Chapter 20 Closed Head Injury
• The first priority in treating the head-injured patient is prompt physiological resuscitation—restoration of blood pressure, oxygenation, and ventilation and obtaining a postresuscitation Glasgow Coma Scale (GCS) score.
• Episodes of hypotension or hypoxia greatly increase risk of morbidity and death after severe head injury; therefore, even a single episode should be avoided if possible.
• Available literature indicates that glucocorticoids do not lower intracranial pressure (ICP) or improve outcome in patients with severe head injury; therefore, the routine use of steroids is not recommended.
• Mannitol is effective in reducing intracranial hypertension but is not to be used as a prophylactic treatment. Recent evidence suggests that hypertonic saline may be as effective, if not more effective, than mannitol for reduction of ICP and maintaining cerebral blood flow/cerebral perfusion pressure.
• Cerebral ischemia may be the single most important secondary event affecting outcome following severe traumatic brain injury (TBI); maintaining the cerebral perfusion pressure ideally greater than 60 mm Hg may help avoid both global and regional ischemia. However, pushing the cerebral perfusion pressure much higher may have undesirable pulmonary effects. ICP monitoring as well as brain oxygen tension monitoring are associated with improved outcomes in TBI patients.
• Chronic prophylactic hyperventilation should be avoided during the first 5 days after a severe TBI, and particularly during the first 24 hours. Prophylactic hyperventilation therapy further reduces cerebral blood flow and thus has been associated with poorer outcomes. It should be used in an acutely deteriorating patient as a temporizing measure until a definitive treatment may be initiated.
• Antiepileptic medications show no reduction in late onset seizures (>7 days after injury). Dilantin or Keppra is recommended for the first 7 days after TBI to help reduce the risk of early onset seizure (<7 days after injury).
• The ventriculostomy remains the gold standard for ICP monitoring and is part of the treatment of elevated ICP. Brain oxygen tension monitors and temperature probes show promise with reductions in morbidity and mortality rates. More comprehensive monitors are under development.
Intracranial hemorrhages complicate 25% to 45% of severe TBI cases, 3% to 12% of moderate TBI cases, and 1 in 500 persons with mild TBI.1 As the skull is impacted, the force is transmitted intracranially and brain movement can lead to disparate types of intracranial hemorrhages. These hemorrhages include subdural hematomas, epidural hematomas, traumatic subarachnoid hemorrhages, intraparenchymal hemorrhages, intracerebral contusions, or combinations of the aforementioned.
Although controlled and strictly comparable data are hard to come by, it is evident that significant strides have been made in our understanding of this condition and in its management. The mortality rate from severe TBI has steadily decreased from 50% to 35% to 25% and even lower over the past three decades.2 Difficult to prove conclusively, the likely causes of this decline include air bags, seat belts, and better-built cars; improved prehospital evaluation and resuscitation; rapid transport to trauma centers; prompt imaging and intervention; surgical decompression; and better neurological critical care. All of these efforts serve to limit secondary brain injury.
The first evidence-based guidelines for the management of TBI patients were published in 1995,3 with an updated version published in 2000.4 Also in 2000, the Cochrane Library published a series of reviews that provided evidence-based standards and guidelines for the management of TBI patients.5–7 The third edition of evidence-based guidelines for the management of severe traumatic brain injury were published in 2007, and are the most current and up-to-date recommendations on the management and treatment of TBI. Evidence-based guidelines for the management of penetrating head injury have also been published,8 as well as guidelines for prehospital and pediatric TBI management.9–11 The Brain Trauma Foundation (BTF) maintains and updates most of these guidelines every few years and they can be reviewed in full at the BTF website (www.braintrauma.org).
Better understanding of the pathophysiology of TBI has led to better neurological critical care aimed at the prevention of secondary injury to the at-risk brain (Fig. 20.1). Along with better monitoring techniques, this has translated into strict parameters for maintaining oxygen saturation and preventing hyponatremia and hyperglycemia. Unfortunately these parameters can often be difficult to control, as many trauma patients have other injuries as well that predispose them to hypoxia, hypotension, anemia, coagulopathies, intracranial mass lesions, and elevated intracranial pressure (ICP). The hallmark treatment of TBI is the monitoring and control of ICP.
While better critical care and physiological management have proved to be very valuable, pharmacological interventions have for the most part yet to be proved effective in the clinical setting. Despite promising results in animal models, clinical trials to date have not generated drug treatments with proven benefit.12
Classification
Although head injuries could be classified in several different ways, the most practical categorizations are based on mechanism, severity, and morphology (Fig. 20.2).
Severity
Prior to 1974, different authors used various terms to describe patients with head injury, making it virtually impossible to compare groups of patients from different centers. In 1974, Teasdale and Jennett,13 by identifying the clinical signs that predicted outcome most reliably and that seemed to have the least interobserver variation, designed what has come to be known as the Glasgow Coma Scale (GCS). The introduction of the GCS (Table 20.1) brought some degree of uniformity into the head injury literature.13 This scale has gained widespread use for the description of patients with head injuries and has also been adopted for the description of patients with altered levels of consciousness due to other causes.
Scoring Component | Points Assigned |
---|---|
Eye opening (E) | |
Spontaneous | 4 |
To call | 3 |
To pain | 2 |
None | 1 |
Motor response (M) | |
Obeys commands | 6 |
Localizes pain | 5 |
Normal flexion (withdrawal) | 4 |
Abnormal flexion (decorticate) | 3 |
Extension (decerebrate) | 2 |
None (flaccid) | 1 |
Verbal response (V) | |
Oriented | 5 |
Confused conversation | 4 |
Inappropriate words | 3 |
Incomprehensible sounds | 2 |
None | 1 |
Scoring | |
GCS sum score = (E + M + V); best possible score = 15; worst possible score = 3. |
Jennett and Teasdale defined coma as the inability to obey commands, to utter words, and to open the eyes.14 A patient needs to meet all three aspects of this definition to be classified as comatose. In a series of 2000 patients with severe head injury, these authors observed 4% who did not speak but could obey commands and another 4% who uttered words but did not obey commands. Among patients who could neither obey nor speak, 16% opened their eyes and were therefore judged not to be in coma. Patients who open their eyes spontaneously, obey commands, and are oriented score a total of 15 points, whereas flaccid patients who do not open their eyes or talk score the minimum of 3 points. No single score within the range of 3 to 15 forms the cut-off point for coma. However, 90% of all patients with a score of 8 or less, and none of those with a score of 9 or more, are found to be in coma according to the preceding definition. Therefore, for all practical purposes, a GCS score of 8 or less has become the generally accepted definition of a comatose patient.
The distinction between patients with severe head injury and those with mild to moderate injury is thus fairly clear. However, distinguishing between mild and moderate head injury is more of a problem.15 Somewhat arbitrarily, head-injured patients with a GCS score of 9 to 13 have been categorized as “moderate,” and those with a GCS score of 14 or 15 have been designated “mild.” Authors have variably classified the GCS 13 patients with the mild or moderate TBI groups, but studies suggest that patients with a GCS of 13 at admission fit better into the moderate rather than mild TBI group. Eighty percent of head injuries are categorized as mild, 10% as moderate, and 10% as severe. Williams and colleagues reported that the neurobehavioral deficits in patients with mild head injury (GCS 14 or 15) and an intracranial lesion on initial computed tomography (CT) scan were similar to those in patients with moderate head injury (GCS 9 to 13), but patients with mild head injury uncomplicated by an intracranial lesion on CT scan did significantly better.16
Morphology
Skull Fractures
In current practice in developed countries, CT scans are the imaging study of choice and plain skull films are rarely obtained. Skull fractures may be seen in the cranial vault or skull base, may be linear or stellate, and may be depressed or nondepressed (Fig. 20.3). Basal skull fractures are harder to document on plain x-ray films and usually require CT scanning with bone-window settings for identification. Another indication of covert skull fracture is the presence of pneumocephalus, which can be readily identified on CT scan.
Fractures can also involve the anterior or posterior tables of the frontal sinus. These fractures can lead to inadequate drainage and recurrent sinusitis if the communication between the sinus and nasal cavity (nasofrontal outflow tract) is obstructed. Furthermore, if the posterior table is violated with obstruction of the nasofrontal outflow tract, there is an increased risk of intracranial infections. Treatment of such fractures should be based on the fine cut CT bone window findings, patency of the nasofrontal outflow tract, and cosmetic deformity caused by the fracture.17,18
Intracranial Lesions
Intracranially lesions may be broadly classified into focal or diffuse injuries. It is not uncommon to for these types of injuries to coexist. Focal lesions include epidural hematomas, subdural hematomas, and contusions/intracerebral hematomas. Diffuse brain injury, also referred to as diffuse axonal injury, may have a relatively benign-appearing CT scan (Fig. 20.4A); however, small punctate hemorrhages may be noted particularly at midline structures. Patients with diffuse axonal injury typically have a poor neurological examination with altered sensorium or even deep coma out of proportion to the findings on their imaging workup.
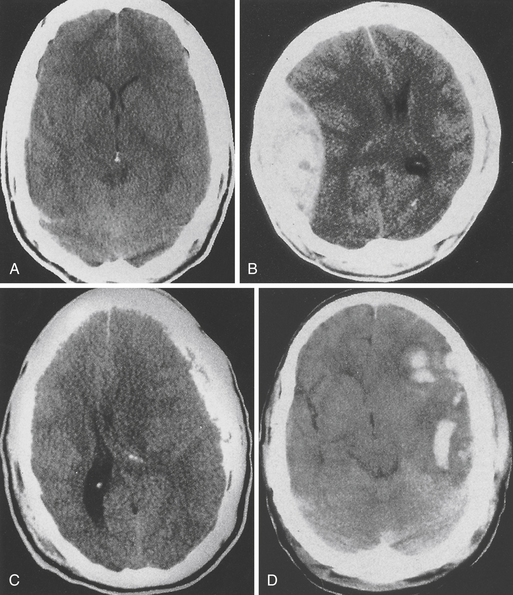
FIGURE 20.4 A, Diffuse brain injury; B, epidural hematoma; C, subdural hematoma; and D, intracerebral hematoma.
Epidural Hematoma (Fig. 20.4B)
This type of bleeding is located between the inner table of the skull and the dura. Most commonly these hemorrhages are located in the temporal or temporoparietal region and are classically associated with tearing of the middle meningeal artery secondary to calvarial fracture. Most epidural hematomas are related to arterial bleeding; however, in up to one third of the cases an epidural may be associated with bleeding from the bone. Epidural hematomas may also occur from tearing of the middle meningeal vein, diploic veins, or venous sinuses, particularly in the parieto-occipital area or the posterior fossa. Nonsurgical epidural hematomas are reported in 2.7% to 4% of TBI patients.10,19–22 In patients presenting with coma up to 9% may harbor an epidural hematoma. Epidural hematomas tend to affect patients between 20 and 30 and are most commonly seen in traffic accidents (53%), falls (30%), and assaults (8%).10 The mortality rate in all age groups and GCS scores undergoing surgery for epidural hematoma is approximately 10%.10 More specifically in patients not in coma the mortality rate approximates 0%, for obtunded patients it is 9%, and for patients presenting in coma it approaches 20%.
Subdural Hematoma (Fig. 20.4C)
Subdural hematoma (SDH) is a much more common entity, with reports of 12% to 29% of patients with severe TBI having an associated SDH on initial imaging.10 The BTF review of surgical subdural hematomas showed that in 2870 patients 21% presented with SDH. They occur most frequently from a tearing of bridging veins between the cerebral cortex and the draining sinuses. However, they can also be associated with lacerations of the brain surface or substance. A skull fracture may or may not be present and the mechanism is often age dependent. Younger patients (18-40 years) presented with SDH after motor vehicle accident (MVA) (56%), with only 12% presenting after a fall.23 In patients older than 65 the opposite is seen, with SDH seen in 22% of MVAs and 56% of falls, often seen in those on anticoagulants used in the management of other chronic disease processes.23 The brain damage underlying acute SDHs results from direct pressure caused by the hematoma, brain swelling, increased intracranial pressure, or diffuse axonal injury as a result of mechanical distortion of the brain parenchyma. The injury in patients with SDH is usually much more severe, and the prognosis is much worse compared to epidural hematomas. MVA is described as the mechanism of injury in 53% to 75% of patients who are comatose with SDH. The mortality rate in a general series may be around 60% but can be lowered by very rapid surgical intervention and aggressive medical management.24 Multiple studies show that the morbidity and mortality rates are increased if the surgery is performed after 3 to 4 hours, and the timing of acute SDH surgical intervention should be done within 2 to 4 hours of TBI if possible.10
Contusions/Intracerebral Hemorrhage (Fig. 20.4D)
Pure cerebral contusions are fairly common, found in 8% of all TBI10,25 and 13% to 35% of severe injuries.10 The incidence is much more apparent as the quality and number of CT scans increase. Furthermore, contusions of the brain are often concomitant with SDH. The vast majority of contusions occurs in the frontal and temporal lobes, although they can occur at almost any site, including the cerebellum and brainstem. The distinction between contusions and traumatic intracerebral hematomas remains somewhat ill-defined. The classic “salt and pepper” lesion is clearly a contusion, but a large hematoma clearly is not. However, there is a gray zone, and contusions can, over a period of hours or days, evolve into intracerebral hematomas.
Management of the intracerebral hematoma is dependent on the neurological status of the patient. Rapid surgical evacuation decompression is recommended if there is a significant mass effect (generally, a 5-mm or greater actual midline shift). The current BTF guidelines state that patients with parenchymal mass lesions and signs of progressive neurological deterioration related to the lesion, refractory intracranial hypertension, or signs of significant mass effect on CT should be treated operatively. Also in patients with GCS scores of 6 to 8 with frontal or temporal contusion greater than 20 cm3 with midline shift greater than 5 mm or cisternal compression on CT scan, and patients with any lesion greater than 50 cm3 in volume should be surgically treated.10
Diffuse Injuries
Diffuse axonal injury is the term used to describe a prolonged post-traumatic state in which there is loss of consciousness from the time of injury that continues beyond 6 hours. This phenomenon may further be broken down into mild, moderate, and severe categories.26 Severe diffuse axonal injury usually occurs in vehicular accidents, comprising about 36% of all patients with diffuse axonal injury. These patients are rendered deeply comatose and remain so for prolonged periods of time. They often demonstrate evidence of decortication or decerebration (motor posturing) and often remain severely disabled, if they survive. These patients often exhibit autonomic dysfunctions such as hypertension, hyperhidrosis, and hyperpyrexia, and were previously thought to have primary brainstem injury. It is now believed that diffuse axonal injury throughout the brain is the more common pathological basis.
Evaluation
Mild Traumatic Brain Injury
Most patients with mild head injury make “uneventful recoveries” but often suffer mild neurological sequelae that can be identified when given in-depth neuropsychological testing. Furthermore, about 3% of patients deteriorate unexpectedly, and can become neurologically devastated if the decline in mental status is not noticed early.27 How can a physician guard against such an occurrence? Early CT scanning of all patients with a history of a TBI is generally advisable, although the classic struggle between “cost-effective” and the “best possible” management is clearly evident here, and practice varies in different centers.
In 1999, a Task Force on Mild Traumatic Brain Injury was devised under the support of the European Federation of Neurological Societies. The efforts of the task force produced the recommendations for the initial management of mild traumatic brain injury (Fig. 20.5).28 Since their recommendations, further work has been done in the evaluation and treatment of mild TBI.
In the head-injured patient, skull x-ray films may be examined for the following features: linear or depressed skull fractures, position of the pineal gland if calcified, air-fluid levels in the sinuses, pneumocephalus, facial fractures, and foreign bodies. However, the routine ordering of skull films has in most instances become obsolete with the availability of CT scanners. Studies comparing skull radiography with CT have shown a low sensitivity and specificity of the presence of a skull fracture on skull radiographs for intracranial hemorrhage.29 A meta-analysis confirmed that skull radiography is of little value in the clinical assessment of mild TBI.30
The cervical spine and other parts must undergo x-ray studies if there is any pain or tenderness. Non-narcotic analgesics are preferred, although codeine may be used if there is an associated painful injury. Tetanus toxoid must be administered if there are any associated open wounds. Routine blood tests are usually not necessary if there are no systemic injuries and the patient is not on anticoagulants. A blood alcohol level and urine toxic screen can be useful both for diagnostic and for medicolegal purposes.
Moderate Traumatic Brain Injury
Patients with moderate head injury constitute approximately 10% of head injury patients seen in the emergency room. They are still able to follow simple commands but are usually confused or somnolent and may have focal neurological deficits such as a hemiparesis. In patients with mild or moderate head injury, extracranial injuries play a significant role in the overall outcome. Approximately 10% of these patients will deteriorate and lapse into coma. Therefore, they should be managed as patients with severe head injuries, although they are not routinely intubated (Fig. 20.6).
On admission to the emergency room, a brief history is obtained and cardiopulmonary stability is ensured prior to neurological assessment. A CT scan of the head is obtained in all moderately head-injured patients. In a review of 341 patients with a GCS score of 9 to 12, 40% of cases had an abnormal initial CT scan and 8% required surgery.31 The patient is admitted for observation even if the CT scan is normal. If the patient improves neurologically and a follow-up CT scan of the head shows no surgical mass lesion, he or she may be discharged from the hospital over the next few days. On the other hand, if the patient lapses into coma, the management principles described for severe head injury are adopted.
Severe Traumatic Brain Injury
Severe TBI patients are those who are unable to follow simple commands even after cardiopulmonary stabilization. Although this definition includes a wide spectrum of brain injury, it identifies the patients who are at greatest risk of suffering significant morbidity and even death. We believe that in such patients a “wait and see” approach can be disastrous and that prompt diagnosis and treatment are of the utmost importance (Box 20.1).24,32,33
Management of Severe Traumatic Brain Injury
Airway
A frequent concomitant of a severe TBI is transient respiratory arrest. Prolonged apnea may often be the cause of “immediate” death at the scene of an accident. If artificial respiration can be instituted immediately, a good outcome is possible.34 Apnea, atelectasis, aspiration, and adult respiratory distress syndrome are frequently associated with severe head injury, and by far the most important aspect of the immediate management of these patients is the establishment of a reliable airway. Patients with severe TBI should be intubated early. One hundred percent oxygen is then used for ventilation until blood gases can be checked and appropriate adjustments of the FIO2 made. There is little danger of oxygen toxicity even when 100% oxygen is used for less than 48 to 72 hours.
Blood Pressure
Hypotension and hypoxia are the principal enemies of the head-injured patient. It has been shown that the presence of hypotension (systolic blood pressure <90 mm Hg) in severe TBI patients increases the mortality rate from 27% to 50%.35 Furthermore, it was found that 35% of patients arriving at major trauma centers are hypotensive. While the airway is being established, another group of emergency room personnel should be checking the patient’s pulse and blood pressure and taking steps to obtain venous access.
The importance of routine abdominal paracentesis in the hypotensive comatose patient has been demonstrated historically.36 In most trauma centers today either high-resolution rapid CT scan or ultrasound (focused assessment with sonography for trauma, FAST) is an acceptable option to rule in or rule out intra-abdominal injury. It must be emphasized that a patient’s neurological examination is meaningless as long as he or she is hypotensive. Time after time, we have seen hypotensive patients who are unresponsive to any form of stimulation revert to a near-normal neurological examination soon after normal blood pressure has been restored.
Cardiopulmonary Stabilization
Brain injury is often adversely affected by secondary insults. In a study of 100 consecutive patients with severe brain injury evaluated on arrival in the emergency room, 30% were hypoxemic (PO2 <65 mm Hg), 13% were hypotensive (systolic blood pressure <95 mm Hg), and 12% were anemic (hematocrit <30%).37 It has subsequently been demonstrated that hypotension at admission (systolic blood pressure <90 mm Hg) is one of three factors in severe head injury with a normal CT scan (the other two being age over 40 years and motor posturing) that, when noted at admission, are associated with subsequent ICP elevation.38 High ICP is in turn associated with poorer outcome. Subsequent analyses have also confirmed a strong association between hypotension and worse outcomes in patients with severe head injury.39 Therefore, it is imperative that cardiopulmonary stabilization be achieved rapidly.
Diagnostic Radiographs
As soon as the preliminary steps toward cardiopulmonary stabilization have been taken, diagnostic radiographs should be obtained. Cervical spine films (cross-table lateral and anteroposterior) or a thin-cut cervical spine CT scan are the first to be taken in the severely traumatized patient and must be read by a knowledgeable reader before the patient’s neck can be moved. Features to look for in this study are loss of alignment of the vertebral bodies, bony fractures or compressions, loss of alignment of the facet joints, and prevertebral soft tissue swelling (more than 5 mm opposite the C3 vertebral body is significant). Every effort must be made to visualize the lower cervical levels (C6-T1); these are often obscured by the shoulders, especially in heavy-set patients. On plain films, fracture-subluxations at these levels may be overlooked if the films are not repeated with caudal traction on both arms and greater x-ray penetration (Fig. 20.7).40 If these maneuvers also fail, a “swimmer’s view” lateral film can be obtained. If any of these films shows any of the abnormalities listed here, the neck must remain immobilized in a hard collar (Philadelphia, Aspen, or Miami J) pending further studies (high-resolution CT scan). In many centers, plain films of the cervical spine have been replaced by a thin-cut CT scan as the initial study.
Cervical spine CT is indicated for the unconscious patient with suspicious or inadequate cervical radiographs and with all cervical fractures or suspected fractures on initial plain films. Several studies have demonstrated the value of the full CT scan, with sagittal and coronal reconstructions, for the exclusion of significant spinal injury.41 Widening, slippage, or rotational abnormalities of the cervical vertebrae suggest soft tissue injury. An absence of such signs appears to exclude significant instability. Additional modalities, such as magnetic resonance imaging (MRI), can also be employed, although these studies are not generally used as initial studies.
The chest film is useful in ruling out endotracheal tube malposition, pneumothorax, hemothorax, lung contusion, hemopericardium, rib fractures, thoracic spine fractures, and other thoracic injury that may have a bearing on patient management.
General Examination
During the process of cardiopulmonary stabilization, the clinician conducts a rapid general examination looking for other injuries. In one series of severe TBI patients, more than 50% had additional major systemic injuries requiring care by other specialists (Table 20.2).37 One must check for head and neck, thoracic, abdominal, pelvic, and spinal injuries, and injuries involving extremities.
TABLE 20.2 Frequency of Systemic Injuries in Patients with Severe Head Injury∗
Type of Injury | Incidence (%) |
---|---|
Long-bone or pelvic fracture | 32 |
Maxillary or mandibular fracture | 22 |
Major chest injury | 23 |
Abdominal visceral injury | 7 |
Spinal injury | 2 |
Modified from Miller JD, Sweet RC, Narayan RK, Becker DP. Early insults to the injured brain. JAMA 1978;240:439-442.
Neurological Examination
As soon as the patient’s cardiopulmonary status has been stabilized, a rapid and directed neurological examination is performed (Box 20.2). Although various factors may confound an accurate evaluation of the patient’s neurological state (e.g., hypotension, hypoxia, intoxication, sedation or paralytic agents), valuable data can nevertheless be obtained. If a patient demonstrates variable responses to stimulation, or if the response on each side is different, the best response appears to be a more accurate prognostic indicator than the worst response. To follow trends in an individual patient’s progress, however, it is better to report both the best and the worst responses. In other words, the right-side and left-side motor responses should be recorded separately.
One should not limit the examination to the GCS. Other important data in the initial assessment of patients with impaired consciousness are the patient’s age, vital signs, pupillary response, and eye movements.42 The GCS score provides a simple grading of the arousal and functional capacity of the cerebral cortex, and the pupillary responses and eye movements serve as measures of brainstem function. Advanced age, hypotension, and hypoxia all adversely affect outcome. Indeed, there is considerable interplay between all these factors in determining the ultimate outcome in the severe TBI patient.
Pupils
Careful observation of pupil size and response to light is important during the initial examination (Table 20.3). A well-known early sign of temporal lobe herniation is mild dilatation of the pupil and a sluggish pupillary light response. Compression or distortion of the oculomotor nerve during tentorial-uncal herniation impairs the function of the parasympathetic axons that transmit efferent signals for pupillary constriction, resulting in mild pupillary dilatation. Sometimes, bilateral miotic pupils (1-3 mm) can occur in the early stages of herniation as a result of compromise of the pupillomotor sympathetic pathways originating in the hypothalamus, permitting a predominance of parasympathetic tone and pupillary constriction. In either instance, continued herniation causes increasing dilatation of the pupil and paralysis of its light response. With full mydriasis (8- to 9-mm pupil), ptosis and paresis of the medial rectus and other ocular muscles innervated by the oculomotor nerve appear. A bright light in a darkened room is always necessary to determine pupillary light responses. A magnifying lens such as the +20-diopter lens on a standard ophthalmoscope is helpful in distinguishing between a weak and an absent pupillary light reaction, especially if the pupil is small. A computerized pupillometer underwent clinical testing, but the results were reportedly unclear.43
TABLE 20.3 Interpretation of Pupillary Findings in Patients with Head Injury
Pupillary Size | Light Response | Interpretation |
---|---|---|
Unilaterally dilated | Sluggish or fixed | Cranial nerve III compression secondary to tentorial herniation |
Bilaterally dilated | Sluggish or fixed | Inadequate brain perfusion Bilateral cranial nerve III palsy |
Unilaterally dilated | Cross-reactive (Marcus Gunn) | Optic nerve injury |
Bilaterally miotic | May be difficult to determine | Drugs (opiates) Metabolic encephalopathy Pontine lesion |
Unilaterally miotic | Preserved | Injured sympathetic pathway (e.g., carotid sheath injury) |
Eye Movements
The oculovestibular response can be tested with ice water and only a small expenditure of time. Obstructions within the external auditory canal due to blood or cerumen must be removed, and ocular movement may be limited in patients with orbital edema. In alert patients, cold caloric stimulation causes fast-phase nystagmus in the direction opposite to the tonic eye deviation. The mnemonic “COWS” (cold opposite, warm same) refers to this phenomenon. However, in comatose patients, functional suppression of the reticular activating system is reflected by the absence of nystagmus in response to caloric stimulation so that only the tonic eye deviation is seen (cold same). Thus, irrigation with cold water in a comatose patient causes ipsilateral deviation of the eyes toward the stimulated side.
Motor Function
The basic examination is completed by a gross test of motor strength, although severely head-injured patients are not sufficiently responsive for such a determination to be reliably made. Each extremity is examined and graded on the internationally used scale shown in Table 20.4. Furthermore, stimuli to assess patients should be standardized. In assessing for localization the elbow should be bent at 90 degrees with the forearms resting on the patient’s chest. If the patient is able to bring the hand at or above the chin, localization should be noted. To assess withdraw, deep nailbed pressure to the second digit is done to test for any movement away from the noxious stimulus. Assessing the lower extremities for withdraw is difficult to differentiate from reflexive triple flexion and thus has diminished validity.
Component | Points Assigned |
---|---|
Normal power | 5 |
Moderate weakness | 4 |
Severe weakness (antigravity) | 3 |
Severe weakness (not antigravity) | 2 |
Trace movement | 1 |
No movement | 0 |
Diagnostic Procedures
Computed Tomography
CT scanning is clearly the procedure of choice in the evaluation of the head-injured patient and has probably significantly improved outcome after head injury.44 It is strongly recommended that an emergency CT scan be obtained as soon as possible (preferably within half an hour) after admission with a severe head injury. Centers dealing with a large number of such patients must make arrangements to have CT technicians in the hospital on a 24-hour basis, or within easy accessibility in an emergency. CT scans should also be repeated whenever there is a change in the patient’s clinical status or an unexplained rise in intracranial pressure.
In a prospective study of CT scan abnormalities in 207 patients with severe TBI, we found the initial CT scan to be normal in 30% of cases. The remaining 70% of patients had CT scan abnormalities: low-density lesions in 10%, high-density nonsurgical lesions in 19%, and high-density lesions requiring surgery in 41%.32
Cerebral contusions are seen as nonhomogeneous areas of high density, often interspersed with areas of low density (“salt and pepper” appearance). The CT appearance results from multiple small areas of hemorrhage within the brain substance, associated with areas of edema (see Fig. 20.4D). The margin is usually poorly defined. A mass effect is often seen, although this appearance may be minimal. Depending on the extent of hemorrhage, the degree of edema, and the time course, a contusion may appear predominantly dense or lucent.
Although it is not always possible to differentiate between subdural and epidural hematomas on CT, the latter are typically biconvex or lenticular in shape, because the close attachment of the dura to the inner table of the skull prevents the hematoma from spreading (see Fig. 20.4B). Approximately 20% of patients with an extracerebral hematoma have blood in both the epidural and subdural spaces at operation or autopsy. Because there is little chance of epidural blood mixing with CSF, these lesions appear as uniformly dense collections and are rarely isodense. However, they may develop in a delayed fashion, especially after evacuation of a contralateral “balancing” lesion.
The typical subdural hematoma is more diffuse than an epidural hematoma and has a concave inner margin that follows the surface of the brain (see Fig. 20.4C). The distinction between acute, subacute, and chronic lesions is somewhat arbitrary. However, most acute subdural hematomas are hyperdense, most subacute lesions are isodense or of mixed density, and most chronic hematomas are hypodense as compared with brain tissue. Effacement of the cerebral sulci over the convexity and distortion of the ipsilateral lateral ventricle may suggest the presence of an isodense hematoma.
Overall, a noncontrast CT scan of the brain is a quick and accurate diagnostic tool for initial and serial assessment in TBI patients. Furthermore, studies have shown that CT can also be used as a prognostic tool in TBI.10,45–47 Predictive value can be maximized by taking all protocols into account, using a combination of the group as a tool to help prognosticate patient outcome including at least the following parameters: status of basal cisterns, midline shift, traumatic subarachnoid or intraventricular hemorrhage, and presence of different types of mass lesions.48
Computed Tomography Angiography
Patients who have skull base fractures through the petrous bone, cervical trauma, or cervical fractures through the transverse foramen are at risk for carotid and vertebral artery dissection. CTA offers a rapid means of cervical and intracranial vascular injury assessment while the patient is the emergency department.49–52 Contraindications to CTA use include allergies to iodine-based contrast dye as well underlying renal disease. Most centers have protocols in place to guide specific criteria in regard to dealing with such patients.
Magnetic Resonance Imaging
A wide range of CT findings in patients with closed head injury can represent a specific GCS score. Furthermore, repeat CT scans offer a cumulative risk or iatrogenic pathology because of the radiation used to obtain the images.53 The use of MRI in TBI patients can help in diagnosis, especially in those with nonspecific CT findings. Particularly helpful imaging sequences include diffusion-weighted imaging (DWI), susceptibility-weighted imaging (SWI), diffusion tensor imaging (DTI), MRI spectroscopy, and functional MRI with established protocols.54–56
Intracranial Pressure
Since the early 1970s, there has been an increasing interest in ICP monitoring and control This has been associated with progressive evolution of related technology. However, the intraventricular catheter (or ventriculostomy) remains the most widely used and most useful device for measuring ICP and helping in its control and for maintaining cerebral perfusion pressure.57,58
Cerebral perfusion pressure (CPP) is the mean arterial blood pressure minus ICP. Because cerebral ischemia may be the single most important secondary effect affecting outcome following severe TBI, it is useful to follow CPP rather than ICP alone.59 The guidelines have recommended maintaining CPP at a minimum of 60 mm Hg to possibly help in the avoidance of both global and regional ischemia. Conversely, too great a CPP may also have a deleterious effect, particularly with respiratory complications.
Head injury is the most common indication for ICP monitoring. As a general rule, patients who can follow simple commands need not be monitored and may satisfactorily be followed clinically. In patients who are unable to follow simple commands and have an abnormal CT scan, the incidence of intracranial hypertension is high (53-63%), and monitoring is warranted.38 Severe TBI patients with normal CT scans generally have a lower incidence of elevated ICP (approximately 13%) unless they have two or more of the following adverse features at admission: systolic blood pressure less than 90 mm Hg, unilateral or bilateral motor posturing, or age over 40 years. In the presence of these adverse features, the incidence of intracranial hypertension (even in patients with normal CT scans) is as high as in those with abnormal CT scans on admission.38 Compression or absence of basal cisterns has also been associated with elevated ICP.60
When intracranial pressure demonstrates an upward trend, certain basic items should be checked. The neck should be in a neutral position to facilitate venous drainage. In most cases, having the head end of the bed elevated approximately 30 degrees is useful.61 The calibration of the system must be checked, and one should confirm that the transducer is level with the foramen of Monro. If the patient is fighting the ventilator, he or she should be sedated or chemically paralyzed. If these measures are not adequate, various methods exist to reduce the ICP, including ventricular drainage, mannitol, and hyperventilation.
New technology now allows one to monitor cerebral oxygenation, via the LICOX CMP System. The purpose of this triple-lumen bolt system is to provide additional data including brain tissue oxygenation (PBTO2) and temperature as well as ICP in patients in whom cerebral hypoxia and ischemia are a concern. Mean normal brain tissue oxygen pressure is greater than 30 mm Hg (range 25-50 mm Hg) with ischemia reported at ranges less than 8 to 12 mm Hg and cell death at less than 5 mm Hg.62
Because the duration and severity of cerebral tissue hypoxia correlates with unfavorable outcomes in severe TBI, this could prove a valuable tool in management and possibly in predicting outcome. The monitor is able to indicate cerebral tissue perfusion status local to the sensor placement, although local brain oxygen levels may not reflect what is happening in the rest of the brain. In the recent literature brain tissue oxygenation monitoring and treatment have shown promise in decreasing morbidity and fatality associated with traumatic brain injury.63–65
Guidelines for Treatment
It is difficult to lay down hard and fast rules regarding the management of a disease as diverse as head injury. The primary aim to treatment is to prevent secondary damage to an already injured brain. Nevertheless, in 1995 a document was developed by the Brain Trauma Foundation that established treatment protocols for the management of TBI.3,4 This document was the result of a joint effort between the Brain Trauma Foundation and the American Association of Neurological Surgeons. A panel of expert neurosurgeons with specific interests in the care of patients with severe head injury examined the available data on TBI. Recommendations of care were formulated relying on scientific evidence rather than expert opinion. The recommendations were classified as level I, II, or III, reflecting the degree of clinical certainty. Table 20.5 presents an outline of these recommendations.3,4 The Brain Trauma Foundation updated these guidelines in 2007.9 These updates will be applied to the following treatment recommendations and are included in Table 20.5.
Medical Therapy
Blood Pressure and Oxygenation
A significant portion of brain injury patients have hypotension and hypoxia in the prehospital and hospital setting. These confounding factors increase the resultant secondary brain injury. Presently the exact level of hypotension that is detrimental to outcome is unknown; however, systolic blood pressure (SBP) less than 90 mm Hg is the currently used definition of hypotension. The level of hypoxia is similarly unknown; however, the definition is apnea cyanosis in the field or a PaO2 less than 60 mm Hg. Clinical studies have yet to show a decrease in morbidity and mortality rates in TBI patients when these factors are corrected. Currently there are insufficient data to support level I recommendations. There is level II evidence for monitoring blood pressure and avoiding hypotension defined as SBP less than 90 mm Hg.9 Level III evidence exists for oxygenation monitoring and avoidance of hypoxia (PaO2 <60 mm Hg or O2 saturation <90%).9
Hyperosmolar Therapy
Mannitol’s physiological effect relies on its ability to expand plasma, reduce hematocrit, and increase deformability of erythrocytes, reducing blood viscosity and increasing cerebral oxygenation delivery. Mannitol’s effect takes place between 15 and 30 minutes while plasma gradients are established. Mannitol should be used with caution in patients with renal issues or on nephrotoxic drugs as serum osmolality rises and patients are at risk for acute tubular necrosis. Serum osmolality should not generally be allowed to go much above 320 mOsm/L, if possible, to avoid systemic acidosis and renal failure. There is clinical and laboratory evidence to suggest that long-term, repeated use of mannitol can worsen brain edema and hence reverse the initial beneficial effect.27 Mannitol has been shown to have an effect on ICP lasting from 90 minutes to 6 hours or more.
Recently studies on small volume resuscitations have shown the therapeutic effectiveness of hypertonic saline (HS) administration. Hypertonic saline solutions were administered in poly-trauma patients with hemorrhagic shock, and the subset of patients in the group with TBI showed an increase in survival and stabilization of hemodynamic properties.9 As a result of this finding, other studies have been conducted showing its utility in the treatment of elevated ICP in trauma, subarachnoid hemorrhage, stroke, and other pathological insults.
Current Brain Trauma Foundation guidelines show level II evidence for mannitol’s effective control of raised ICP at doses between 0.25 mg/kg to 1 g/kg of body weight.9 Level II evidence exists to avoid hypotension (SBP <90 mm Hg).9 Recent studies of HS show its utility in a continuous infusion form or as bolus treatment form equivalent to or superior to mannitol for refractory elevated ICP.66–69 More studies need to be performed to evaluate mannitol versus HS with respect to clinical outcome after TBI.
Prophylactic Hypothermia
The utility of hypothermia in TBI has inconsistent results in the medical literature. During the BTF review of the literature mortality rate was affected only by the duration of cooling, which meant a decrease in mortality rate if cooling was for more than 48 hours.9 Target cooling temperatures or rate of rewarming had no effect on outcome. The pathophysiological theory behind this treatment is that ICP can be decreased by decreasing brain metabolic demand.
Interpretation of trials involving hypothermia is difficult secondary to confounding factors affecting outcome. It appears that patients who are treated with hypothermia have a trend to more favorable outcome, Glasgow Outcome Scale scores of 4 to 5. Currently there are no level I or level II recommendations for this treatment. A Cochrane Database review in 2009 found no evidence that hypothermia is beneficial in the treatment of head injury. Hypothermia may be effective in reducing death and unfavorable outcomes for traumatic head-injured patients, but significant benefit was only found in low-quality trials.9 High-quality trials found no decrease in the likelihood of death with hypothermia, but this finding was not statistically significant and could be due to the play of chance.9 Hypothermia should not be used except in the context of a high-quality randomized controlled trial with good allocation concealment.9
Infection Prophylaxis
In polytrauma patients the risk of infection can be increased significantly secondary to intubation, invasive lines, and placement of intracranial monitors. Infections can be broken down into infection/colonization of ICP monitors and infection/colonization of peripheral lines/pneumonia. Most trials of prophylactic antibiotics have shown selection of more virulent gram-negative organisms. Currently BTF guidelines recommend at a level II the use of periprocedural antibiotics for intubation to reduce the incidence of pneumonia.9 This does not change length of stay or mortality rates. Early tracheostomy reduces mechanical ventilation days, but it does not alter mortality rate or nosocomial pneumonia rate. There is level III evidence against the routine use of prophylactic antibiotics for ventricular catheter placement.9 There is currently level III evidence against the routine use of ventricular catheter exchange to prevent infection.9
Deep Venous Thrombosis Prophylaxis
The risk of developing deep venous thrombosis (DVT) in the absence of prophylaxis was found to be 20% after severe TBI in a study by Kaufman and associates.70 This risk is related to systemic trauma as well as the relative period of immobility these patients face. DVTs of the distal lower extremity veins tend to be clinically silent and tend to stay that. Proximal lower extremity DVTs are more likely to produce symptoms clinically and result in pulmonary embolism (PE). The treatment of DVT and PE in the TBI patient is complicated by the uncertainty of the safety of anticoagulation, specifically in postcraniotomy patients or those with intracerebral hemorrhage from their trauma.
In studies comparing pharmacological versus mechanical treatment for the prevention of DVT, pharmacological treatment is more efficacious in preventing DVT but there is a trend toward increased risk of intracranial bleeding.9 There are no current recommendations as to the timing or optimal dosing of pharmacological prophylaxis in neurosurgical patients with the current evidence.
Current BTF guidelines show level III evidence for graduated compression stockings or intermittent pneumatic compression stockings unless lower extremity injury prevents their use.9 Low-molecular-weight heparin or low-dose unfractionated heparin should be used in combination with mechanical prophylaxis, but there is an increased risk for expansion of intracranial hemorrhage. There are currently no timing recommendations for the initiation of pharmacological DVT prophylaxis.
Intracranial Pressure Monitoring
Monitoring ICP can be used to monitor patients for worsening intracranial injury, help predict outcome, calculate CPP, and if ventriculostomy is used, therapeutic CSF drainage. When comparing patients with ICP monitoring to prior reports of patients without monitoring, monitored patients have improved outcome.9
Currently BTF guidelines show level II evidence for placing an ICP monitor in all salvageable patients with severe TBI defined by a postresuscitation GCS score of 3 to 8 and an abnormal CT scan of the head.9 An abnormal CT of the head is defined as one showing hematomas, contusions, swelling, herniation, or compressed basal cisterns.9 Current level III evidence exists for ICP monitoring in patients with severe TBI with a normal CT and two or more of the following: (1) age over 40 years, (2) unilateral or bilateral motor posturing, or (3) systolic blood pressure less than 90 mm Hg.38 These three factors were found to have higher incidence of elevated ICP in patients with normal admission CT scan.
Intracranial Pressure Monitor Technology
As stated in the previous section different types of intracranial monitors exist. The ideal monitor should have several useful qualities including ease of insertion, safety of insertion, accuracy, reliability, cost effectiveness, and the need for minimal troubleshooting. The Association for the Advancement of Medical Instrumentation (AAMI) was developed in association with a neurosurgery committee. This association led to the development of the American National Standard for Intracranial Pressure Monitoring Devices. The job of this standard is to provide labeling, safety, and performance requirements of intracranial monitors. According to the AAMI standard, ICP monitors should have (1) pressure ranges of 0 to 100 mm Hg, (2) accuracy ±2 mm Hg in the range of 0 to 20 mm Hg, and (3) maximum error of 10% in the range of 20 to 100 mm Hg.9 The current ICP monitors work via external strain, catheter tip strain, or catheter tip fiberoptics. Catheter tip fiberoptics are calibrated prior to intracranial insertion and are at risk for measurement drift and possible inaccurate readings.
Current available ICP monitors were ranked based on accuracy, reliability, and cost. The order of ranking is as follows: (1) intraventricular devices (fluid-coupled catheter), (2) intraventricular devices (microstrain gauge or fiberoptic), (3) parenchymal pressure transducers, (4) subdural devices, (5) subarachnoid fluid couple devices, and (6) epidural devices.9
The ventricular catheter connected to an external strain gauge remains the most cost effective and reliable method of ICP monitoring.9 However, solid state monitors such as the Camino or Codman systems are generally reliable, although they cannot be recalibrated once they have been inserted.
Treatment Thresholds and Optimal Cerebral Perfusion Pressure
The threshold to treat elevated ICP should be based on the patient’s CT scan, clinical picture, and ICP number. Multiple small studies show the optimal treatment window to be between 15 and 25 mm Hg. It is important to emphasize that patients with temporal hematomas or posterior fossa hemorrhages may have deceptively low ICP despite being under neurological duress. Current level II evidence recommends treating patients with ICP greater than 20 mm Hg with ICP lowering measures.9
The management paradigm of elevated CPP (>70 mm Hg) for treatment of TBI became popular in the late 1980s. It has since been found that the optimal CPP ranges between 50 and 70 mm Hg.9 Currently level II evidence recommends keeping CPP less than 70 mm Hg because systemic complications are increased when the CPP is elevated above this level. Current level III evidence suggests a CPP less than 50 mm Hg should be avoided; the optimal range is somewhere between 50 and 70 mm Hg.9 Ancillary monitoring of blood flow, oxygenation, or metabolism helps to facilitate on a patient-by-patient basis the optimal CPP.
Brain Oxygenation Monitoring and Threshold for Treatment
Multiple studies have shown that a diminished oxygenation of brain tissue (as measured by the partial pressure of oxygen in brain tissue, PBTO2) is correlated with poorer outcomes and death.63–65 Treatments to keep PBTO2 greater than 25 mm Hg along with CPP and ICP management have shown decreased mortality rates when compared to outcomes of treating CPP and ICP alone.63–65
Even though treatments to maintain cerebral oxygenation seem promising there is only level III evidence for jugular venous monitoring and brain tissue oxygen tension monitoring.9 Treatment thresholds are less than 50% for jugular venous saturation and more than 15 mm Hg for brain tissue oxygenation.9
Anesthesia, Analgesics, and Sedatives
It has been long understood that pain and agitation may raise ICP in the TBI patient. Pain medications and sedatives have often been used to calm patients to prevent a dangerous rise in ICP that may be associated with severe agitation. Barbiturates have been used since the 1930s with the knowledge of their ability to lower ICP.71 Barbiturates are also known to decrease cerebral metabolism having a cerebral protective effect.72,73 The use of barbiturates couples blood flow to cerebral metabolism, decreasing blood flow where metabolism is low and shunting blood flow to areas where metabolism is high.
Propofol has more recently been studied because of its quick onset of action, and its short duration of action allows quick neurological assessment. Studies have shown that propofol does have a minimal effect at lowering ICP, and one study reported high-dose propofol showing a favorable neurological outcome when compared to a low-dose propofol.74 Propofol must be used with caution, especially in high doses, as some patients develop propofol infusion syndrome, which may lead to death.
BTF guidelines currently have level II evidence that prophylactic administration of barbiturates to burst suppression is not recommended. High-dose barbiturate treatment is recommended for refractory ICP control; however, hemodynamic stability must be maintained and improved outcomes have never been proved.9 Propofol is recommended for the control of ICP, but not for improvement of 6-month outcomes.9
Nutrition
Current level II evidence recommends that patients should be fed full caloric replacement by 7 days after injury.9
Antiseizure Prophylaxis
The role of prophylactic anticonvulsants in patients with severe head injury has been more clearly defined with the advent of the published guidelines. Post-traumatic seizures are classified as “early,” occurring within 7 days of injury, or “late,” occurring more than 7 days after the injury.75,76 It is desirable to prevent early and late seizure activity, although these medications have been associated with adverse and neurobehavioral ill effects. The classic study by Jennett77 found post-traumatic epilepsy to occur in about 5% of all patients admitted to the hospital with closed head injuries and in 15% of those with severe head injuries. Three main factors were found to be linked to a high incidence of late epilepsy: early seizures occurring within the first week, an intracranial hematoma, or a depressed skull fracture. Recent updates show that post-traumatic seizures are also associated with GCS scores less than 10, penetrating head wound, or a seizure within the first 24 hours. Although certain earlier studies were unable to show significant benefit of prophylactically administered anticonvulsants, a double-blind study of 404 patients with severe head injury, who were randomized to receive phenytoin or placebo beginning within 24 hours of injury and continuing for 1 year, found that phenytoin reduced the incidence of seizures in the first week after injury but not thereafter.78 This study appears to justify stopping prophylactic convulsants after the first week in most cases. In patients who have had a seizure, anticonvulsants are continued for at least a year.
With the recent use of Keppra (levetiracetam) as an antiepileptic, with its relatively low side effect profile, studies have been done to evaluate its efficacy compared to the gold standard Dilantin (phenytoin). It does appear to be as effective as Dilantin at preventing early seizures with a lower side effect profile.79,80 Larger scale studies will need to be done to confirm this finding. It should also be noted the Keppra had a tendency to show more seizure activity on EEG analysis in one study.79
Current BTF recommendations show level II evidence that Dilantin and valproate are not indicated to prevent late onset seizure activity.9 Level II evidence shows that antiepileptic medication is indicated to prevent early onset seizure (within 7 days); however, early onset post-traumatic seizure have not been proved to be associated with worse outcomes.9
Hyperventilation
Hyperventilation has been used in the treatment of severe TBI for the past 20 years. Recent pathophysiological understanding of TBI and the mechanism of ICP lowering of hyperventilation have put it under recent scrutiny. Hyperventilation decreases ICP by decreasing CO2 leading to cerebral vasoconstriction. This in turn leads to decreased cerebral blood flow (CBF). Aggressive sustained hyperventilation may lead to cerebral ischemia and stroke, especially in the severe TBI patient who may already have alterations in CBF and autoregulation.
Current BTF recommendations show level II evidence against the routine use of prophylactic hyperventilation to PaCO2 less than 25 mm Hg.9 Level III evidence exists that hyperventilation may be used as a temporizing measure in the acutely deteriorating patient.9 Hyperventilation should be avoided in the first 24 hours after TBI when CBF may be critically reduced. If hyperventilation is used, there is level III evidence for jugular venous oxygen monitoring or brain tissue oxygen tension monitoring.9
Surgical Therapy
Indications for Surgery
Our policy is to operate on all comatose patients with an intracranial mass lesion and 5 mm or more of midline shift unless they are brain-dead. This policy is based on evidence that some patients with bilaterally nonreactive pupils, impaired oculocephalic responses, and decerebrate posturing can nevertheless make a good recovery. In one series, 3 of 19 such patients who were treated maximally ended up in the “good” or “moderately disabled” category, despite the foreboding constellation of signs.81
The management of brain contusions is somewhat less clear-cut. Galbraith and Teasdale,82 in their series of 26 patients with acute traumatic intracranial hematomas who were managed without surgery, found that all patients with ICP greater than 30 mm Hg eventually deteriorated and required surgery. In contrast, only one patient with ICP less than 20 mm Hg deteriorated. Patients in the 20 to 30 mm Hg range were about evenly divided between the surgical and nonsurgical groups.
We have recently analyzed our experience with 130 head-injured patients with pure contusions who were managed with CT scanning and ICP monitoring as needed.83 This study showed that patients with brain contusions who could follow commands at admission did not require ICP monitoring and, as a rule, did well with simple observation. However, those who could not follow commands (in the absence of a focal lesion in the speech area) often had intracranial hypertension and needed to have their ICP monitored. The majority of these patients who had a midline shift of 5 mm or more required surgery.
It has been demonstrated conclusively that patients with a large (over 30 mL) temporal lobe hematoma have a much greater risk of developing tentorial herniation than those with a frontal or parieto-occipital lesion.84 The bias should therefore tilt toward early surgery in such cases.
Once a decision has been made as to whether the patient is a surgical candidate or not, he or she is promptly moved to the operating room or to the neurosurgical intensive care unit (NICU), respectively. If the patient is harboring a mass lesion, mannitol (1-2 g/kg) should be administered en route to the operating room. In addition, the patient can be hyperventilated briefly to achieve an arterial PCO2 of 25 to 30 mm Hg. As in all the maneuvers undertaken thus far, time is of the essence. The sooner the mass lesion is evacuated, the better the possibility of a good recovery.24 If, on the other hand, no surgical lesion is found, the patient is carefully monitored in the NICU, both clinically and with various physiological parameters, notably ICP recordings and serial CT scans. Any rise in ICP above 20 mm Hg that cannot be readily explained and reversed or any deterioration in neurological status warrants prompt repetition of the CT scan followed by appropriate corrective measures.
Subdural Hematomas
Acute subdural hematomas may result from bleeding from lacerated brain, ruptured cortical vessels, or an avulsed bridging vein. The most common sites for brain injury are the inferior frontal lobes and the anterior temporal lobes. In the surgical management of subdural hematomas, a large fronto-temporoparietal question mark–shaped incision is recommended. This allows the surgeon to deal with bleeding near the midline as well as to débride effectively parts of the frontal, temporal, and parietal lobes as needed. If the patient is deteriorating rapidly, a quick temporal decompression can be performed via a small craniectomy before opening up the rest of the flap. This maneuver could reduce the probability of tentorial herniation. A generous subtemporal craniectomy may be useful in postoperative ICP control. The role of decompressive craniotomy with removal of the bone flap and creation of a dural pouch to allow brain swelling is currently being examined.
Contusions/Intracerebral Hematomas
Contusions are most often located in the anterior and inferior frontal lobes as well as the anterior temporal lobes. Quite commonly, the CT appearance of a contusion evolves over several days so that what are initially small “salt and pepper” lesions coalesce to form hematomas. This phenomenon is also termed delayed traumatic intracerebral hematoma. Patients who are awake and alert but demonstrate cerebral contusions can be managed without surgery in the vast majority of cases.83 However, patients who are comatose and have a significant midline shift usually need surgery. Between these two extremes, there are patients who demonstrate alterations in levels of consciousness or focal neurological deficits; in these, the decision to undertake surgical débridement is not always easy. As a general rule, débridement of the left frontal and temporal lobes is undertaken more reluctantly because the speech area is on this side.
Prognosis
The Glasgow Outcome Scale (GOS) has been widely accepted as a standard means of describing outcome in head injury patients. This is a simple five-point scale (Table 20.6).85 These categories are sometimes lumped together as either favorable outcomes (G, MD) or unfavorable outcomes (SD, V, or D). Post-traumatic amnesia is a fairly good prognostic indicator of outcome. First described by Russel in 1932, post-traumatic amnesia is defined as the duration of time from the point of injury until the patient has continuous memory of ongoing events. In most cases, the retrospective measurement of post-traumatic amnesia is unreliable. Therefore, Harvey Levin developed the Galveston Orientation and Amnesia Test (GOAT) to provide an objective reliable measurement of post-traumatic amnesia. The duration of post-traumatic amnesia has proved to be highly correlated with ultimate functional outcomes.86
Good recovery (G) | Patient returns to preinjury level of function |
Moderately disabled (MD) | Patient has neurological deficits but is able to look after self |
Severely disabled (SD) | Patient is unable to look after self |
Vegetative (V) | No evidence of higher mental function |
Dead (D) |
Several statistical studies have reported the use of various prognostic indicators for predicting outcome in severe head injury. Because of unexpected medical and surgical complications and the inherent unpredictability of disease, there is no absolutely unfailing prediction system. Based on experience with a large group of patients, an algorithm has been developed for approximate expected outcomes associated with certain prognostic features.87 An attempt to predict mortality with 100% certainty appeared to work in one center.88 However, when this system was applied to other patient populations, some patients who were predicted to die based on this scale instead survived.89
Bullock M.R., Chestnut R., Ghajar J., et al. Surgical management of TBI author group. Neurosurgery. 2006;58(suppl 3):S1-S62.
Bullock R., Chestnut R.M., Clifton G., et al. Guidelines for the management of severe head injury. J Neurotrauma. 2000;17:449-627.
Chestnut R.M., Ghajar J., Maas A.I.L., et al. Early indicators of prognosis in severe traumatic brain injury. J Neurotrauma. 2000;17:557-590.
Feldman Z., Narayan R.K. Intracranial pressure monitoring: techniques and pitfalls. In: Cooper P.R., editor. Head Injury. 3rd ed. Philadelphia: Williams & Wilkins; 1993:247-274.
Oddo M., Levine J.M., Frangos S., et al. Effect of mannitol and hyptertonic saline on cerebral oxygenation in patients with severe traumatic brain injury and refractory intracranial hypertension. J Neurol Neurosurg Psychiatry. 2009;80(8):916-920.
Temkin N.R., Dikmen S.S., Winn H.R. Posttraumatic seizures. Neurosurg Clin North Am. 1991;2:425-435.
Please go to expertconsult.com to view the complete list of references.
1. Thurman D., Guerrero J. Trends in hospitalization associated with traumatic brain injury. JAMA. 1999;282:954-957.
2. Lu J., Marmarou A., Choi S., et al. Mortality from traumatic brain injury. Acta Neurochir. 2005;95(suppl):281-285.
3. Bullock R., Chestnut R.M., Clifton G., et al. Guidelines for the management of severe head injury. J Neurotrauma. 1996;13:641-734.
4. Bullock R., Chestnut R.M., Clifton G., et al. Guidelines for the management of severe head injury. J Neurotrauma. 2000;17:449-627.
5. Alderson P., Roberts I. Corticosteroids for acute traumatic brain injury. Cochrane Database Syst Rev. 2000;2:CD000196.
6. Roberts I. Barbiturates for acute traumatic brain injury. Cochrane Database Syst Rev. 2000;2:CD000033.
7. Schierhout G., Roberts I. Mannitol for acute traumatic brain injury. Cochrane Database Syst Rev. 2000;2:CD001049.
8. Guidelines for the management of penetrating brain injury. J Trauma. 2001;51:S3-S15.
9. Bullock M.R., Povlishock J.T. Guidelines for the management of severe traumatic brain injury. J Neurotrauma. 2007;24(suppl 1):S1-106.
10. Bullock M.R., Chestnut R., Ghajar J., et al. Surgical management of TBI author group. Neurosurgery. 2006;58(suppl 3):S1-S62.
11. Chestnut R., Ghajar J., Marion D.W., et al. Early indicators of prognosis in severe traumatic brain injury. Brain Trauma Foundation. 2000:1-103.
12. Narayan R.K., Michel M.E., Ansell B., et al. Clinical trials in head injury. J Neurotrauma. 2002;19:503-557.
13. Teasdale G., Jennett B. Assessment of coma and impaired consciousness. Lancet. 1974;2:81-84.
14. Jennett B., Teasdale G. Assessment of impaired consciousness. In: Plum F., editor. Management of Head Injuries. Philadelphia: FA Davis; 1981:77-93.
15. Miller J.D. Minor, moderate and severe head injury. Neurosurg Rev. 1986;9:135-139.
16. Williams D.H., Levin H.S., Eisenberg H.M. Mild head injury classification. Neurosurgery. 1990;27:422-428.
17. Rodriguez E.D., Stanwix M.G., Nam A.J., et al. Twenty-six year experience treating frontal sinus fractures: a novel algorithm based on anatomical fracture pattern and failure of conventional techniques. Plast Reconstr Surg. 2008;122(6):1850-1866.
18. Stanwix M.G., Nam A.J., Manson P.N., et al. Critical computed tomographic diagnostic criteria for frontal sinus fractures. J Oral Maxillofac Surg. 2010;68(11):2714-2722.
19. Cordobes F., Lobato R., Rivas J., et al. Observations on 82 patients with extradural hematoma: comparison of results before and after the advent of computerized tomography. J Neurosurg. 1981;54:179-186.
20. Gupta S., Tandon S., Mohanty S., et al. Bilateral traumatic extradural haematomas: report of 12 cases with a review of the literature. Clin Neurol Neurosurg. 1992;94:127-131.
21. Maggi G., Aliberti F., Petrone G., Ruggiero C. Extradural hematomas in children. J Neurosurg Sci. 1998;42:95-99.
22. Wu J., Hsu C., Liao S., Wong Y. Surgical outcome of traumatic intracranial hematoma at a regional hospital in Taiwan. J Trauma. 1999;47:39-43.
23. Howard M.A.3rd, Gross A.S., Dacey R.J.Jr., Winn H.R. Acute subdural hematomas: an age-dependent clinical entity. J Neurosurg. 1989;71:858-863.
24. Seelig J.M., Becker D.P., Miller J.D., et al. Traumatic acute subdural hematoma: major mortality reduction in comatose patients treated within four hours. JAMA. 1981;304:1511-1518.
25. Mandera M., Zralek C., Krawcyzk I., et al. Surgery or conservative treatment in children with traumatic intracerebral haematoma. Childs Nerv Syst. 1999;15(26):267-269.
26. Gennarelli T.A. Cerebral concussion and diffuse brain injuries. In: Cooper P.R., editor. Head Injury. 2nd ed. Baltimore: Williams & Wilkins; 1987:108-124.
27. Dacey R.G., Alves W.M., Rimel R.W., et al. Neurosurgical complications after apparently minor head injury—assessment of risk in a series of 610 patients. J Neurosurg. 1986;65:203-210.
28. Vos P.E., Battistin L., Birbamer G., et al. EFNS guideline on mild traumatic brain injury: report of an EFNS task force. Euro J Neurol. 2002;9:207-219.
29. Borczuk P. Predictors of intracranial injury in patients with mild head trauma. Ann Emerg Med. 1995;25:731-736.
30. Hofman P.A., Nelelemans P., Kemerink G.J., Wilmink J.T. Value of radiological diagnosis of skull fracture in management of mild head injury: meta-analysis. J Neurol Neurosurg Psychiatr. 2000;68:416-422.
31. Stein S.C., Ross S.E. Moderate head injury: a guide to initial management. J Neurosurg. 1992;77:562-564.
32. Stone J.L., Lowe R.J., Jonasson O., et al. Acute subdural hematoma: direct admission to a trauma center yields improved results. J Trauma. 1986;26:445-450.
33. Miller J.D., Butterworth J.F., Gudeman S.K., et al. Further experience in the management of severe head injury. J Neurosurg. 1981;54:289-299.
34. Levine J.E., Becker D.P. Reversal of incipient brain death from head injury apnea at the scene of accidents. N Engl J Med. 1979;301:109.
35. Chestnut RM, Marshall LF, Klauber MR, et al. Analysis of the role of secondary brain injury in determining outcome from severe head injury. Presented at the Annual Meeting of the American Association of Neurological Surgeons, Nashville, TN, 1990.
36. Butterworth J.F., Maull K.I., Miller J.D., et al. Detection of occult abdominal trauma in patients with severe head injuries. Lancet. 1980;2:759-762.
37. Miller J.D., Sweet R.C., Narayan R.K., Becker D.P. Early insults to the injured brain. JAMA. 1978;240:439-442.
38. Narayan R.K., Kishore P.R.S., Becker D.P., et al. Intracranial pressure: to monitor or not to monitor? A review of our experience with severe head injury. J Neurosurg. 1982;56:650-659.
39. Chestnut R.M., Ghajar J., Maas A.I.L., et al. Early indicators of prognosis in severe traumatic brain injury. J Neurotrauma. 2000;17:557-590.
40. American College of Surgeons Committee on Trauma. Resources for Optimal Care of the Injured Patient. Chicago: American College of Surgeons; 1993.
41. Schenarts P.J., Diaz J., Kaiser C., et al. Prospective comparison of admission computed tomographic scan and plain films of the upper cervical spine in trauma patients with altered mental status. J Trauma. 2001;51:663-668.
42. Narayan R.K., Greenberg R.P., Miller J.D., et al. Improved confidence of outcome prediction in severe head injury: a comparative analysis of the clinical examination, MEPs, CT scanning and ICP. J Neurosurg. 1981;54:751-762.
43. Taylor W.R., Chen J.W., Meltzer H., et al. Quantitative pupillometry, a new technology: normative data and preliminary observations in patients with acute head injury. Technical note. J Neurosurg. 2003;98:205-213.
44. Wester K., Aas-Aune G., Skretting P., Syversen A. Management of acute head injuries in a Norwegian county: effects of introducing CT scanning in a local hospital. J Trauma. 1989;29:238-241.
45. Hukkelhoven C.W., Steyerberg E.W., Habbema J.D., et al. Predicting outcome after traumatic brain injury: development and validation of a prognostic score based on admission characteristics. J Neurotrauma. 2005;22(10):1025-1039.
46. Lobato R.D., Cordobes F., Rivas J.J., et al. Outcome from severe head injury related to the type of intracranial lesion. A computerized tomography study. J Neurosurg. 1983;59(5):762-774.
47. Servadei F., Murray G.D., Penny K. The value of the worst computed tomographic scan in clinical studies of moderate and severe head injury. Neurosurgery. 2000;46:70-77.
48. Maas A.I., Hukkelhoven C.W., Marshall L.F., Steyerberg E.W. Prediction of outcome in traumatic brain injury with computed tomographic characteristics: a comparison between the computed tomographic classification and combinations of computed tomographic predictors. Neurosurgery. 2005;57(6):1173-1182.
49. Sliker C.W. Blunt cerebrovascular injuries: imaging with multidetector CT angiography. Radiographics. 2008;28(6):1689-1708. discussion 1709–1710
50. Wei C.W., Montanera W., Selchen D., et al. Blunt cerebrovascular injuries: diagnosis and management outcomes. Can J Neurol Sci. 2010;37(5):574-579.
51. Goodwin R.B., Berry P.R.2nd, Dorbish R.J., et al. Computed tomographic angiography versus conventional angiography for the diagnosis of blunt cerebrovascular injury in trauma patients. J Trauma. 2009;67(5):1046-1050.
52. Biffl W.L., Ray C.E.Jr., Moore E.E., et al. Noninvasive diagnosis of blunt cerebrovascular injuries: a preliminary report. J Trauma. 2002;53(5):850-856.
53. Brenner D.J., Hall E.J. Computed tomography—an increasing source of radiation exposure. N Engl J Med. 2007;357(22):2277-2284.
54. Sidaros A., Engberg A.W., Sidaros K., et al. Diffusion tensor imaging during recovery from severe traumatic brain injury and relation to clinical outcome: a longitudinal study. Brain. 2008;131(Pt 2):559-572.
55. Ashwal S., Babikian T., Gardner-Nichols J., et al. Susceptibility-weighted imaging and proton magnetic resonance spectroscopy in assessment of outcome after pediatric traumatic brain injury. Arch Phys Med Rehabil. 2006;87(12 suppl 2):S50-S58.
56. Perbarg V., Puybasset L., Tollard E., et al. Relation between brain lesion location and clinical outcome in patients with severe traumatic brain injury: a diffusion tensor imaging study using voxel-based approaches. Hum Brain Mapp. 2009;30(12):3924-3933.
57. Feldman Z., Narayan R.K. Intracranial pressure monitoring: techniques and pitfalls. In: Cooper P.R., editor. Head Injury. 3rd ed. Philadelphia: Williams & Wilkins; 1993:247-274.
58. Rosner M.J., Daughton S. Cerebral perfusion pressure management in head injury. J Trauma. 1990;30:933-941.
59. Miller J.D. Head injury and brain ischemia—implications for therapy. Br J Anesth. 1995;57:120-130.
60. Marshall L.F., Marshall S.B., Klauber M.R., et al. A new classification of head injury based on computerized tomography. J Neurosurg. 1991;75(suppl):S14-S20.
61. Feldman Z., Kanter M.J., Robertson C.S., et al. Effect of head elevation on intracranial pressure, cerebral perfusion pressure and cerebral blood flow in head-injured patients. J Neurosurg. 1992;76:207-211.
62. Van Santbrink H., Maas A.I., Avezaat C.J. Continuous monitoring of partial pressure of brain tissue oxygen in patients with severe head injury. Neurosurgery. 1996;38:21-31.
63. Narotam P.K., Morrison J.F., Nathoo N. Brain tissue oxygen monitoring in traumatic brain injury and major trauma: outcome analysis of a brain tissue oxygen-directed therapy. J Neurosurg. 2009;111(4):672-682.
64. Spiotta A.M., Stiefel Mf, Gracias V.H., et al. Brain tissue oxygen-directed management and outcome in patients with severe traumatic brain injury. J Neurosurg. 2010;113(3):571-580.
65. Stiefel M.F., Spiotta A., Gracias V.H., et al. Reduced mortality rate in patients with severe traumatic brain injury treated with brain tissue oxygen monitoring. J Neurosurg. 2005;103(5):805-811.
66. Kerwin A.J., Schinco M.A., Tepas J.J.3rd, et al. The use of 23.4% hypertonic saline for the management of elevated intracranial pressure in patients with severe traumatic brain injury: a pilot study. J Trauma. 2009;67(2):277-282.
67. Ware M.L., Nemani V.M., Meeker M., et al. Effects of 23.4% sodium chloride solution in reducing intracranial pressure in patients with traumatic brain injury: a preliminary study. Neurosurgery. 2005;57(4):727-736.
68. Oddo M., Levine J.M., Frangos S., et al. Effect of mannitol and hyptertonic saline on cerebral oxygenation in patients with severe traumatic brain injury and refractory intracranial hypertension. J Neurol Neurosurg Psychiatry. 2009;80(8):916-920.
69. Ogden A.T., Mayer S.A., Connolly E.S.Jr. Hyperosmolar agents in neurosurgical practice: the evolving role of hypertonic saline. Neurosurgery. 2005;57(2):207-215.
70. Kaufman H.H., Satterwhite T., McConnell B.J., et al. Deep vein thrombosis and pulmonary embolism in head-injured patients. Angiology. 1983;34:627-638.
71. Eisenberg H.M., Frankowski R.F., Contant C.F., et al. High-dose barbiturates control elevated intracranial pressure in patients with severe head injury. J Neurosurg. 1988;69:15-23.
72. Demopoulous H.B., Flamm E.S., Pietronigro D.D., et al. The free radical pathology and the microcirculation in the major central nervous system trauma. Acta Physiol Scand Suppl. 1980;292:91-119.
73. Goodman J.C., Valadka A.B., Gopinath S.P., et al. Lactate and excitatory amino acids measured by microdialysis are decreased by pentobarbital coma in head-injured patients. J Neurotrauma. 1996;13:549-556.
74. Kelly P.F., Goodale D.B., Williams J., et al. Propofol in the treatment of moderate and severe head injury: a randomized, prospective double-blinded pilot trial. J Neurosurg. 1999;90:1042-1057.
75. Temkin N.R., Dikmen S.S., Winn H.R. Posttraumatic seizures. Neurosurg Clin North Am. 1991;2:425-435.
76. Yablon S.A. Posttraumatic seizures. Arch Phys Med Rehabil. 1993;74:983-1001.
77. Jennett B. Epilepsy after Nonmissile Head Injuries, 2nd ed, London: Heinemann, 1975.
78. Temkin N.R., Dikmen S.S., Wilensky A.J., et al. A randomized, double-blind study of phenytoin for the prevention of post-traumatic seizures. N Engl J Med. 1990;323:497-502.
79. Jones K.E., Puccio A.M., Harshman K.J., et al. Levetiracetam versus phenytoin for seizure prophylaxis in severe traumatic brain injury. Neurosurg Focus. 2008;25(4):E3.
80. Szaflarski J.P., Sangha K.S., Lindsell C.J., Shutter L.A. Prospective, randomized, single-blinded comparative trial of intravenous levetiracetam versus phenytoin for seizure prophylaxis. Neurocrit Care. 2010;12(2):165-172.
81. Becker D.P., Miller J.D., Ward J.D., et al. The outcome from severe head injury with early diagnosis and intensive management. J Neurosurg. 1977;47:491-502.
82. Galbraith S., Teasdale G. Predicting the need for operation in the patient with an occult traumatic intracranial hematoma. J Neurosurg. 1981;55:75-81.
83. Sheinberg M.A., Kanter M.J., Robertson C.S., et al. Continuous monitoring of jugular venous oxygen saturation in head-injured patients. J Neurosurg. 1992;76:212-217.
84. Andrews B.T., Chiles B.W., Olsen W.L., Pitts L.H. The effect of intracerebral hematoma location on the risk of brain-stem compression and on clinical outcome. J Neurosurg. 1988;69:518-522.
85. Jennett B., Bond M. Assessment of outcome after severe brain damage: a practical scale. Lancet. 1975;1:480-484.
86. Ellenberg J.H., Levin H.S., Saydjari C. Posttraumatic amnesia as a predictor of outcome after severe closed head injury. Prospective assessment. Arch Neurol. 1996;53(8):782-791.
87. Choi S.C., Muizelaar J.P., Barnes T.Y., Young H.F. Prediction tree for severely head injured patients. J Neurosurg. 1991;75:251-255.
88. Gibson R.M., Stephenson G.C. Aggressive management of severe closed head trauma: time for reappraisal. Lancet. 1989;2(8659):369-371.
89. Feldman Z., Contant C.F., Robertson C.S., et al. Evaluation of the Leeds prognostic score for severe head injury. Lancet. 1991;337:1451-1453.