Chapter 12 Coagulation Monitoring
MONITORING HEPARIN EFFECT
Activated Coagulation Time
The ACT was first introduced by Hattersley in 1966 and is still the most widely used monitor of heparin effect during cardiac surgery. Whole blood is added to a test tube containing an activator—diatomaceous earth (celite) or kaolin. The presence of activator augments the contact activation phase of coagulation, which stimulates the intrinsic coagulation pathway. ACT can be performed manually, whereby the operator measures the time interval from when blood is injected into the test tube to when clot is seen along the sides of the tube. More commonly, the ACT is automated as it is in the Hemochron and Hemotec systems. In the automated system, the test tube is placed in a device that warms the sample to 37°C. The Hemochron device (International Technidyne Corp., Edison, NJ) rotates the test tube, which contains celite activator and a small iron cylinder, to which 2 mL of whole blood is added. Before clot forms, the cylinder rolls along the bottom of the rotating test tube. When clot forms, the cylinder is pulled away from a magnetic detector, interrupts a magnetic field, and signals the end of the clotting time. Normal ACT values range from 80 to 120 seconds. The Hemochron ACT can also be performed using kaolin as the activator in a similar manner (Fig. 12-1).
The Hemotec ACT device (Medtronic Hemotec, Parker, CO) is a cartridge with two chambers that contain kaolin activator and is housed in a heat block. Blood (0.4 mL) is placed into each chamber and a daisy-shaped plunger is raised and passively falls into the chamber. The formation of clot will slow the rate of descent of the plunger, and this decrease in velocity of the plunger is detected by a photo-optical system that signals the end of the ACT test. The Hemochron and Hemotec ACTs have been compared in a number of investigations and have been found to differ significantly at low heparin concentrations.1 However, differences in heparin concentration, activator concentration, and the measurement technique make comparison of these tests difficult and have led to the realization that the Hemochron ACT result and the Hemotec ACT result are not interchangeable. In adult patients given 300 U/kg of heparin for cardiopulmonary bypass (CPB), the Hemochron and Hemotec (Hepcon) ACTs were both therapeutic at all time points; however, at two points, the Hemochron ACT was statistically longer. This difference was even more pronounced in pediatric patients, who have higher heparin consumption rates. The apparent “overestimation” of ACT by the Hemochron device during hypothermic CPB may be due to the different volumes of blood that each assay warms to 37°C.
ACT monitoring of heparinization is not without pitfalls, and its use has been criticized because of the extreme variability of the ACT and the absence of a correlation with plasma heparin levels (Fig. 12-2). Many factors have been suggested to alter the ACT, and these factors are prevalent during cardiac surgical procedures. When the extracorporeal circuit prime is added to the patient’s blood volume, hemodilution occurs and may theoretically increase ACT. Evidence suggests that this degree of hemodilution alone is not enough to actually alter ACT. Hypothermia increases ACT in a “dose-related” fashion. It has been shown that although hemodilution and hypothermia significantly increase the ACT of a heparinized blood sample, similar increases do not occur in the absence of added heparin. The effects of platelet alterations are a bit more problematic. At mild to moderate degrees of thrombocytopenia, the baseline and heparinized ACT are not affected. It is not until platelet counts are reduced to below 30,000 to 50,000/μL that ACT may be prolonged. Patients treated with platelet inhibitors such as prostacyclin, aspirin, or platelet membrane receptor antagonists have a prolonged heparinized ACT compared with patients not treated with platelet inhibitors. This ACT prolongation is not exclusively related to decreased levels of platelet factor 4 (PF4) (PF4 is a heparin-neutralizing substance), because it also occurs when blood is anticoagulated with substances that are not neutralized by PF4. Platelet lysis, however, significantly shortens the ACT due to the release of PF4, and other platelet membrane components, which may have heparin-neutralizing activities. Anesthesia and surgery also decrease the ACT and create a hypercoagulable state, possibly by creating a thromboplastic response or through activation of platelets.
Heparin Resistance
Many clinical conditions are associated with heparin resistance. Sepsis, liver disease, and pharmacologic agents represent just a few (Table 12-1). Many investigators have documented decreased levels of antithrombin III (ATIII) secondary to heparin pretreatment, whereas others have not found decreased ATIII levels.2 In patients receiving preoperative heparin infusions, lower baseline ACT was the only risk factor found for predicting heparin resistance compared with patients not receiving preoperative heparin.
Table 12-1 Disease States Associated with Heparin Resistance
Disease State | Comment |
---|---|
Newborn | Decreased ATIII levels until 6 months of age |
Venous thromboembolism | May have increased factor VIII level |
Accelerated clearance of heparin | |
Pulmonary embolism | Accelerated clearance of heparin |
Congenital ATIII deficiency | 40% to 60% of normal ATIII concentration |
Type I | Reduced synthesis of normal/abnormal ATIII |
Type II | Molecular defect within the ATIII molecule |
Acquired ATIII deficiency | <25% of normal ATIII concentration |
Preeclampsia | Levels unchanged in normal pregnancy |
Cirrhosis | Decreased protein synthesis |
Nephrotic syndrome | Increased urinary excretion of ATIII |
DIC | Increased consumption of ATIII |
Heparin pretreatment | 85% of normal ATIII concentration due to accelerated clearance |
Estrogen therapy | Decreased protein synthesis |
Cytotoxic drug therapy (L-asparaginase) | Decreased protein synthesis |
ATIII = antithrombin III; DIC = disseminated intravascular coagulation.
Patients receiving preoperative heparin therapy traditionally require larger heparin doses to achieve a given level of anticoagulation when that anticoagulation is measured by the ACT. Presumably, this “heparin resistance” is due to deficiencies in the level or activity of ATIII. Other possible causes include enhanced factor VIII activity and platelet dysfunction causing a decrease in ACT response to heparin. In vitro addition of ATIII enhances the ACT response to heparin. ATIII concentrate is now available and represents a reasonable method of treating patients with documented ATIII deficiency.3
Heparin-Induced Thrombocytopenia
The options for treating these patients are few. If the clinician has the luxury of being able to discontinue the heparin for a few weeks, often the antibody disappears and allows a brief period of heparinization for CPB without complication.4 Changing the tissue source of heparin was an option when bovine heparin was predominantly in use. Some types of low-molecular-weight heparin (LMWH) have been administered to patients with HIT, but reactivity of the particular LMWH with the patient’s platelets should be confirmed in vitro. Supplementing heparin administration with pharmacologic platelet inhibition using prostacyclin, iloprost, aspirin, or aspirin and dipyridamole has been reported, all with favorable outcomes. Tirofiban with unfractionated heparin has been used in this clinical circumstance. Plasmapheresis may be used to reduce antibody levels. The use of heparin could be avoided altogether through anticoagulation with direct thrombin inhibitors such as argatroban, hirudin, or bivalirudin. These thrombin inhibitors have become standard of care in the management of the patient with HIT II. Monitoring their effects during CPB is more complex.
Measurement of Heparin Sensitivity
An HDR curve can be generated manually using the baseline ACT and the ACT response to an in vivo or in vitro dose of heparin. Extrapolation to the desired ACT provides the additional heparin dose required for that ACT. Once the actual ACT response to the heparin dose is plotted, further dose-response calculations are made based on the average of the target ACT and the actual ACT (Fig. 12-3). This methodology was first described by Bull and associates and forms the scientific basis for the automated dose-response systems manufactured by Hemochron and Hemotec. The Hemochron RxDx system uses the heparin-response test (HRT), which is an ACT with a known quantity of in vitro heparin (3 IU/mL). Using an algorithm that incorporates the patient’s baseline ACT, estimated blood volume, and HRT, a dose-response curve is generated that enables calculation of the heparin dose required to attain the target ACT. The patient’s heparin sensitivity can be calculated in seconds/IU/mL by dividing the HRT by 3 IU/mL.
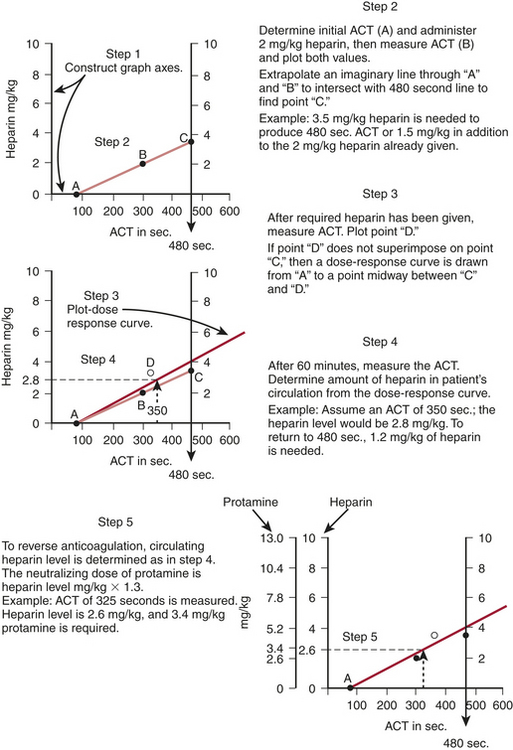
Figure 12-3 Construction of a dose-response curve for heparin. ACT = activated coagulation time.
(From Bull BS, Huse WM, Brauer FS, et al: Heparin therapy during extracorporeal circulation: II. The use of a dose-response curve to individualize heparin and protamine dosage. J Thorac Cardiovasc Surg 69:685-689, 1975.)
The RxDx system also provides an individualized protamine dose using the protamine response test (PRT). This is an ACT with one of two specific quantities of protamine, depending on the amount of circulating heparin suspected (2 IU/mL or 3 IU/mL). Using the patient’s heparinized ACT, the PRT, and an estimate of the patient’s blood volume, the protamine dose needed to return the ACT to baseline can be calculated based on a protamine-response curve. Jobes and coworkers reported that the heparin dose directed by the RxDx system resulted in ACT values well above the target ACT. In their patients, in vivo heparin sensitivity was higher than in vitro sensitivity. RxDx also resulted in lower protamine doses, lower postoperative mediastinal tube losses, and reduced transfusion requirements compared with a ratio-based system of heparin/protamine administration.5 In a larger study that standardized the treatment of heparin rebound, the reduced protamine dose was confirmed; however, the reductions in bleeding were not substantiated. The use of a protamine dose-response curve has been shown to successfully reduce the protamine dose in vascular surgery compared with standard weight-based protamine dosing.6
The Hepcon HMS system uses the HDR cartridge in the Hepcon instrument (Fig. 12-4). Each cartridge houses six chambers. Chambers 1 and 2 contain heparin at a concentration of 2.5 U/mL, chambers 3 and 4 contain heparin at a concentration of 1.5 U/mL, and chambers 5 and 6 do not contain heparin. Once information regarding patient weight, height, and CPB prime volume is entered, the information that can be obtained from this test includes the baseline ACT (chambers 5 and 6) and an HDR slope. The dose-response slope, which is the increase in ACT from 1.5 U/mL to 2.5 U/mL heparin, is extrapolated to the desired target ACT or target heparin concentration and the heparin dose is calculated.
Heparin Concentration
In a prospective randomized trial, Despotis and colleagues demonstrated that by using a transfusion algorithm in association with Hepcon-based heparin management, chest tube drainage was minimally reduced and transfusion of non–red blood cell products could be significantly reduced relative to a group of patients who had ACT-based heparin management.7 They attributed their results to better preservation of the coagulation system by high heparin doses because the doses of heparin administered in the Hepcon group were nearly twice the doses used in the ACT management group. The Hepcon, remains one of the more sensitive tests for detecting residual heparinization after protamine reversal because the heparin concentration can be measured by protamine titration to levels as low as 0.4 IU/mL.
HEPARIN NEUTRALIZATION
Protamine Effects on Coagulation Monitoring
In addition to hemodynamic sequelae, protamine has adverse effects on coagulation.8 Large doses prolong the WBCT and the ACT, possibly via thrombin inhibition. In animals and in humans, protamine has been associated with thrombocytopenia, likely due to activation of the complement cascade. The anticoagulant effect of protamine may also be due to inhibition of platelet aggregation, alteration in the platelet surface membrane, or depression of the platelet response to various agonists. These alterations in platelet function result from the presence of the heparin-protamine complex, not protamine alone. Protamine-heparin complexes activate ATIII in vitro and result in complement activation. The anticoagulant effects of free protamine occur when protamine is given in doses in excess of those used clinically; however, the risk of free protamine being the cause of a hemostatic defect is small, given the rapid clearance of protamine relative to heparin.
HEPARIN NEUTRALIZATION MONITORS
To administer the appropriate dose of protamine at the conclusion of CPB, it would be ideal to measure the concentration of heparin present and give the dose of protamine necessary to neutralize only the circulating heparin. As a result of heparin metabolism and elimination, which vary considerably among individuals, the dose of protamine required to reverse a given dose of heparin decreases over time. Furthermore, protamine antagonizes the anti-IIa effects of heparin more effectively than the anti-Xa effects and thus varies in its potency depending on the source of heparin and its anti-IIa properties. Administration of a large fixed dose of protamine or a dose based on the total heparin dose given is no longer the standard of care and may result in an increased incidence of protamine-related adverse effects. An optimal dose of protamine is desired because unneutralized heparin results in clinical bleeding and an excess of protamine may produce an undesired coagulopathy. The use of individualized protamine dose-response curves uniformly results in a reduced protamine dose and has been shown to reduce postoperative bleeding.9 One such dose-response test, the Hemochron PRT test, is an ACT performed on a heparinized blood sample that contains a known quantity of protamine. With knowledge of the ACT, PRT, and the estimated blood volume of the patient, the protamine dose needed to neutralize the existing heparin level can be extrapolated. The Hepcon instrument also has a protamine dose-response test, which is the protamine titration assay. The chamber that clots first contains the dose of protamine that most closely approximates the circulating dose of heparin. By measuring the circulating heparin level, the protamine dose required for its neutralization is calculated based on a specified heparin/protamine dose ratio.
At the levels of heparinization needed for cardiac surgery, tests that are sensitive to heparin become not clottable. ACT is relatively insensitive to heparin and is ideal for monitoring anticoagulation at high heparin levels but is too insensitive to accurately diagnose incomplete heparin neutralization. ACT had a high predictive value for adequate anticoagulation (confirmed by laboratory activated partial thromboplastin time [aPTT]) when greater than 225 seconds but was poorly predictive for inadequate anticoagulation when less than 225 seconds. The low levels of heparin present when heparin is incompletely neutralized are best measured by other more sensitive tests of heparin-induced anticoagulation, such as heparin concentration, aPTT, and TT. Thus, after CPB, confirmation of return to the unanticoagulated state should be performed with a sensitive test for heparin anticoagulation10 (Box 12-1).
Heparinase
Heparinase (Neutralase I) is an enzyme that specifically degrades heparin by catalyzing cleavage of the saccharide bonds found in the heparin molecule. As demonstrated by the ACT, heparinase in a dose of 5 μg/kg has been shown to successfully neutralize heparin effects in healthy volunteers and in patients who have undergone CPB. A dose of 7 μg/kg has been demonstrated to be even more efficacious in returning ACT to baseline values. Doses sufficient to neutralize a dose of 300 U/kg had no significant hemodynamic effects in a canine model.11 Investigators have not found any platelet-depressive effects of heparinase in contrast to the well-documented platelet dysfunction associated with protamine therapy. Return to the unanticoagulated state after the use of heparinase has been confirmed using ACT monitoring or heparin concentration monitoring.
TESTS OF COAGULATION
Bedside Tests of Coagulation
Many investigators have studied the Ciba Corning Biotrack system for monitoring anticoagulation in different clinical scenarios. For patients receiving oral anticoagulant therapy, the Biotrack 512 monitor has been found to be suitable for monitoring PT and INR. The bedside Biotrack aPTT with the laboratory aPTT and heparin level in patients receiving therapeutic heparinization after interventional cardiac catheterization have been compared. The authors found a strong correlation (r = 0.89) between the Biotrack aPTT and the aPTT from the hospital laboratory. The correlation between Biotrack aPTT and heparin level was not strong, probably because of the many other factors such as heparin neutralization and clearance that affect the heparin concentration in vivo. Another study in patients receiving heparin compared the Ciba Corning Biotrack aPTT assay with standard laboratory aPTT and documented that Biotrack was less sensitive to heparin than the laboratory aPTT; however, the correlation coefficient of these two tests was r = 0.82. In patients on warfarin therapy, the Biotrack aPTT was more sensitive than the laboratory aPTT and yielded consistently higher results for the aPTT value. In another study in patients being anticoagulated for nonsurgical applications, the bedside aPTT was similar to the standard aPTT in its prediction of treatment in simple therapeutic algorithms. However, in more complex clinical situations, there was less agreement between the bedside aPTT and laboratory aPTT.12
In a comparison of bedside coagulation monitors after cardiac surgical procedures, acceptable accuracy and precision levels for Hemochron and Ciba Corning Biotrack PT in comparison with standard laboratory plasma PT, were documented, making them potentially valuable for use in the perioperative period. Neither Hemochron nor Ciba Corning aPTT reached this level of clinical competence compared with standard laboratory tests. Others have documented that this monitor seems to be more precise for PT than for aPTT. Because of rapid turn-around times, these point-of-care coagulation monitors may be useful in predicting patients who will bleed after cardiac surgery and have also been successfully used in transfusion algorithms to decrease the number of allogeneic blood products given to cardiac surgical patients.13
MONITORING FIBRINOLYSIS
End Products of Fibrin Degradation
Other methods for quantifying fibrinolysis include measurement of the end products of fibrin degradation. Fibrin degradation products are the result of the cleavage of fibrin monomers and polymers and can be measured using a latex agglutination assay. When plasmin cleaves cross-linked fibrin, dimeric units are formed that comprise one D-domain from each of two adjacent fibrin units. These “D-dimers” are frequently measured by researchers in clinical and laboratory investigations. They are measured by either enzyme-linked immunosorbent assays or latex agglutination techniques and thus are not available for on-site use. Controversy still exists regarding whether D-dimer level or fibrin degradation products are the most sensitive test for detecting fibrinolysis, but most agree that the presence of D-dimers is the most specific for cross-linked fibrin degradation.14
Monitoring the Thrombin Inhibitors
A new class of drugs, the selective thrombin inhibitors, is a viable alternative to heparin anticoagulation for CPB. These agents include hirudin, argatroban, and other experimental agents. A major advantage of these agents over heparin is that they are able to effectively inhibit clot-bound thrombin in an ATIII-independent fashion. The platelet thrombin receptor is believed to be the focus of thrombin’s procoagulant effects in states of thrombosis such as after coronary artery angioplasty. Because surface-bound thrombin is more effectively suppressed, thrombin generation can be reduced at lower levels of systemic anticoagulation than are achieved during anticoagulation by the heparin-ATIII complex. This translates into less bleeding despite the lack of a clinically useful antidote for the thrombin antagonists.15 Thrombin antagonists are also not susceptible to neutralization by PF4 and thus are not neutralized at endothelial sites where activated platelets reside. They are also useful in patients with HIT in whom the administration of heparin and subsequent antibody-induced platelet aggregation would be dangerous.
Hirudin
Hirudin, a coagulation inhibitor isolated from the salivary glands of the medicinal leech (Hirudo medicinalis), is a potent inhibitor of thrombin that, unlike heparin, acts independently of ATIII and inhibits clot-bound thrombin as well as fluid-phase thrombin. Hirudin does not require a cofactor and is not susceptible to neutralization by PF4. This would seem to be beneficial in patients in whom platelet activation and thrombosis are potential problems. Recombinant hirudin (r-hirudin) was administered as a 0.25-mg/kg bolus and an infusion to maintain the hirudin concentration at 2.5 μg/mL as determined by the ecarin clotting time in studies by Koster and coworkers.16 The ecarin clotting time, modified for use in the TAS analyzer, has been used in large series of patients with HIT. Compared with standard treatment with heparin or LMWHs, r-hirudin–treated patients maintained platelet counts and hemoglobin levels and had few bleeding complications if renal function was normal. Hirudin is a small molecule (molecular weight 7 kDa) that is eliminated by the kidney and is easily hemofiltered at the end of CPB.
Bivalirudin
Bivalirudin is a small 20–amino acid molecule with a plasma half-life of 24 minutes. It is a synthetic derivative of hirudin and thus acts as a direct thrombin inhibitor. Bivalirudin binds to both the catalytic binding site and the anion-binding exosite on fluid phase and clot-bound thrombin. The part of the molecule that binds to thrombin is actually cleaved by thrombin itself, so the elimination of bivalirudin activity is independent of specific organ metabolism. Bivalirudin has been used successfully as an anticoagulant in interventional cardiology procedures as a replacement for heparin therapy. In fact, in interventional cardiology, bivalirudin has been associated with less bleeding and equivalent ischemic outcomes compared with heparin plus a platelet inhibitor. This may be the result of bivalirudin being both an antithrombin anticoagulant and an antithrombin at the level of the platelet. Merry and colleagues showed equivalence with regard to bleeding outcomes and an improvement in graft flow after off-pump cardiac surgery when bivalirudin was used (0.75-mg/kg bolus, 1.75-mg/kg/hr infusion).17 Case reports confirm the safety of bivalirudin use during CPB, although current trials are under way. Monitoring of anticoagulant activity is performed using the ecarin clotting time with similar prolongation to that seen with hirudin anticoagulation.18 The ecarin clotting time has a closer correlation with anti-IIa activity and plasma drug levels than does the ACT. For this reason, standard ACT monitoring during antithrombin therapy is not preferred if ecarin clotting time can be measured.
Bivalirudin has been favorably compared with heparin in patients undergoing coronary angioplasty for unstable angina. The half-life of aPTT prolongation is approximately 40 minutes, and reductions in formation of fibrinopeptide A are evidence of thrombin inhibition and fibrinogen preservation. Careful monitoring should be used because there may be a rebound prothrombotic state after cessation of therapy, which could lead to recurrence of anginal symptoms (Box 12-2).
MONITORING PLATELET FUNCTION
Platelet Count
Protamine-heparin complexes and protamine alone also contribute to platelet depression after CPB. Mild to moderate degrees of hypothermia are associated with reversible degrees of platelet activation and platelet dysfunction, which may be partly mitigated by the use of aprotinin therapy. Overall, the potential coagulation benefits of normothermic CPB compared with hypothermic CPB require further study in well-conducted randomized trials (Box 12-3).
Platelet-Mediated Force Transduction
An instrument that measures the force developed by platelets during clot retraction has been shown to be directly related to platelet concentration and function19 (Hemodyne). The apparatus consists of a cup and a parallel upper plate. The cup is filled with blood or the platelet-containing solution, and the upper plate is lowered onto the clotting solution. Clot forms and adheres to the outer edges of the cup and to the plate above. A thin layer of oil is deposited onto the surfaces that are exposed to air. The upper plate is coupled to a displacement transducer that translates displacement due to platelet retraction into a force. Normal values for platelet force development have been suggested by the investigators. The antiplatelet effects of heparin have been evaluated using this force retractometer. Using this instrument, investigators have shown that high heparin concentrations completely abolish platelet force generation. Furthermore, the concentration of protamine required to reverse the anticoagulant effects of heparin is not sufficient to reverse these antiplatelet effects. The antiplatelet effects of protamine alone have also been evaluated using this monitor.
BEDSIDE PLATELET FUNCTION TESTING
Thromboelastography
The coaguloviscometers that were developed in the 1920s formed the basis of viscoelastic coagulation testing that is now known as thromboelastography. Thromboelastography in its current form was developed by Hartert in 1948 and has been used in many different clinical scenarios to diagnose coagulation abnormalities.20 Although not yet truly portable, the thromboelastograph (TEG; Haemoscope, Skokie, IL) can be performed “on site” either in the operating room or in a laboratory and provides a rapid whole blood analysis that yields information about clot formation and clot dissolution. Within minutes, information is obtained regarding the integrity of the coagulation cascade, platelet function, platelet-fibrin interactions, and fibrinolysis. The principle is as follows: whole blood (0.36 mL) is placed into a plastic cuvette into which a plastic pin is suspended; this plastic pin is attached to a torsion wire that is coupled to an amplifier and recorded; a thin layer of oil is added to the surface of the blood to prevent drying of the specimen; and the cuvette oscillates through an arc of 4 degrees, 45 minutes at 37°C. When the blood is liquid, movement of the cuvette does not affect the pin. However, as clot begins to form, the pin becomes coupled to the motion of the cuvette and the torsion wire generates a signal that is recorded. The recorded tracing can be stored by computer, and the parameters of interest are calculated using a simple software package. Alternatively, the tracing can be generated on line with a recording speed of 2 mm/min. The tracing generated has a characteristic conformation that is the signature of the TEG (Fig. 12-5).
Characteristic TEG tracings can be recognized to be indicative of particular coagulation defects. A prolonged R value indicates a deficiency in coagulation factor activity or level and is seen typically in patients with liver disease and in patients on anticoagulants such as warfarin or heparin. MA and a angle are reduced in states associated with platelet dysfunction or thrombocytopenia and are even further reduced in the presence of a fibrinogen defect. LY 30, or the lysis index at 30 minutes after MA, is increased in conjunction with fibrinolysis. These particular signature tracings are depicted in Figure 12-6.
TEG is a useful tool for diagnosing and treating perioperative coagulopathy in patients undergoing cardiac surgical procedures due to a variety of potential coagulation defects that may exist. Within 15 to 30 minutes, on-site information is available regarding the integrity of the coagulation system, the platelet function, fibrinogen function, and fibrinolysis. With the addition of heparinase, TEG can be performed during CPB and can provide valuable and timely information regarding coagulation status.21 Because TEG is a viscoelastic test and evaluates whole blood hemostasis interactions, it is suggested that TEG is a more accurate predictor of postoperative hemorrhage than routine coagulation tests that analyze individual components of the hemostasis system. A number of clinical trials have confirmed that in cardiac surgical patients TEG has a greater predictive value and greater specificity than routine coagulation tests for diagnosing patients known as “bleeders.” Tuman and associates studied 42 patients, of whom 9 were classified as bleeders.21 A routine coagulation screen consisting of ACT, PT, aPTT, and platelet count had only a 33% accuracy for predicting bleeding, whereas TEG and Sonoclot (Sienco Inc., Morrison, CO) (another viscoelastic test) had 88% and 74% accuracy, respectively. Other investigators have also found that TEG abnormalities predict postoperative bleeding and, using TEG parameters, they were also able to identify a population of patients who respond to therapy with desmopressin acetate.
In a large retrospective evaluation in more than 1000 patients, Spiess and associates found that the institution of a transfusion algorithm using TEG resulted in a significant reduction in the incidence of mediastinal exploration and in the rate of transfusion of allogeneic blood products.22 Because of its ease of use and application at the bedside, TEG has been used in many research settings to assess drug effects on platelet function and clot strength.
Thromboelastography Modifications
Thromboelastography was originally performed using recalcified citrated whole blood or celite activator. The addition of recombinant human tissue factor as an activator can accelerate the rate of thrombin formation and thus the formation of fibrin. This serves to shorten the time required for development of the MA. Because the MA is primarily reflective of clot strength and platelet function, this information can be obtained more quickly with tissue factor enhancement. The recombinant tissue factor is a thromboplastin agent and is available from a number of manufacturers.23
The thienopyridine ADP-receptor blockers clopidogrel and ticlopidine are widely used in cardiovascular medicine. The ability to measure the platelet defect induced by these drugs is very difficult unless sophisticated laboratory techniques such as ADP-aggregometry are used. Aggregometry yields accurate results; however, it is not readily available in the perioperative period as a point-of-care test. Native TEG analysis does not measure the thienopyridine-induced platelet defect because the formation of thrombin in the assay has an overwhelming effect on the development of the TEG MA. A modification of the TEG removes thrombin from the assay and studies a nonthrombin clot, strengthened by the addition of ADP. Figure 12-7 depicts the different signature TEG tracings that are used to calculate the platelet contribution to MA when a platelet inhibitor is present. This assay was specifically created to measure the platelet inhibition by ADP antagonists such as clopidogrel and is referred to as the platelet mapping assay. The MAkh is the maximal activation of platelets and fibrin and is the largest amplitude that can be achieved. The MAf is the MA that is obtained when a thrombin-depleted fibrin clot is formed without a platelet contribution. The MApi is the MAf contribution plus the platelet contribution. MApi is created by adding an activator such as ADP to the MAf assay (for clopidogrel testing). Only platelets that can be activated by ADP contribute to the MApi. The following formula calculates the percent reduction in platelet activity using this assay.
Clopidogrel, ticlopidine, and even aspirin inhibition can now be studied at the point-of-care using this modification.24
Tests of Platelet Response to Agonist
HemoSTATUS
An initial investigation of HemoSTATUS in cardiac surgical patients was performed by Despotis and colleagues.25 The authors studied 150 patients and conducted multivariate analyses to evaluate the relationship between postoperative blood loss and multiple demographic, operative, and hemostatic measurements. They demonstrated a significant correlation between HemoSTATUS measurements on arrival in the intensive care unit (ICU) and 4-hour postoperative mediastinal tube drainage (r = −0.85, channel 5; r = −0.82, channel 6). Using receiver operating characteristic (ROC) curves for the detection of excessive mediastinal tube drainage, the accuracy of a number of hemostasis assays was measured. The highest predictability for bleeding was found in both the channel 5 clot ratio and the bleeding time. The PT, aPTT, and platelet count had much lower predictive value. HemoSTATUS-derived clot ratios also had the capability to detect enhanced platelet function after the administration of pharmacologic platelet therapy (desmopressin acetate) and after the transfusion of platelet concentrates. Subsequent investigations in cardiac surgical patients have confirmed a significant yet weak correlation of HemoSTATUS with postoperative bleeding but have not found this test to be superior to TEG or routine coagulation tests in its predictive value.
SUMMARY
1. Reich D.L., Zahl K., Perucho M.H., Thys D.M. An evaluation of two activated clotting time monitors during cardiac surgery. J Clin Monit. 1992;8:33.
2. Linden M.D., Schneider M., Baker S., et al. Decreased concentration of antithrombin after preoperative therapeutic heparin does not cause heparin resistance during cardiopulmonary bypass. J Cardiothorac Vasc Anesth. 2004;18:131.
3. Levy J.H., Montes F., Szlam F., Hillyer C.D. The in vitro effects of antithrombin III on the activated coagulation time in patients on heparin therapy. Anesth Analg. 2000;90:1076.
4. Warkentin T.E., Greinacher A. Heparin-induced thrombocytopenia and cardiac surgery. Ann Thorac Surg. 2003;76:638.
5. Jobes D.R., Aitken G.L., Shaffer G.W. Increased accuracy and precision of heparin and protamine dosing reduces blood loss and transfusion in patients undergoing primary cardiac operations. J Thorac Cardiovasc Surg. 1995;110:36.
6. Szalados J.E., Ouriel K., Shapiro J.R. Use of the activated coagulation time and heparin dose-response curve for the determination of protamine dosage in vascular surgery. J Cardiothorac Vasc Anesth. 1994;8:515.
7. Despotis G.J., Joist J.H., Hogue C.W.Jr., et al. The impact of heparin concentration and activated clotting time monitoring on blood conservation: A prospective, randomized evaluation in patients undergoing cardiac operation. J Thorac Cardiovasc Surg. 1995;110:46.
8. Warkentin T.E., Crowther M.A. Reversing anticoagulants both old and new. Can J Anaesth. 2002;49:S11.
9. LaDuca F.M., Zucker M.L., Walker C.E. Assessing heparin neutralization following cardiac surgery: Sensitivity of thrombin time-based assays versus protamine titration methods. Perfusion. 1999;14:181.
10. Shigeta O., Kojima H., Hiramatsu Y., et al. Low-dose protamine based on heparin-protamine titration method reduces platelet dysfunction after cardiopulmonary bypass. J Thorac Cardiovasc Surg. 1999;118:354.
11. Levy J.H., Cormack J.G., Morales A. Heparin neutralization by recombinant platelet factor 4 and protamine. Anesth Analg. 1995;81:35.
12. Werner M., Gallagher J.V., Ballo M.S., Karcher D.S. Effect of analytic uncertainty of conventional and point-of-care assays of activated partial thromboplastin time on clinical decisions in heparin therapy. Am J Clin Pathol. 1994;102:237.
13. Nuttall G.A., Oliver W.C., Beynen F.M., et al. Determination of normal versus abnormal activated partial thromboplastin time and prothrombin time after cardiopulmonary bypass. J Cardiothorac Vasc Anesth. 1995;9:355.
14. Whitten C.W., Greilich P.E., Ivy R., et al. D-dimer formation during cardiac and noncardiac thoracic surgery. Anesth Analg. 1999;88:1226.
15. Lincoff A.M., Bittl J.A., Kleiman N.S., et al. Comparison of bivalirudin versus heparin during percutaneous coronary intervention (the Randomized Evaluation of PCI Linking Angiomax to Reduced Clinical Events [REPLACE] trial). Am J Cardiol. 2004;93:1092.
16. Koster A., Hansen R., Grauhan O., et al. Hirudin monitoring using the TAS ecarin clotting time in patients with heparin-induced thrombocytopenia type II. J Cardiothorac Vasc Anesth. 2000;14:249.
17. Merry A.F., Raudkivi P.J., Middleton N.G., et al. Bivalirudin versus heparin and protamine in off-pump coronary artery bypass surgery. Ann Thorac Surg. 2004;77:925.
18. Koster A., Spiess B., Chew D.P., et al. Effectiveness of bivalirudin as a replacement for heparin during cardiopulmonary bypass in patients undergoing coronary artery bypass grafting. Am J Cardiol. 2004;93:356.
19. Carr M.E.Jr. In vitro assessment of platelet function. Transfus Med Rev. 1997;11:106.
20. Spiess B.D. Thromboelastography and cardiopulmonary bypass. Semin Thromb Hemost. 1995;21:27.
21. Tuman K.J., McCarthy R.J., Djuric M., et al. Evaluation of coagulation during cardiopulmonary bypass with a heparinase-modified thromboelastographic assay. J Cardiothorac Vasc Anesth. 1994;8:144.
22. Spiess B.D., Gillies B.S., Chandler W., Verrier E. Changes in transfusion therapy and reexploration rate after institution of a blood management program in cardiac surgical patients. J Cardiothorac Vasc Anesth. 1995;9:168.
23. Shore-Lesserson L., Manspeizer H.E., DePerio M., et al. Thromboelastography-guided transfusion algorithm reduces transfusions in complex cardiac surgery. Anesth Analg. 1999;88:312.
24. Bliden K.P., Dichiara J., Tantry U., et al. Increased risk in patients with high platelet aggregation receiving chronic clopidogrel therapy. J Amer Coll Cardiol. 2007;49:657.
25. Despotis G.J., Levine V., Saleem R., et al. Use of point-of-care test in identification of patients who can benefit from desmopressin during cardiac surgery: A randomised controlled trial. Lancet. 1999;354:106.