcon CPB management. With the exception of during cold CPB, fibrinopeptide A (FPA) levels in patients who had heparin concentration monitoring were virtually indistinguishable from those in patients who had ACT monitoring (Figure 17-9). The Hepcon, however, remains one of the more sensitive tests for detecting residual heparinization after protamine reversal because the heparin concentration can be measured by protamine titration to levels as low as 0.4 IU/mL (see Monitoring for Heparin Rebound section later in this chapter).

High-Dose Thrombin Time
A functional test of heparin-induced anticoagulation that correlates well with heparin levels is the high-dose thrombin time (HiTT; International Technidyne, Edison, NJ). The TT is a clotting time that measures the conversion of fibrinogen to fibrin by thrombin. The TT is prolonged by the presence of heparin and by hypofibrinogenemias or dysfibrinogenemias. Because the TT is sensitive to very low levels of heparin, a high dose of thrombin is necessary in the TT to accurately assay the high doses of heparin used for CPB. The HiTT is performed by adding whole blood to a prewarmed, prehydrated test tube that contains a lyophilized thrombin preparation. After the addition of 1.5 mL blood, the tube is inserted into a Hemochron well and the time to clot formation is measured. In vitro assays indicate that HiTT is equivalent to the ACT in evaluation of the anticoagulant effects of heparin at heparin concentrations in the range of 0 to 4.8 IU/mL (Figure 17-10). Unlike ACT, HiTT is not altered by hemodilution and hypothermia, and has been shown to correlate better with heparin concentration than the ACT during CPB.58 While on CPB, heparin concentration and HiTT decrease, whereas the Hemochron and the Hepcon ACT increase (Figure 17-11). Another potential advantage of HiTT monitoring is for patients receiving aprotinin therapy. In the presence of heparin, aprotinin augments the celite ACT,59 possibly because its kallikrein-inhibiting capacity prolongs activation of the intrinsic coagulation pathway by XIIa. This should not be interpreted to represent enhanced anticoagulation. The kaolin ACT is less affected by aprotinin therapy than the celite ACT, perhaps because kaolin, unlike celite, activates the intrinsic pathway by stimulation of factor XI directly.60 Others have suggested that kaolin binds to aprotinin and reduces the anticoagulant effect of aprotinin in vitro. However, the heparinized kaolin ACT is still somewhat prolonged in the presence of aprotinin. HiTT is not affected by aprotinin therapy and can be used as a measure of heparinization for CPB patients receiving aprotinin therapy.59 The high-dose thromboplastin time is another measure of anticoagulation that is not affected by aprotinin therapy.61 The high-dose thromboplastin time is a WBCT in which celite is replaced by 0.3 mL of rabbit brain thromboplastin to which 1.2 mL blood is added. This test measures the time to coagulation via activation of the extrinsic pathway. This pathway of coagulation is also stimulated during pericardiotomy because of the rich thromboplastin environment of the pericardial cavity.
Heparin neutralization

Protamine Effects on Coagulation Monitoring
Reversal of heparin-induced anticoagulation is most frequently performed with protamine. Biologically, protamine binds to positively charged groups such as phosphate groups and may have important properties in angiogenesis and immune function. Different successful dosing plans have been proposed.62,63 The recommended dose of protamine for heparin reversal is 1 to 1.3 mg protamine per 100 units heparin; however, this dose often results in a protamine excess.
Protamine injection causes adverse hemodynamic effects.64 Protamine reactions have been classified into three types.65 The most common of these reactions is the type I reaction characterized by hypotension. Type II (immunologic) reactions are categorized as IIA (anaphylaxis), IIB (anaphylactoid), and IIC (noncardiogenic pulmonary edema). Type III reactions are heralded by hypotension and catastrophic pulmonary hypertension leading to right-heart failure.33,52,66
In addition to hemodynamic sequelae, protamine has adverse effects on coagulation.67 Large doses prolong the WBCT and the ACT, possibly via thrombin inhibition.68 In animals and in humans, protamine has been associated with thrombocytopenia, likely because of activation of the complement cascade.64 The anticoagulant effect of protamine also may be caused by inhibition of platelet aggregation, alteration in the platelet surface membrane, or depression of the platelet response to various agonists.68–70 These alterations in platelet function result from the presence of the heparin–protamine complex, not protamine alone. Protamine–heparin complexes activate AT III in vitro and result in complement activation. The anticoagulant effects of free protamine occur when protamine is given in doses in excess of those used clinically; however, the risk of free protamine being the cause of a hemostatic defect is small, given the rapid clearance of protamine relative to heparin.

Monitoring for Heparin Rebound
The phenomenon referred to as heparin rebound describes the re-establishment of a heparinized state after heparin has been neutralized with protamine. Various explanations for heparin rebound have been proposed.9,56,71,72 The most commonly postulated is that rapid distribution and clearance of protamine occur shortly after protamine administration, leaving unbound heparin remaining after protamine clearance. Furthermore, endogenous heparin antagonists have an even shorter life span than protamine and are eliminated rapidly, resulting in free heparin concentrations. Also possible is the release of heparin from tissues considered heparin storage sites (endothelium, connective tissues). Endothelial cells bind and depolymerize heparin via PF4. Uptake into the cells of the reticuloendothelial system, vascular smooth muscle, and extracellular fluid may account for the storage of heparin that contributes to reactivation of heparin anticoagulation, referred to as heparin rebound.9
Residual low levels of heparin can be detected by sensitive heparin concentration monitoring in the first hour after protamine reversal and can be present for up to 6 hours after surgery. Gravlee et al’s56 study suggests that without careful monitoring for heparin rebound in the postoperative period, increased bleeding as a result of heparin rebound may occur, specifically when greater doses of heparin have been administered. Monitoring for heparin rebound can be accomplished using tests that are sensitive to low levels of circulating heparin.52,57,73,74 These tests are also useful monitors for confirmation of heparin neutralization at the conclusion of CPB (see later).

Heparin Neutralization Monitors
To administer the appropriate dose of protamine at the conclusion of CPB, it would be ideal to measure the concentration of heparin present and give the dose of protamine necessary to neutralize only the circulating heparin. As a result of heparin metabolism and elimination, which vary considerably among individuals, the dose of protamine required to reverse a given dose of heparin decreases over time. Furthermore, protamine antagonizes the anti-IIa effects of heparin more effectively than the anti-Xa effects and, thus, varies in its potency depending on the source of heparin and its anti-IIa properties. Administration of a large fixed dose of protamine or a dose based on the total heparin dose given is no longer the standard of care and may result in an increased incidence of protamine-related adverse effects. An optimal dose of protamine is desired because unneutralized heparin results in clinical bleeding and an excess of protamine may produce an undesired coagulopathy. The use of individualized protamine dose–response curves uniformly results in a reduced protamine dose and has been shown to reduce postoperative bleeding.49,73 One such dose–response test, the Hemochron PRT test, is an ACT performed on a heparinized blood sample that contains a known quantity of protamine. With knowledge of the ACT, PRT, and the estimated blood volume of the patient, the protamine dose needed to neutralize the existing heparin level can be extrapolated. The Hepcon instrument also has a PRT, which is the protamine titration assay. The chamber that clots first contains the dose of protamine that most closely approximates the circulating dose of heparin. The protamine dose required for its neutralization is calculated on the basis of a specified heparin/protamine dose ratio by measuring the circulating heparin level.
At the levels of heparinization needed for cardiac surgery, tests that are sensitive to heparin become unclottable. ACT is relatively insensitive to heparin and is ideal for monitoring anticoagulation at high heparin levels but is too insensitive to accurately diagnose incomplete heparin neutralization. Reiner et al75 showed that ACT had a high predictive value for adequate anticoagulation (confirmed by laboratory aPTT) when longer than 225 seconds but was poorly predictive for inadequate anticoagulation when shorter than 225 seconds. The low levels of heparin present when heparin is incompletely neutralized are best measured by other more sensitive tests of heparin-induced anticoagulation, such as heparin concentration, aPTT, and TT. Thus, after CPB, confirmation of return to the unanticoagulated state should be performed with a sensitive test for heparin anticoagulation76–79 (Box 17-3).
Thrombin Time
TT is the time it takes for the conversion of fibrinogen to fibrin clot when blood or plasma is exposed to thrombin. Fibrin strands form in seconds. Detection of fibrin formation using standard laboratory equipment involves incubation of the blood or plasma sample within the chamber in which an optical or electrical probe sits. A detector senses either movement of the probe or the creation of an electrical field (electrical detection) because of fibrin formation and hence signals the end of the test. Hemochron manufactures a point-of-care TT test that uses a lyophilized preparation of thrombin in a Hemochron test tube to which 1 mL blood is added. Identification of fibrin formation in a Hemochron machine uses the standard Hemochron technology described previously with the ACT. The manufacturer suggests that the normal TT is 39 to 53 seconds for whole blood and 43 to 68 seconds for citrated blood. Because the TT specifically measures the activity of thrombin, it is very sensitive to heparin-induced enhancement of AT III activity. It is a useful test in the post-CPB period for differentiating the cause of bleeding when both PT and aPTT are prolonged because it excludes the intrinsic and extrinsic coagulation pathway limbs and evaluates the conversion of factor I to Ia. The TT is increased in the presence of heparin, hypofibrinogenemia, dysfibrinogenemia, amyloidosis, or antibodies to thrombin.80 The TT is also increased in the presence of fibrin degradation products if the systemic fibrinogen concentration is low.
Bedside Tests of Heparin Neutralization
Hepcon measures heparin concentration via a protamine titration assay. Cartridges with varying ranges of protamine concentration are available for use. The cartridge with the lower concentration of protamine in the titration is useful for the detection of residual circulating heparin and is sensitive to levels of heparin as low as 0.2 IU/mL. Whole-blood PT and aPTT assays are sensitive to deficiencies in coagulation factors and overly sensitive to low levels of heparin (aPTT); they lack specificity in assessing residual heparinization. The heparin-neutralized thrombin time (HNTT) is a TT assay with a small dose of protamine sufficient to neutralize 1.5 IU/mL heparin. Because the TT is increased in the presence of heparin, hypofibrinogenemia, or dysfibrinogenemia, HNTT and TT should be performed together to discriminate among these three causes. A normal HNTT in the presence of an increased TT virtually confirms residual heparin effect and would indicate the need for protamine administration. If HNTT is prolonged as well as the TT, the cause of bleeding may be attributed either to a fibrinogen problem or to a concentration of heparin greater than that which could be neutralized by the HNTT. In one study comparing bedside monitors of anticoagulation, the TT-HNTT difference bore a significant correlation with the aPTT increase. Using the line of best fit, aPTT elevation of 1.5 times the control corresponded to a 31-second difference in the TT and HNTT, indicating a convenient threshold value of TT-HNTT for the administration of protamine.80

Platelet Factor 4
A component of the alpha granule of platelets, PF4 binds to and inactivates heparin. The physiologic role of PF4 is that at the site of vascular injury, PF4 is released from platelets, binds heparin (or heparin-like compounds), and promotes thrombin and clot formation. PF4 adequately neutralizes heparin inhibition of factors Xa and IIa and may be superior to protamine in neutralizing anti-Xa effects. Animal data suggest that PF4 is devoid of the adverse hemodynamic effects seen with protamine and that it may be able to be infused more rapidly.81,82 Heparin reversal has been documented by WBCT, ACT, and heparin concentration at a PF4 concentration of 40 U/mL, approximately twice the reversal dose of protamine.74 Levy et al83 subsequently found the reversal dose of PF4 to be approximately 60 U/mL and documented similar ACT and viscoelastic measurements of clot formation compared with protamine.

Heparinase
Heparinase (Neutralase I) is an enzyme that specifically degrades heparin by catalyzing cleavage of the saccharide bonds found in the heparin molecule. As demonstrated by the ACT, heparinase in a dose of 5 mg/kg has been shown to successfully neutralize heparin effects in healthy volunteers and in patients who have undergone CPB. A dose of 7 mg/kg has been demonstrated to be even more efficacious in returning ACT to baseline values. Doses sufficient to neutralize a dose of 300 IU/kg of heparin had no significant hemodynamic effects in a canine model.84 Investigators have not found any platelet-depressive effects of heparinase in contrast with the well-documented platelet dysfunction associated with protamine therapy.69 Return to the unanticoagulated state after the use of heparinase has been confirmed using ACT monitoring or heparin concentration monitoring.