CHAPTER 34 Clinical Evaluation of Adult Hydrocephalus
Classification and Etiology
The term hydrocephalus is derived from the Greek: hydro meaning water and kefale meaning skull. Its classification still provokes controversy and often reflects outmoded methods of investigation. Commonly, hydrocephalus indicates dilated ventricles and hence increased volume of intracranial cerebrospinal fluid (CSF). However, this definition does not exclude cerebral atrophy caused by various neurodegenerative disorders (“hydrocephalus ex vacuo”). Hence, it is preferable to define hydrocephalus as the state of excessive intracranial accumulation of CSF that results from excessive production, circulation, or absorption of CSF. It is frequently, but not always characterized by ventriculomegaly. Similarly, the presence of excess CSF in the subarachnoid or subdural spaces over the brain convexity may or may not be a result of true hydrocephalus. Hydrocephalus is the consequence of active secretion of CSF by the choroid plexus—active CSF secretion continues even though ICP increases (see Chapter 33).
The following specific types of hydrocephalus are generally recognized, but there are challenging patients who defy such labels, and their clinical and pathophysiologic features may change over time.1
Communicating Hydrocephalus
Communicating hydrocephalus is characterized by panventricular dilation and occurs as a result of obstruction to the flow of CSF in the subarachnoid space, distal to the foramina of Luschka and Magendie. Therefore, there is communication between the ventricles and the subarachnoid space. This condition is commonly caused by infection or hemorrhage or is idiopathic. Lumbar puncture is generally safe. Table 34-1 lists the types of communicating hydrocephalus.
DEFECTS OF FLOW IN THE SUBARACHNOID SPACE |
CSF, cerebrospinal fluid.
From Pickard JD. Adult communicating hydrocephalus. Br J Hosp Med. 1982;27:35.
Long-Standing Overt Ventriculomegaly in Adults
This form of hydrocephalus develops during childhood, with symptoms being manifested during adulthood. Severe ventriculomegaly in adults is associated with macrocephalus measuring greater than 2 SD in head circumference or neuroradiologic evidence of a significantly expanded or destroyed sella turcica, or both. Aqueduct stenosis is present but may be secondary because it may develop after third ventriculostomy. A spectrum of patients ranging from those with symptoms and signs of increased ICP to those with dementia, gait disturbance, and urinary incontinence may be encountered (see the section on normal-pressure hydrocephalus [NPH]). There is a major risk with surgical intervention for subdural hematomas. The challenge is the timing of intervention—the point at which the significant risk for surgical complications outweighs the slow but inexorable natural history of decline.
Normal-Pressure Hydrocephalus
NPH is a syndrome characterized by gait apraxia, dementia, and incontinence with normal CSF pressure and dilated ventricles. The designation “normal” is misleading because continuous intracranial monitoring has demonstrated the presence of waves of increased ICP, particularly during rapid eye movement (REM) sleep.2 It has been suggested that these abnormal CSF pressure spikes, called B waves, slowly increase ventricular size by exerting intermittent high pressure on the brain parenchyma that results in ischemic damage. Abnormalities of the aging brain parenchyma may make it more susceptible to these forces.3 However, the exact pathogenesis of NPH is still a matter of debate. Theories regarding the underlying pathophysiology of NPH are discussed in a later section. Despite the uncertainty regarding its evolution, NPH is a syndrome that is treatable by CSF diversion (i.e., shunt insertion).
Slit Ventricle Syndrome
The lateral ventricles may collapse in some patients secondary to overshunting or remain at a fixed size because of subependymal gliosis. This may lead to intermittent or complete shunt malfunction. Patients may experience raised ICP without ventricular enlargement, and therefore imaging findings may be falsely reassuring in such cases (unresponsive ventricles). Symptomatic patients may respond to a change in valve setting if a programmable valve is in situ or to revision surgery (change of valve or incorporation of an antisiphon device to prevent overdrainage). Patients with progressive neurological deterioration secondary to raised ICP may require subtemporal decompression. When the ventricles are slit intermittently, endoscopic third ventriculostomy may be possible during periods of relative ventricular dilation. Please see Chapter 186 for a discussion of these issues in children, including shunt removal.
Pathophysiology (see also Chapter 33)
Formation, Circulation, and Reabsorption
The total volume of CSF in the cranial-spinal axis is approximately 150 mL, distributed equally between the two compartments. However, net CSF production is about 0.35 mL/min (500 mL/day), which results in a CSF turnover rate of approximately three to four times per day. Intracranially, CSF is produced mainly by the choroid plexus of the ventricles (70% to 80%).4 The remaining amount is produced by the ependymal lining of the ventricles, by the brain’s capillary bed, and by metabolic water production. The proportion of CSF produced in the ventricular system is unequal; the vast majority of CSF produced by the choroid plexus is from the lateral ventricles, although small amounts are produced in the third and fourth ventricles. CSF is formed by filtration of plasma through fenestrated capillaries and active transport of water and solutes through the epithelial cells of the choroid plexus at the blood-CSF barrier.
CSF circulates through the ventricular system and exits through the foramina of Luschka and Magendie into the cerebellomedullary cistern and onward to the spinal cavity and the subarachnoid spaces of the cerebral convexities. Circulation of CSF is thought to be driven by hydrostatic pressure generated by cerebral arterial pulsation and changes in venous pressure secondary to respiration, change of posture, and other mechanisms.5,6 CSF is resorbed by arachnoid villi that protrude from the subarachnoid space into the lumen of the dural sinuses. Solutes pass via one-way bulk flow into the venous circulation. The exact mechanism of this process has not been fully elucidated but may involve differential pressure between CSF and the venous system and one-way valves formed by overlapping endothelial cells lining the arachnoid villi.5 Figure 34-1 illustrates the concepts just discussed.
Cerebrospinal Fluid Obstruction and Sequelae
Experimental models of acute ventricular obstruction have provided an understanding of the pathophysiologic processes occurring after disruption of the CSF circulation. Initial rapid ventricular dilation is followed by effacement of the cerebral sulci, fissures, and basal cisterns. Transependymal passage of CSF occurs through either an intact or disrupted ependymal lining and results in periventricular edema. Absorption of CSF occurs in the edematous white matter via alternative pathways, such as direct absorption into blood via the blood vessels.7 Destruction of tracts secondary to edema and subsequent gliosis of damaged tissue are believed to occur within the periventricular white matter, with relative sparing of gray matter.8 The resulting white matter damage and reduction in cerebral blood volume may progress to cerebral atrophy. This concept may explain the persistence of neurological deficits in some patients despite successful reduction of ventricular volume after shunt surgery. As a result of some or all of these processes, pathologic compensation for raised ICP may be reached. In a minority of cases, CSF production is reduced because of atrophy of the choroid plexus.7
Progressive Ventriculomegaly in the Context of Normal Pressure and the Concept of Combined Dementia
The confounding situation of progressive ventricular dilation in patients with NPH has led to many theories regarding the underlying pathophysiologic processes occurring in this condition. Hakim and Adams originally proposed that as ventricular enlargement progresses, the biomechanical forces required to maintain the ventricles in a dilated state are smaller.9 Distortion of tissue, including white matter tracts and blood vessels, may lead to damage and ischemia. Loss of elasticity within the brain parenchyma may result in a pressure gradient between the ventricles and periventricular tissue. The resulting excess fluid in the interstitial space may lead to failure of drainage of toxic metabolites. There is also evidence of disruption of cerebral blood flow or distortion of blood vessels, which is believed to lead to watershed ischemia and deep lacunar infarcts. The pattern of disruption of cerebral blood flow in white matter has been demonstrated to take the form of a U-shaped relationship with distance from the ventricles, with the maximal reduction occurring adjacent to the ventricles and progressive normalization toward the subcortical white matter.10
NPH is also thought to be a CSF circulation disorder resulting from an imbalance between production and absorption. Abnormalities in the aging brain may make it uniquely susceptible to intermittent spikes of B waves and result in progressive ventriculomegaly. It has been demonstrated that resistance to CSF outflow increases in a nonlinear fashion with advancing age despite a decrease in the rate of CSF production, which also occurs with increasing age.11 Failure of efficient CSF turnover may also result in an accumulation of potentially toxic metabolic products, such as β-amyloid peptides (Aβ) and tau protein. Such aggregates are thought to be neurotoxic and contribute to small-vessel damage and subsequent leakage of additional toxic metabolites into the interstitial space.12 Moreover, it has been proposed that the two changes noted in aging, reduced CSF production and increased resistance to CSF outflow, may be implicated in a common pathway in the pathophysiology of Alzheimer’s disease and NPH. A predominance of reduced CSF production and turnover may be manifested as Alzheimer’s disease, and conversely, NPH may result from a predominant increase in CSF outflow resistance. A spectrum of disease may exist, including a subset of patients who either have both conditions or have risks for the development of both even if one process is predominant.13
Initial Features of Hydrocephalus
Both communicating and obstructive hydrocephalus may give rise to the same symptoms and signs (i.e., those of hydrocephalus or raised ICP). Alternatively, both types may be associated with normal CSF pressure or spontaneously arrest. The initial features specific to NPH are discussed in the following section. Table 34-2 lists the common symptoms and signs in patients with acute versus chronic hydrocephalus.
TABLE 34-2 Common Initial Features of Acute versus Chronic Hydrocephalus
ACUTE—RAISED INTRACRANIAL PRESSURE |
Normal-Pressure Hydrocephalus
Clinical Findings and Differential Diagnoses
The concept of a “symptomatic occult hydrocephalus with ‘normal’ CSF pressure” was described by Hakim and Adams in 1965 in their landmark paper of observations based on three cases, two secondary and one idiopathic.9 Current guidelines deal primarily with idiopathic as opposed to secondary NPH, which occurs after trauma (such as the two patients in 1965), subarachnoid hemorrhage, intracranial surgery, or meningitis. The manifestations of secondary NPH may be delayed, sometimes many years after the incident in question. Most cases seen by clinicians are of the idiopathic variety. Despite advances in the diagnosis and management of this condition, the exact pathogenesis of NPH remains uncertain. The classic finding in patients with NPH is a clinical triad of symptoms—gait disturbance, dementia, and urinary incontinence. Patients may or may not have the complete triad of symptoms. In addition, many other symptoms have been reported, such as lethargy, apathy, impaired wakefulness, and visuospatial disturbances.
Gait Disturbance
Gait disturbance is the most common initial symptom and occurs in almost 90% of patients.7 Ojemann and colleagues in 1969 noted that gait disturbance could be an initial manifestation of NPH,14 thereby changing the emphasis from earlier descriptions of cognitive disturbance being the primary initial symptom. Fisher subsequently presented a series of 16 patients with shunt-responsive hydrocephalus in which gait disturbance was the initial manifestation in 12 of them.15 In that series, dementia preceded gait disturbance in 1 patient and occurred at the same time in 3 patients. However, in 11 cases of shunt failure, dementia came first in 9 patients and gait disturbance was relatively less severe or was absent.
Common initial symptoms include unsteadiness, recurrent falls, shuffling, and reduced walking speed. More advanced symptoms include difficulty initiating gait and imbalance on turning. Gait in patients with NPH is described as being “magnetic” in nature, characterized by a broad base and slow, small steps with reduced height clearance as though the feet are “stuck to the floor.” Patients may have difficulty rising from a chair or complain of their legs “giving way.”16 Patients may have disturbances in stance with a tendency to lean forward and imbalance exacerbated by eye closure.14,17
The anatomic basis for gait disturbance in patients with NPH remains controversial. In 1947, Yakovlev proposed a theory that paraplegia in patients with hydrocephalus is caused by compression of the internal capsule fibers by the distended third ventricle. However, the general lack of upper motor neuron signs and upper limb involvement in NPH would suggest that such a theory may not account for the characteristic gait apraxia seen in this condition. Indeed, a study using motor evoked potentials and central motor conduction time in both the upper and lower limbs in patients with NPH did not demonstrate any evidence of major pyramidal tract dysfunction or subclinical upper limb involvement in patients who responded to shunt surgery. Prolonged central motor conduction time was seen in the lower limbs of patients who did not improve after surgery.17 However, such methods may be insufficiently subtle to detect small lesions or reversible white matter damage occurring as a result of tissue distortion from hydrocephalus. Pyramidal tract damage may represent progression of these lesions to an end-stage phase of NPH that cannot be reversed by surgery.
Urinary Incontinence
Urinary incontinence may be a separate symptom or may be a consequence of gait disturbance or cognitive impairment. Some patients have urinary frequency rather than true incontinence. This symptom is thought to be due to involvement of the sacral fibers of the corticospinal tract.18 Because urgency of micturition and incontinence are both common problems in older age, the clinical finding may be that of a change or worsening of urinary symptoms rather than a new problem.
Cognitive Impairment
NPH is estimated to account for less than 5% of all cases of dementia. It is essential that patients undergo neuropsychological testing to distinguish the pattern of dementia in NPH from other conditions such as age-related cognitive decline as a result of neurodegenerative processes, including Alzheimer’s disease. The pattern of NPH appears to be a subcortical frontal dysexecutive syndrome.19,20 Cognitive deficits in patients with NPH typically include memory loss, reduced attention, difficulty planning, slowness in thought, and apathy. There may be speech disturbance because of dysexecutive or motivational problems.16 This pattern differs from the cortical deficits of aphasia, apraxia, and agnosia seen in patients with Alzheimer’s disease.21
The most difficult differential diagnosis to consider in the context of NPH is Binswanger’s disease, a form of subcortical vascular encephalopathy. Patients with this condition exhibit a predominantly frontal cognitive deterioration and gait disturbance (ataxia or motor dysfunction, or both), although focal neurological signs may be present. The pathologic changes occurring in patients with Binswanger’s disease are believed to be the result of small-vessel ischemia and subsequent extensive white matter damage. Neuropsychological testing demonstrates features consistent with a frontal subcortical type of dementia, which is similar to the pattern noted in NPH. Similar magnetic resonance imaging (MRI) features may be seen, including ventriculomegaly and the coexistence of MRI white matter changes, such as deep white matter hyperintensities and subcortical lacunar infarctions. Table 34-3 summarizes the list of differential diagnoses for the clinical triad in NPH, aside from other hydrocephalus disorders.
TABLE 34-3 Differential Diagnoses for the Clinical Triad in Normal-Pressure Hydrocephalus, Aside from Other Hydrocephalus Disorders
GAIT DISTURBANCE |
Data from Idiopathic Normal-Pressure Hydrocephalus Guidelines; Relkin N, Marmarou A, Klinge P, et al. Diagnosing idiopathic normal-pressure hydrocephalus. Neurosurgery. 2005;57:S4; and expert opinion.
Neuroradiologic Features
Guidelines published by the Idiopathic NPH Study Group16 include a set of imaging criteria required to justify the diagnosis of idiopathic NPH. Imaging in the form of computed tomography (CT) or MRI is required to establish the diagnosis of NPH. Important differential diagnoses to rule out include obstruction of CSF pathways as a result of tumor or similar pathology, significant cerebral atrophy, and evidence of cerebrovascular ischemia. Ventricular enlargement not entirely attributable to cerebral atrophy or congenital enlargement (Evans’ index >0.3 or comparable measure) should be present. Evans’ index is defined as the maximal width of the anterior ventricular horns divided by the maximal width of the calvaria at the level of the foramen of Monroe. An alternative measurement is the bicaudate ratio, which has been demonstrated to have excellent interobserver agreement and is more sensitive to changes in ventricular size.22 This is the minimal intercaudate distance divided by the brain width along the same line. Significant ventriculomegaly is defined as a ratio of 0.25 or greater with this method.
There should also be one of the following supportive features: enlargement of the temporal horns of the lateral ventricles not entirely attributable to hippocampus atrophy; callosal angle of 40 degrees or greater; evidence of altered brain water content, including periventricular signal changes not attributable to microvascular ischemic changes or demyelination; or aqueductal or fourth ventricular flow void on MRI. Other imaging findings were acknowledged to be supportive of the diagnosis but not required, including a brain imaging study performed before the onset of symptoms demonstrating the absence of ventriculomegaly or smaller ventricles, a radionuclide cisternogram showing delayed clearance of the radiotracer over the cerebral convexities after 48 to 72 hours, cine MRI showing an increased ventricular flow rate, or a single-photon emission computed tomography (SPECT)-acetazolamide challenge showing decreased periventricular perfusion that is not altered by acetazolamide.16
The imaging finding of deep white matter hyperintensities in a patient with NPH has been shown to be inversely correlated with shunt responsiveness.23 Such white matter hyperintensities are probably a marker of comorbidity, with patients being more prone to general complications of surgery when they are present. However, other studies have demonstrated that the presence of these lesions may not be predictive of a poor outcome after shunt surgery. This was the subject of an MRI study by Tullberg and colleagues, who used conventional MRI sequences to examine these lesions and other MRI variables in a group of NPH patients undergoing surgery.24 The authors found no correlation between the presence of these parameters and poor outcome after surgery. Akiguchi and associates further demonstrated that there was improvement in ventriculomegaly and mean total scores for white matter lesions in patients who clinically improved after surgery, thus implying that these white matter lesions may be reversible.25 In this patient cohort the majority had parkinsonism (71%), but other coexisting comorbid conditions, such as small-vessel disease (29%), hypertension (41%), and diabetes (35%), were also found. Eighty-eight percent of patients had white matter lesions noted on CT or MRI. These contradictory findings illustrate the continuing debate regarding the presence of deep white matter hyperintensities and their correlation to small-vessel disease.
Supplementary Prognostic Testing
Guidelines on the value of supplementary tests conclude that a single standard for the prognostic evaluation of patients with idiopathic NPH is lacking. However, supplementary tests can increase the prognostic accuracy to greater than 90%.26
Three supplementary tests are currently recommended as options:
The method of choice depends on local experience and the availability of equipment. Direct ICP measurement may be useful to exclude other more acute causes of hydrocephalus but does not contribute to prognostic assessment. Radionuclide cisternography is no longer a favored option because this technique does not improve the diagnostic accuracy of combined clinical and CT criteria in patients with presumed NPH.27
A lumbar puncture “tap test” has been shown to produce a specificity of 100% with a sensitivity of 26%,28 provided that it is performed at a high volume (i.e., withdrawal of 40 to 50 mL of CSF). Symptomatic improvement after removal of CSF has a high positive predictive value (73% to 100%) of a probably favorable outcome with shunt placement.26 It has to be remembered that improvement after a shunt is often delayed in many patients, so a simple tap test would not be expected to reveal all patients who might benefit from a shunt. However, the low sensitivity of the “tap test” precludes using this method as a diagnostic tool for exclusion. Nonetheless, a lumbar puncture is often used as a first-line investigative tool to establish that CSF pressure is within the normal range (5 to 18 mm Hg/7 to 24 cm H2O) and that no biochemical or microbiologic abnormalities are present. Prolonged external lumbar drainage in excess of 300 mL is associated with high sensitivity (50% to 80%), specificity (80%), and positive predictive value (80% to 100%).26,28 However, this method requires inpatient stay and carries a risk for the complications of nerve root irritation, hemorrhage, and CSF infection.
Measurement of CSF outflow resistance, thought to reflect the CSF absorption pathways, is well established. Fluid is injected into a CSF space (e.g., ventricles or lumbar sac) either by bolus or infusion. CSF outflow resistance can then be calculated with a pressure-volume study and used to assess the CSF circulation for signs of disturbance.29 The advantage of this technique is that it requires only day attendance and can also be performed through a preimplanted ventricular reservoir device. In the Dutch NPH study, outflow resistance greater than 18 mm Hg/mL per minute had a specificity of 87% and a sensitivity of 46%.30
Current guidelines recommend that all patients suspected of having idiopathic NPH be considered for supplementary tests with one or more of the three methods.23 Prolonged external drainage has the highest sensitivity and positive predictive value but is associated with a higher complication rate. The other two methods can be considered in an outpatient setting, but measurement of CSF outflow resistance requires specialist equipment and interpretation of results. However, measurement of CSF outflow resistance can also be used as a method of comparing CSF hydrodynamics before and after shunt insertion to investigate the shunt responsiveness of a patient in the context of deterioration or failure to respond to intervention.
It should also be noted that alternative methods are available, such as the lumbar subcutaneous shunt proposed by Mendelow’s group.31
Neuroradiologic Features of Hydrocephalus
Figures 34-2 to 34-13 illustrate key features of hydrocephalus seen on imaging.
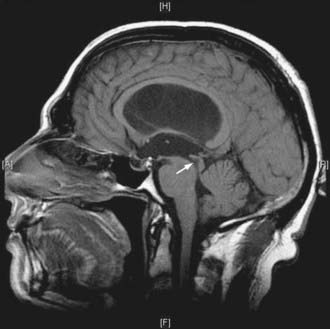
FIGURE 34-7 Example of a patient with stenosis of the aqueduct (arrow)—sagittal T1-weighted magnetic resonance imaging.
Physiologic Testing of Cerebrospinal Fluid Dynamics
When the clinical and radiologic features clearly reveal hydrocephalus and raised ICP, no further investigations are required unless a mass lesion is the cause of the hydrocephalus. However, there are many patients with chronic forms of ventriculomegaly in whom the clinical and radiologic features overlap with other conditions. The study of CSF dynamics, although invasive, may help in the diagnosis, be of assistance in decisions regarding surgical intervention, and provide a baseline for the long-term management of a shunted patient in whom shunt malfunction may develop. Despite early enthusiasm, such physiologic testing has not been widely adopted.32–36 More recent systematic studies have led to the first guidelines for the management of NPH,30,37–40 which recommend the inclusion of some forms of physiologic measurements.26
Cerebrospinal Fluid Drainage and Dynamics
Drainage of CSF into the cerebral venous system is described in Chapter 33. The pathophysiologic processes of CSF obstruction and ventriculomegaly are described in the section “Pathophysiology.” In communicating hydrocephalus, intrathecal injection of radioisotopes has previously demonstrated little passage of these markers over the convexity (i.e., a convexity block). Accumulation within the ventricles may persist for more than 48 hours as indeed reentry of CSF (ventricular stasis or reflux). This is thought to cause reversal of the normal flow of interstitial fluid from the brain into the ventricles so that there is net flow into the brain parenchyma. The mechanism of absorption of such fluid remains a subject of conjecture. Experimental studies suggest that perivascular absorption may occur in the opposite direction of cerebral blood flow with absorption thereafter into the cervical lymphatics via the olfactory mucosa. There is no direct evidence for such a mechanism in humans.
Mathematical Modeling of the Cerebrospinal Fluid Circulation—a Platform for Interpretation of Pressure-Volume Tests of Cerebrospinal Fluid Dynamics
Davson and coworkers41 demonstrated the passive nature of CSF absorption such that
where If is the CSF production rate and Rout is outflow resistance. Rout has been assessed in normal subjects and found to range from 6 to 10 mm Hg/mL per minute.35,42 The inverse of Rout is termed the conductance to CSF reabsorption or outflow.36
Sagittal sinus pressure (PSS) is considered to be a constant parameter that is determined by central venous pressure. However, it is not certain that interaction between changes in CSF pressure and PSS does not exist in all circumstances; in patients with benign intracranial hypertension, PSS is frequently elevated because of stenosis of the lateral sinuses.43 Direct measurement of venous sinus pressure along with ICP reveals strict coupling between both pressures (Fig. 34-14).44
The Pressure-Volume Curve
It is known that as CSF pressure increases, the compliance of the brain decreases. The electrical model of CSF compensation adapted from Marmarou32 is presented in Figure 34-15. Storage of CSF is proportional to cerebrospinal compliance C (mL/mm Hg):
Compliance of the cerebrospinal space is inversely proportional to the gradient of CSF pressure p and the reference pressure po45,46:
More sophisticated models have been formulated but have yet to become useful in clinical practice.48–52
Monitoring of Intracranial Pressure
ICP monitoring is relevant in patients with chronic forms of hydrocephalus and in some patients with possible shunt malfunction but confusing symptoms and signs. Although isolated measurements of CSF pressure in patients with communicating hydrocephalus and NPH may be in the normal range, overnight monitoring may reveal dynamic phenomena such as increased Lundberg “B waves.”53 B waves are slow waves of ICP lasting 20 seconds to 2 minutes. These waves are almost universally present in ICP recordings, probably even in healthy volunteers.36,54,55 The presence of B waves for more than 80% of the period of ICP monitoring is thought to indicate that it is much more likely than not that shunting would be helpful. Various attempts at more precise quantification have yet to be generally accepted.
Monitoring of ICP can be performed safely with intraparenchymal probes. ICP monitoring via lumbar puncture or a needle inserted into a preimplanted reservoir is used less frequently. Connection of the pressure monitor to a computer performing real-time analysis is very helpful. The best results can be derived from overnight ICP monitoring. If this is impossible, a minimum of 30 minutes of ICP monitoring is required. Instant manometric lumbar CSF pressure measurements may be helpful but are known to be misleading56 (Fig. 34-16). In patients with normal CSF dynamics, baseline pressure should be normal (i.e., <15 mm Hg) on overnight monitoring. Vasogenic waves of ICP, particularly intensive during the REM phase of sleep, are probably also present in normal conditions. The presence of vasogenic waves greater than 25 mm Hg for a period of around 10 minutes should be classified as intermittent intracranial hypertension. The average overnight RAP index should be less than 0.6 in patients with good compensatory reserve.57,58 The overnight magnitude of slow waves is considered increased when their average value is greater than 1.5 mm Hg. The presence of plateau waves is always a bad prognostic sign. An example of pathologic overnight ICP recording is presented in Figure 34-17.
During the recording, detection of pulse amplitude proves that the ICP waveform is properly being transmitted to the transducer. Lack of amplitude implies an invalid pressure recording in most cases.59 In our own material there is no evidence that pulse amplitude is a strong predictor of outcome after shunting as reported by other authors.59 Analysis of a subgroup of idiopathic NPH patients with a stable follow-up assessment suggested that when the pulse amplitude is large (>3 mm Hg), improvement is very likely (>90% patients improve). When the pulse amplitude is less than 2 mm Hg, improvement is as equally probable as lack of improvement.
There is no difference in amplitude between males and females. Pulse amplitude increases slightly with age but does not show any correlation with the duration of symptoms of NPH or their severity. However, pulse amplitude is lower in patients with idiopathic NPH and no any evidence of coexisting cerebrovascular disease than in patients with clear evidence of vascular problems.60 After shunting, pulse amplitude decreases. Differences are significant both at baseline and during infusion studies.61 In shunted patients, pulse amplitude is lower in the context of normally functioning shunts than in patients with blocked shunts.
Clinical Tests of Cerebrospinal Fluid Dynamics
The computerized infusion test62,63 is a modification of the traditional constant-rate infusion as described by Katzman and Hussey.64 The method requires infusion of fluid into any accessible CSF compartment proximal to any suspected block. The options are a lumbar tap or intraventricular infusion via a subcutaneously positioned reservoir connected to an intraventricular catheter or shunt antechamber. In such cases, two hypodermic needles (25 gauge) are used: one for the pressure measurement and the second for the infusion. There is an approximate 0.5% risk of introducing infection during CSF infusion studies, which has to be weighed against the risk of misdiagnosis or unnecessary shunt revisions.
During the infusion, the mean pressure and pulse amplitude readings over time are calculated (Fig. 34-18A and B). Resistance to CSF outflow can be calculated by simple arithmetic as the difference between the value of the plateau pressure during infusion and the resting pressure divided by the infusion rate. Precise measurement of the final pressure plateau is not possible when strong vasogenic waves arise or excessive elevation of pressure above the safe limit of 40 mm Hg is recorded. However, computerized analysis produces results even in difficult cases when the infusion is terminated prematurely. The algorithm uses time series analysis for retrieval of volume-pressure curves (Fig. 34-18C), least-mean-square model fitting (Fig. 34-18A), and examination of the relationship between pulse amplitude and mean CSF pressure (Figure 34-18D).
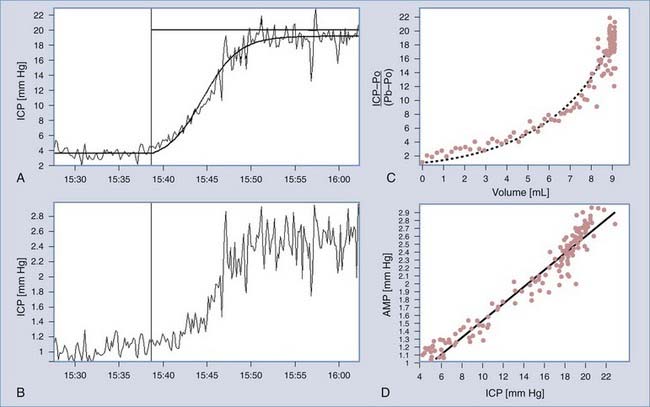
FIGURE 34-18 Methods of identification of the model of cerebrospinal fluid (CSF) circulation during infusion at a constant rate. A, Recording of real CSF pressure (intracranial pressure [ICP]) versus increasing time during infusion with an interpolated modeling curve.7 Infusion at a constant rate of 1.5 mL/min starts from the vertical line. B, Recording of pulse amplitude (AMP) during infusion. It is customarily presented as different variables, in addition to mean ICP. The rise in AMP is usually well correlated with the rise in ICP. C, Pressure-volume curve. On the x-axis, an effective increase in volume is plotted (i.e., infusion and production minus reabsorption of CSF). On the y-axis is the increase in pressure measured as a gradient of the current pressure minus the reference pressure Po relative to baseline pressure Pb. D, Linear relationship between pulse amplitude and mean ICP. The intercept of the line with the x-axis (ICP) theoretically indicates the reference pressure Po.
Differentiation between Brain Atrophy and Normal-Pressure Hydrocephalus
CSF dynamics in patients with NPH is characterized by a normal baseline pressure (ICP <18 mm Hg). Resistance to CSF outflow (>13 mm Hg/mL per minute) is increased. The B waves recorded during infusion studies are regular. Pulse amplitude is well correlated with mean ICP. Compensatory reserve at baseline is usually good (RAP index <0.6), and the elastance coefficient is usually slightly increased (E >0.2 1/mL)—see Figure 34-19.
Patients suffering predominantly from brain atrophy have normal CSF circulation. Infusion studies in these patients typically demonstrate low opening pressure, resistance to CSF outflow, and low pulse amplitude (ICP <12 mm Hg, RCSF <12 mm Hg/mL per minute, amplitude <2 mm Hg). Compensatory reserve at baseline is very good (RAP <0.5) as a result of the low elasticity of the atrophic brain (E <0.2 1/mL). Vasogenic waves are rather limited during the period of the recording. Mean ICP increases smoothly during the infusion and decreases in a similar fashion after infusion, similar to the inflation and deflation of a balloon (Fig. 34-20).
Noncommunicating and Acute Hydrocephalus
Obstructive hydrocephalus can be safely assessed by ventricular infusion (via a reservoir). Typically, high intracranial resting pressure and high resistance to CSF outflow are demonstrated (ICP >15 mm Hg, RCSF >13 mm Hg/mL per minute). Elasticity is high (>0.20 1/mL), RAP is elevated above 0.6, and the pulse amplitude is high (>4 mm Hg), findings indicative of poor compensatory reserve. Acute communicating hydrocephalus has a similar pattern of parameters, with frequent deep vasogenic waves (including plateau waves53).
Testing of Cerebrospinal Fluid Dynamics in Shunted Patients
When a shunt drains properly, resting pressure remains at or below the shunt’s operating pressure. Infusion studies or overnight ICP monitoring repeated with a shunt in situ should always be considered and the results compared with the tests performed before surgery. Abnormal cerebrospinal compensatory parameters, such as high resting pressure, increased resistance to CSF outflow, low compensatory reserve, increased activity of slow waves, or a high amplitude of the pulse waveform, should return to normal after successful shunting.61,65 In valves with low hydrodynamic resistance and a well-defined opening pressure, a sharp plateau of the pressure trend is seen about 1 to 5 mm Hg above the level of the shunt’s operating pressure.66 The magnitude of this plateau should not exceed a value as derived from the following equation:
where Rshunt is the hydrodynamic resistance of the opened shunt and 5 mm Hg is a “safety margin” and a credit for possible non-zero abdominal pressure (in patients with possible increased abdominal pressure, this value should be increased to 10 to 15 mm Hg). When shunt operating pressures and Rshunt are measured in the laboratory, these parameters provide invaluable guidelines for shunt testing in vivo67; see Figure 34-21 for examples of a working (A) and blocked (B) shunt.
When a shunted patient has low-pressure headaches, small or slit ventricles, subdural collections, or chronic hematomas, CSF overdrainage should be considered. Overdrainage related to body posture may be assessed with a tilting test. When the baseline pressure measured in the horizontal body position is low (usually negative), overdrainage is possible. A change in posture to sitting generally produces a further decrease in pressure. If the pressure decreases to a value lower than −10 mm Hg (the 95% confidence limit for ICP in the upright position in nonshunted patients is around −8 mm Hg), overdrainage is likely (Fig. 34-22).
The majority of contemporary valves usually have low hydrodynamic resistance,68 a feature that may result in overdrainage from periodic oscillations in cerebrovascular volume. The expanding cerebrovascular bed acts like the membrane of a water pump with a distal low-resistance valve.69 Early morning headache should not be always assumed to be “high pressure.” It may be a consequence of the low pressure caused by nocturnal overdrainage.
In shunted patients with slit ventricles, baseline pressure recorded from the shunt antechamber may not demonstrate a pulse waveform. In this situation, collapse of the ventricular walls around the proximal catheter results in the lack of pressure transmission. A pulse waveform often appears after infusion starts as the buildup of pressure opens up the ventricular cavity (Fig. 34-23).
Management
Surgical management is required for patients with symptomatic acute or chronic hydrocephalus. Surgery is recommended for patients with idiopathic NPH and a favorable risk-to-benefit ratio.70 Medical therapies, such as acetazolamide and repeated lumbar puncture, do not have a role in longer term management but may be used as temporizing measures before definitive treatment. Surgical management of any obstructive lesion, such as a tumor, may be required in addition to CSF diversion. The two main forms of surgical management for hydrocephalus are shunt insertion and endoscopic third ventriculostomy. Endoscopic choroid plexus coagulation is favored in some units, but the results of a randomized controlled trial are awaited. Relief from symptomatic hydrocephalus and prevention of neurological deterioration may be achieved with or without a significant reduction in ventricular size, particularly in patients with chronic hydrocephalus.
Shunt Insertion
The most commonly used shunt in modern neurosurgery is a ventriculoperitoneal shunt. A ventricular catheter is placed into the lateral ventricles, usually from a frontal or occipital approach, and connected to the remainder of the shunt system. The ideal trajectory of the ventricular catheter would avoid areas of functional neuroanatomy and the choroid plexus while being at sufficient depth for CSF drainage to occur through all distal drainage openings despite changes in ventricular size. Stereotactic or image-guided placement of ventricular catheters is increasingly being used with normal or small ventricles to reduce the incidence of misplaced ventricular catheters. When raised ICP is associated with small ventricular size, such as benign intracranial hypertension, placement of a lumboperitoneal or lumbopleural shunt may be the preferred option. Ventriculoatrial shunts were previously in common use and may still be the treatment of choice in patients with significant truncal obesity, extensive abdominal abnormalities, or a history of multiple abdominal procedures. Indeed, it is possible to place the distal end of a shunt into any visceral cavity, such as the pleural cavity. Other more unusual shunt options that have been described include the Torkildsen shunt (ventricle to the cisternal space) and the Sinushunt (ventricle to the venous sinus). In addition to shunts placed within the ventricle, treatment of hydrocephalus may involve drainage of one or more cystic or subdural cavities, such as an arachnoid cyst or subdural hygroma.71
It is usual practice to incorporate a valve mechanism into the shunt circuit at the time of insertion. CSF drainage can be regulated either by pressure or by flow. Antisiphon devices to prevent overdrainage can be attached to the shunt circuit if required. Some valves incorporate such devices. Reservoir devices can also be fitted to allow access for subsequent CSF analysis or interrogation of CSF hydrodynamics. Some valves have separate reservoir chambers within their design. Many modern valves are programmable and allow subsequent adjustments in differential pressure after implantation. This permits changes to be made in the valve setting after insertion, often on an outpatient basis. The type of valves selected for patients with acute hydrocephalus is based on local experience, published data, and available supplementary information, such as drainage requirements assessed by external ventricular or lumbar drainage. In patients with NPH, no series has demonstrated a significant benefit with a particular type of shunt or valve, although there is a trend favoring low-pressure valves.72 Patients with low-pressure hydrocephalus may require a valveless shunt system. Shunt devices are discussed in greater depth later.
Endoscopic Third Ventriculostomy
Patients with obstructive hydrocephalus may benefit from endoscopic third ventriculostomy rather than shunt insertion (see Chapter 36). This technique involves passing an endoscope (rigid or flexible) through the lateral ventricles (usually via one of the frontal horns) directly into the third ventricle. If the floor of the third ventricle can safely be visualized, a stoma can be created within it to allow fluid to drain directly into the basal cisterns. The advantage of this procedure over a shunt is that it avoids the potential morbidity of shunt infection and lifelong risk for revision. This procedure has little role in the management of true communicating hydrocephalus. However, some patients with NPH have a late-onset form of relative aqueduct stenosis. In these patients, there is a mismatch between the degree of ventriculomegaly in the lateral ventricles and third ventricle and between the aqueduct of Sylvius and the fourth ventricle. This implies that the hydrocephalus has an obstructive element (although the hydrocephalus is still “communicating” in terms of the chronic signs and symptoms). In this situation, endoscopic third ventriculostomy may be considered. Gangemi and coauthors reported improvement in 72% of NPH patients with this technique and a relatively low complication rate (intracerebral hemorrhage in 4%).73
Shunts
An operating flow pressure of around 0.3 mL/min marks the range of ICP that can be measured in a patient with a properly functioning shunt in a horizontal body position. In ball-on-spring valves, this pressure is very stable over time but is sensitive to dynamic changes in ICP. This type is in contrast to silicone membrane valves, in which the operating pressure may vary within a range of 4 to 6 mm Hg in low, medium, or high ranges. Randomized trials have failed to demonstrate the superiority of different types of valve construction.74 It is well recognized that physiologic ICP is variable within the limits of 0 to 15 mm Hg. Artificial attempts to set a constant ICP by using valves with minutely determined opening pressure and very low hydrodynamic resistance may not be useful.
Generally, a shunt consists of three parts: inlet tubing (ventricular or lumbar drain), which is a thin short tube with an inner diameter of 0.9 to 1.2 mm; a valve; and a distal drain, a longish silicone rubber tube—see Figure 34-24.
Valves can be classified according to their construction:
Examples of pressure-flow curves of differential and flow-regulating valves are shown in Figure 34-25. Differential pressure valves are characterized by opening/closing pressure (pressure above/below the point at which flow starts/ceases) and an inverse of the slope of the curve within the range of full drainage (≥0.3 mL/min), which is termed the resistance of the valve. In flow-regulating valves, the resistance of the valve (within the range of regulation) is infinitely high. In most differential pressure valves, the resistance is very low (lower than physiologic resistance to CSF outflow).68
Opening and closing pressure may be programmed externally in some models. Magnetic programming was used for the first time in a French model—the Sophy Programmable Valve. The next generation of programmable valves was designed by S. and C. Hakim. Much more precise programming (18 steps) was achieved. The programmable Strata valve followed, a programmable equivalent of the popular fixed-pressure Delta Valve, integrated with a membrane siphon controlling device.75 Patients should be counselled regarding the possibility that some magnetic fields may modify the setting of these valves under some circumstances, such as in an MRI scanner. Some valves are more prone to this problem than others.76 In pediatric patients with programmable valves, parents should be made aware that strong magnetic toys could potentially result in a change in the setting of the valve. Newer adjustable valves (Pro-GAV [B. Braun, Melsungen, Germany] and Polaris [Sophysa, Orsay, France]) offer mechanisms that are able to prevent accidental readjustment, even in a 3-T MRI scanner.
Because the majority of contemporary valves have low hydrodynamic resistance, the net resistance of a shunt depends to a great extent on the diameter and length of the distal drain. In patients in whom clinical complications related to overdrainage are likely to develop, implantation of an antisiphon device should be considered. However, the risk for overdrainage related to vasomotor nocturnal waves may still be high.29 A standard peritoneal open-end catheter (usually around 90 cm long, 1.1 to 1.2 mm in diameter) provides a resistance of 2.5 to 3.5 mm Hg/mL per minute. This may amount to 100% to 200% of the overall resistance of the valve itself. It must be recognized that shortening of a drain decreases overall shunt resistance, thus making it potentially more susceptible to overdrainage.
Therefore, the ideal shunt should restore the normal circulation of CSF and the normal pattern of extrachoroidal fluid flow within the brain, prevent excessive buildup of ICP, and encourage restitution of the cerebral mantle, which consists of both gray and white matter. After shunting, the hydrodynamic performance of the shunt interacts with the patient’s own CSF circulatory pattern. The result of shunting can be forecasted by a parallel connection of the model of CSF dynamics and pressure-flow characterization of the shunt. When a patient does not improve after shunting or deteriorates after a period of improvement, an infusion study or overnight ICP monitoring may confirm shunt malfunction if the hydrodynamic performance curve of a shunt is known.58,77,78 Many of the parameters of shunts are never disclosed or are disclosed insufficiently or even inaccurately by manufacturers. Programs of shunt evaluation have created an opportunity to understand and demonstrate some important phenomena related to the hydrodynamics of CSF flow in shunted patients and generate guidelines for “more physiologic” valves.67,68,79
Complications
Surgical approaches to NPH are associated with relatively low morbidity and mortality rates. The general risks related to shunt surgery include infection, bleeding, CSF leakage, seizures, and neurological deficit. There is an estimated 3% to 4% risk for intracerebral hemorrhage73,80 and an estimated 1% to 2% risk for catastrophe, including coma or mortality. The risk for mortality can be greater for an individual NPH patient with multiple comorbid conditions. More commonly occurring complications are listed in the following paragraphs.71 Shunt surgery for other types of communicating or acute hydrocephalus is associated with similar risks. Revision or multiple reoperations for hydrocephalus can be challenging to manage. Figure 34-26 lists reasons given for shunt revision in adults (17 years and older) obtained from the U.K. Shunt Registry. It is important to note that these data have been derived purely on an intention-to-treat basis.
Both overdrainage and underdrainage of CSF can occur with any type of shunt system. Excessive CSF drainage from the ventricles is thought to increase the risk for subdural hematoma, the reported incidence of which varies from 2% to 17%.70 The resulting hematoma can lead to neurological deficits, coma, or death. It may be useful to perform a postoperative CT scan to verify the accuracy of catheter placement and exclude new subdural hygromas into which hemorrhage may occur. Subdural fluid collections have a wide spectrum, from CSF subdural hygromas to frank hematomas. Strategies for treatment include conservative management by means of serial scanning with or without resetting of the valve to reduce CSF drainage. Symptomatic acute or chronic subdural hematomas require evacuation by either bur holes or a minicraniotomy. Severe cases may necessitate ligation of the shunt tubing until resolution of the hematoma can be achieved.
Shunt malfunction may require formal revision surgery to optimize CSF drainage. However, good long-term outcomes have been reported despite the need for multiple shunt operations in the case of shunt blockage.81 Malfunction can occur within any part of the shunt system, from blockage of the drainage openings of the ventricular catheter, to valve dysfunction, to distal blockage. The distal end of a ventriculoperitoneal catheter may become kinked or malpositioned because of shifting and peristalsis of abdominal contents. A clot or emboli may form as a result of a ventriculoatrial catheter. Disconnection or breakage of one or more shunt parts may occur, particularly in patients with epilepsy or other movement disorders or in patients prone to falls. Iatrogenic shunt dysfunction as a result of malpositioning of the shunt during insertion may necessitate revision surgery. Shunt systems can intermittently malfunction because of positional reasons. Strategies to reduce this complication include meticulous planning of placement of the various shunt components, such as antisiphon/antigravity devices, which work best in a true vertical position. Careful handling of any excess tubing during shunt insertion or revision surgery is required to avoid unintentional kinks in the shunt circuit.
Patient Outcomes
A study of CSF dynamics after shunting by Petrella and colleagues investigated this dilemma in a cohort of 25 patients with NPH.61 Patients who still had some adverse symptoms, mainly headaches, slow improvement, or no improvement of ventriculomegaly after shunt insertion, underwent infusion studies to assess shunt function. In all cases the shunts were confirmed to be draining CSF adequately. The authors demonstrated that there were significant improvements in mean ICP, pressure-volume compensation, and resistance to CSF outflow, as well as a decrease in vasogenic pressure waves. Such a study demonstrates the usefulness of fitting shunt systems with built-in access devices for the interrogation of shunt malfunction. However, the return of disturbed CSF dynamics to normal values, even in patients with disappointing clinical or radiologic improvement, implies that management of hydrocephalus as a pure CSF circulatory disorder may be insufficient. The element of reversibility of damage to important white matter connections may be essential for the continued improvement of symptoms after successful CSF diversion.
Audit data from our home institution have shown that in patients with NPH who underwent insertion of a ventriculoperitoneal shunt after assessment in a multidisciplinary CSF clinic, formal neuropsychological testing, and infusion studies, 69% (101 of 146) demonstrated initial clinical improvement.82 Of the 31% of patients who were shunt nonresponders, most had potential overlap with other neurodegenerative conditions, including Parkinson’s and Alzheimer’s disease. At a 2-year follow-up, 54% of the patients reported continued or sustained improvement. Despite initial improvement and a working shunt, as determined by further testing, 15% of patients suffered a late decline in terms of gait or cognition, or both. These figures are similar to outcomes from other worldwide series. Published rates of improvement after surgical intervention range from 53% to 78.9%.27,30,83 A prospective study by Malm and coworkers demonstrated improvement in gait and cognitive function in 72% and 67% of patients, respectively.38 Raftopoulos and coauthors reported improvement in cognitive function in 66.6% of patients and improvement in bladder function and gait apraxia in more than 90% 1 year after surgery.84 A study by Savolainen and associates demonstrated that the early benefits of surgery declined significantly over a 5-year period from 74% to 47%.85
However, there is controversy over the relative contribution of concomitant comorbid disease to the outcome figures in longer term follow-up studies of NPH. In the published idiopathic NPH guidelines, Klinge and colleagues concluded that a standard for outcome assessment of shunt treatment of idiopathic NPH was lacking because of different criteria for selection of patients, postoperative assessment, and follow-up intervals.40 The 1-year postshunt period was thought to be a potential determinant of shunt outcome; shorter term results are more likely to be influenced by shunt-associated risks and longer term results may be related to concomitant cerebrovascular and vascular diseases. Recommendations made included the following: follow-up at 3, 6, and 12 months; careful evaluation of nonresponders for shunt malfunction; and the use of objective scales for measurement of improvement.
Bergsneider M, Black PM, Klinge P, et al. Surgical management of idiopathic normal-pressure hydrocephalus. Neurosurgery. 2005;57:S29.
Black PM, Ojemann RG, Tzouras A. CSF shunts for dementia, incontinence, and gait disturbance. Clin Neurosurg. 1985;32:632.
Boon AJ, Tans JT, Delwel EJ, et al. Dutch Normal-Pressure Hydrocephalus Study: randomized comparison of low- and medium-pressure shunts. J Neurosurg. 1998;88:490.
Boon AJ, Tans JT, Delwel EJ, et al. Dutch Normal-Pressure Hydrocephalus Study: prediction of outcome after shunting by resistance to outflow of cerebrospinal fluid. J Neurosurg. 1997;87:687.
Czosnyka M, Czosnyka ZH, Whitfield PC, et al. Age dependence of cerebrospinal pressure-volume compensation in patients with hydrocephalus. J Neurosurg. 2001;94:482.
Czosnyka Z, Czosnyka M, Owler B, et al. Clinical testing of CSF circulation in hydrocephalus. Acta Neurochir Suppl. 2005;95:247.
Czosnyka Z, Czosnyka M, Richards HK, et al. Laboratory testing of hydrocephalus shunts—conclusion of the U.K. Shunt Evaluation Programme. Acta Neurochir (Wien). 2002;144:525.
Czosnyka ZH, Czosnyka M, Whitfield PC, et al. Cerebral autoregulation among patients with symptoms of hydrocephalus. Neurosurgery. 2002;50:526.
Davson H, Hollingsworth G, Segal MB. The mechanism of drainage of the cerebrospinal fluid. Brain. 1970;93:665.
Devito EE, Pickard JD, Salmond CH, et al. The neuropsychology of normal pressure hydrocephalus (NPH). Br J Neurosurg. 2005;19:217.
Drake JM, Kestle JR, Milner R, et al. Randomized trial of cerebrospinal fluid shunt valve design in pediatric hydrocephalus. Neurosurgery. 1998;43:294.
Eide PK. Intracranial pressure parameters in idiopathic normal pressure hydrocephalus patients treated with ventriculo-peritoneal shunts. Acta Neurochir (Wien). 2006;148:21.
Hakim S, Adams RD. The special clinical problem of symptomatic hydrocephalus with normal cerebrospinal fluid pressure. Observations on cerebrospinal fluid hydrodynamics. J Neurol Sci. 1965;2:307.
Higgins JN, Owler BK, Cousins C, et al. Venous sinus stenting for refractory benign intracranial hypertension. Lancet. 2002;359:228.
Iddon JL, Pickard JD, Cross JJ, et al. Specific patterns of cognitive impairment in patients with idiopathic normal pressure hydrocephalus and Alzheimer’s disease: a pilot study. J Neurol Neurosurg Psychiatry. 1999;67:723.
Krauss JK, Droste DW, Vach W, et al. Cerebrospinal fluid shunting in idiopathic normal-pressure hydrocephalus of the elderly: effect of periventricular and deep white matter lesions. Neurosurgery. 1996;39:292.
Lundberg N. Continuous recording and control of ventricular fluid pressure in neurosurgical practice. Acta Psychiatr Scand Suppl. 1960;36:1.
Malm J, Kristensen B, Karlsson T, et al. The predictive value of cerebrospinal fluid dynamic tests in patients with the idiopathic adult hydrocephalus syndrome. Arch Neurol. 1995;52:783.
Ojemann RG, Fisher CM, Adams RD, et al. Further experience with the syndrome of “normal” pressure hydrocephalus. J Neurosurg. 1969;31:279.
Pickard JD. Adult communicating hydrocephalus. Br J Hosp Med. 1982;27:35.
Relkin N, Marmarou A, Klinge P, et al. Diagnosing idiopathic normal-pressure hydrocephalus. Neurosurgery. 2005;57:S4.
Silverberg GD, Mayo M, Saul T, et al. Alzheimer’s disease, normal-pressure hydrocephalus, and senescent changes in CSF circulatory physiology: a hypothesis. Lancet Neurol. 2003;2:506.
Tullberg M, Jensen C, Ekholm S, et al. Normal pressure hydrocephalus: vascular white matter changes on MR images must not exclude patients from shunt surgery. AJNR Am J Neuroradiol. 2001;22:1665.
Vanneste J, Augustijn P, Dirven C, et al. Shunting normal-pressure hydrocephalus: do the benefits outweigh the risks? A multicenter study and literature review. Neurology. 1992;42:54.
Walchenbach R, Geiger E, Thomeer RT, et al. The value of temporary external lumbar CSF drainage in predicting the outcome of shunting on normal pressure hydrocephalus. J Neurol Neurosurg Psychiatry. 2002;72:503.
Williams MA, Razumovsky AY, Hanley DF. Evaluation of shunt function in patients who are never better, or better than worse after shunt surgery for NPH. Acta Neurochir Suppl. 1998;71:368.
1 Pickard JD. Adult communicating hydrocephalus. In: Harrison MJG, editor. Contemporary Neurology. London: Butterworth; 1984:543-555.
2 Verrees M, Selman WR. Management of normal pressure hydrocephalus. Am Fam Physician. 2004;70:1071.
3 Factora R. When do common symptoms indicate normal pressure hydrocephalus? Cleve Clin J Med. 2006;73:447.
4 Pickard JD. Adult communicating hydrocephalus. Br J Hosp Med. 1982;27:35.
5 Rapoport SI. Blood-Brain Barrier in Physiology and Medicine. New York: Raven Press; 1976.
6 Williams B. Cerebrospinal fluid pressure changes in response to coughing. Brain. 1976;99:331.
7 Milhorat TH. Hydrocephalus and the Cerebrospinal Fluid. Baltimore: Williams & Wilkins; 1972.
8 Weller RO, Shulman K. Infantile hydrocephalus: clinical, histological, and ultrastructural study of brain damage. J Neurosurg. 1972;36:255.
9 Hakim S, Adams RD. The special clinical problem of symptomatic hydrocephalus with normal cerebrospinal fluid pressure. Observations on cerebrospinal fluid hydrodynamics. J Neurol Sci. 1965;2:307.
10 Momjian S, Owler BK, Czosnyka Z, et al. Pattern of white matter regional cerebral blood flow and autoregulation in normal pressure hydrocephalus. Brain. 2004;127:965.
11 Czosnyka M, Czosnyka ZH, Whitfield PC, et al. Age dependence of cerebrospinal pressure-volume compensation in patients with hydrocephalus. J Neurosurg. 2001;94:482.
12 Silverberg GD. Normal pressure hydrocephalus (NPH): ischaemia, CSF stagnation or both. Brain. 2004;127:947.
13 Silverberg GD, Mayo M, Saul T, et al. Alzheimer’s disease, normal-pressure hydrocephalus, and senescent changes in CSF circulatory physiology: a hypothesis. Lancet Neurol. 2003;2:506.
14 Ojemann RG, Fisher CM, Adams RD, et al. Further experience with the syndrome of “normal” pressure hydrocephalus. J Neurosurg. 1969;31:279.
15 Fisher CM. The clinical picture in occult hydrocephalus. Clin Neurosurg. 1977;24:270.
16 Relkin N, Marmarou A, Klinge P, et al. Diagnosing idiopathic normal-pressure hydrocephalus. Neurosurgery. 2005;57:S4.
17 Zaaroor M, Bleich N, Chistyakov A, et al. Motor evoked potentials in the preoperative and postoperative assessment of normal pressure hydrocephalus. J Neurol Neurosurg Psychiatry. 1997;62:517.
18 Corkill RG, Cadoux-Hudson TA. Normal pressure hydrocephalus: developments in determining surgical prognosis. Curr Opin Neurol. 1999;12:671.
19 Iddon JL, Pickard JD, Cross JJ, et al. Specific patterns of cognitive impairment in patients with idiopathic normal pressure hydrocephalus and Alzheimer’s disease: a pilot study. J Neurol Neurosurg Psychiatry. 1999;67:723.
20 Devito EE, Pickard JD, Salmond CH, et al. The neuropsychology of normal pressure hydrocephalus (NPH). Br J Neurosurg. 2005;19:217.
21 Bret P, Guyotat J, Chazal J. Is normal pressure hydrocephalus a valid concept in 2002? A reappraisal in five questions and proposal for a new designation of the syndrome as “chronic hydrocephalus”. J Neurol Neurosurg Psychiatry. 2002;73:9.
22 van Zagten M, Kessels F, Boiten J, et al. Interobserver agreement in the assessment of cerebral atrophy on CT using bicaudate and sylvian-fissure ratios. Neuroradiology. 1999;41:261.
23 Krauss JK, Droste DW, Vach W, et al. Cerebrospinal fluid shunting in idiopathic normal-pressure hydrocephalus of the elderly: effect of periventricular and deep white matter lesions. Neurosurgery. 1996;39:292.
24 Tullberg M, Jensen C, Ekholm S, et al. Normal pressure hydrocephalus: vascular white matter changes on MR images must not exclude patients from shunt surgery. AJNR Am J Neuroradiol. 2001;22:1665.
25 Akiguchi I, Ishii M, Watanabe Y, et al. Shunt-responsive parkinsonism and reversible white matter lesions in patients with idiopathic NPH. J Neurol. 2008;255:1392.
26 Marmarou A, Bergsneider M, Klinge P, et al. The value of supplemental prognostic tests for the preoperative assessment of idiopathic normal-pressure hydrocephalus. Neurosurgery. 2005;57:S17.
27 Vanneste J, Augustijn P, Dirven C, et al. Shunting normal-pressure hydrocephalus: do the benefits outweigh the risks? A multicenter study and literature review. Neurology. 1992;42:54.
28 Walchenbach R, Geiger E, Thomeer RT, et al. The value of temporary external lumbar CSF drainage in predicting the outcome of shunting on normal pressure hydrocephalus. J Neurol Neurosurg Psychiatry. 2002;72:503.
29 Czosnyka Z, Czosnyka M, Owler B, et al. Clinical testing of CSF circulation in hydrocephalus. Acta Neurochir Suppl. 2005;95:247.
30 Boon AJ, Tans JT, Delwel EJ, et al. Dutch normal-pressure hydrocephalus study: prediction of outcome after shunting by resistance to outflow of cerebrospinal fluid. J Neurosurg. 1997;87:687.
31 Ushewokunze S, Haja Mydin HN, Prasad R, et al. Lumbar subcutaneous shunt: a novel technique for therapeutic decision making in normal pressure hydrocephalus (NPH) and benign intracranial hypertension (BIH). Br J Neurosurg. 2008;22:678.
32 Marmarou A. A Theoretical Model and Experimental Evaluation of the Cerebrospinal Fluid System. Philadelphia, PA: Drexel University; 1973.
33 Marmarou A, Shulman K, Rosende RM. A nonlinear analysis of the cerebrospinal fluid system and intracranial pressure dynamics. J Neurosurg. 1978;48:332.
34 Ekstedt J. CSF hydrodynamic studies in man. 1. Method of constant pressure CSF infusion. J Neurol Neurosurg Psychiatry. 1977;40:105.
35 Ekstedt J. CSF hydrodynamic studies in man. 2 . Normal hydrodynamic variables related to CSF pressure and flow. J Neurol Neurosurg Psychiatry. 1978;41:345.
36 Borgesen SE, Gjerris F. The predictive value of conductance to outflow of CSF in normal pressure hydrocephalus. Brain. 1982;105:65.
37 Boon AJ, Tans JT, Delwel EJ, et al. Dutch Normal-Pressure Hydrocephalus Study: the role of cerebrovascular disease. J Neurosurg. 1999;90:221.
38 Malm J, Kristensen B, Karlsson T, et al. The predictive value of cerebrospinal fluid dynamic tests in patients with the idiopathic adult hydrocephalus syndrome. Arch Neurol. 1995;52:783.
39 Marmarou A, Foda MA, Bandoh K, et al. Posttraumatic ventriculomegaly: hydrocephalus or atrophy? A new approach for diagnosis using CSF dynamics. J Neurosurg. 1996;85:1026.
40 Klinge P, Fischer J, Brinker T, et al. PET and CBF studies of chronic hydrocephalus: a contribution to surgical indication and prognosis. J Neuroimaging. 1998;8:205.
41 Davson H, Hollingsworth G, Segal MB. The mechanism of drainage of the cerebrospinal fluid. Brain. 1970;93:665.
42 Albeck MJ, Borgesen SE, Gjerris F, et al. Intracranial pressure and cerebrospinal fluid outflow conductance in healthy subjects. J Neurosurg. 1991;74:597.
43 Higgins JN, Owler BK, Cousins C, et al. Venous sinus stenting for refractory benign intracranial hypertension. Lancet. 2002;359:228.
44 Pickard JD, Czosnyka Z, Czosnyka M, et al. Coupling of sagittal sinus pressure and cerebrospinal fluid pressure in idiopathic intracranial hypertension—a preliminary report. Acta Neurochir Suppl. 2009;105:283.
45 Avezaat C, Eijndhoven JHM. Cerebrospinal Fluid Pulse Pressure and Craniospinal Dynamics. A Theoretical, Clinical and Experimental Study [thesis]. The Hague: Academic Hospital Rotterdam and Erasmus University; 1984.
46 Raabe A, Czosnyka M, Piper I, et al. Monitoring of intracranial compliance: correction for a change in body position. Acta Neurochir (Wien). 1999;141:31.
47 Czosnyka M, Czosnyka Z, Momjian S, et al. Cerebrospinal fluid dynamics. Physiol Meas. 2004;25:R51.
48 Ursino M, Di Giammarco P. A mathematical model of the relationship between cerebral blood volume and intracranial pressure changes: the generation of plateau waves. Ann Biomed Eng. 1991;19:15.
49 Hoffmann O. CSF dynamics: Integration of pulsatory components and autoregulation into mathematical model. In: Ishii S, Nagai H, Brock M, editors. Intracranial Pressure V. Berlin: Springer-Verlag; 1983:169-173.
50 Czosnyka M, Piechnik S, Richards HK, et al. Contribution of mathematical modelling to the interpretation of bedside tests of cerebrovascular autoregulation. J Neurol Neurosurg Psychiatry. 1997;63:721.
51 Rekate HL, Brodkey JA, Chizeck HJ, et al. Ventricular volume regulation: a mathematical model and computer simulation. Pediatr Neurosci. 1988;14:77.
52 Sorek S, Bear J, Karni Z. A non-steady compartmental flow model of the cerebrovascular system. J Biomech. 1988;21:695.
53 Lundberg N. Continuous recording and control of ventricular fluid pressure in neurosurgical practice. Acta Psychiatr Scand Suppl. 1960;36:1.
54 Gjerris F, Borgesen SE. Pathophysiology of CSF circulation. In: Crockard A, Hayward A, Hoff JT, editors. Neurosurgery. The Scientific Basis of Clinical Practice. Oxford: Blackwell Scientific; 1992:146-174.
55 Pickard JD, Teasdale G, Matheson M, et al. Intraventricular pressure waves—the best predictive test for shunting in normal pressure hydrocephalus. In: Shulman K, Marmarou A, Miller JD, et al, editors. Intracranial Pressure IV. Berlin: Springer-Verlag; 1980:498-500.
56 Owler BK, Fong KC, Czosnyka Z. Importance of ICP monitoring in the investigation of CSF circulation disorders. Br J Neurosurg. 2001;15:439.
57 Kim DJ, Czosnyka Z, Keong N, et al. Index of cerebrospinal compensatory reserve in hydrocephalus. Neurosurgery. 2009;64:494.
58 Schuhmann MU, Sood S, McAllister JP, et al. Value of overnight monitoring of intracranial pressure in hydrocephalic children. Pediatr Neurosurg. 2008;44:269.
59 Eide PK. Intracranial pressure parameters in idiopathic normal pressure hydrocephalus patients treated with ventriculo-peritoneal shunts. Acta Neurochir (Wien). 2006;148:21.
60 Czosnyka ZH, Czosnyka M, Whitfield PC, et al. Cerebral autoregulation among patients with symptoms of hydrocephalus. Neurosurgery. 2002;50:526.
61 Petrella G, Czosnyka M, Keong N, et al. How does CSF dynamics change after shunting? Acta Neurol Scand. 2008;118:182.
62 Borgesen SE, Albeck MJ, Gjerris F, et al. Computerized infusion test compared to steady pressure constant infusion test in measurement of resistance to CSF outflow. Acta Neurochir (Wien). 1992;119:12.
63 Czosnyka M, Batorski L, Laniewski P, et al. A computer system for the identification of the cerebrospinal compensatory model. Acta Neurochir (Wien). 1990;105:112.
64 Katzman R, Hussey F. A simple constant-infusion manometric test for measurement of CSF absorption. I. Rationale and method. Neurology. 1970;20:534.
65 Maksymowicz W, Czosnyka M, Koszewski W, et al. The role of cerebrospinal compensatory parameters in the estimation of functioning of implanted shunt system in patients with communicating hydrocephalus (preliminary report). Acta Neurochir (Wien). 1989;101:112.
66 Taylor R, Czosnyka Z, Czosnyka M, et al. A laboratory model of testing shunt performance after implantation. Br J Neurosurg. 2002;16:30.
67 Czosnyka Z, Czosnyka M, Richards HK, et al. Laboratory testing of hydrocephalus shunts—conclusion of the U.K. Shunt Evaluation Programme. Acta Neurochir (Wien). 2002;144:525.
68 Aschoff A, Kremer P, Benesch C, et al. Overdrainage and shunt technology. A critical comparison of programmable, hydrostatic and variable-resistance valves and flow-reducing devices. Childs Nerv Syst. 1995;11:193.
69 Czosnyka Z, Czosnyka M, Richards HK, et al. Posture-related overdrainage: comparison of the performance of 10 hydrocephalus shunts in vitro. Neurosurgery. 1998;42:327.
70 Bergsneider M, Black PM, Klinge P, et al. Surgical management of idiopathic normal-pressure hydrocephalus. Neurosurgery. 2005;57:S29.
71 Greenberg MS. Hydrocephalus. In Greenberg MS, editor: Handbook of Neurosurgery, 6th ed, New York: Thieme, 2006.
72 Boon AJ, Tans JT, Delwel EJ, et al. Dutch Normal-Pressure Hydrocephalus Study: randomized comparison of low- and medium-pressure shunts. J Neurosurg. 1998;88:490.
73 Gangemi M, Maiuri F, Buonamassa S, et al. Endoscopic third ventriculostomy in idiopathic normal pressure hydrocephalus. Neurosurgery. 2004;55:129.
74 Drake JM, Kestle JR, Milner R, et al. Randomized trial of cerebrospinal fluid shunt valve design in pediatric hydrocephalus. Neurosurgery. 1998;43:294.
75 Portnoy HD, Schulte RR, Fox JL, et al. Anti-siphon and reversible occlusion valves for shunting in hydrocephalus and preventing post-shunt subdural hematomas. J Neurosurg. 1973;38:729.
76 Lavinio A, Harding S, Van Der Boogaard F, et al. Magnetic field interactions in adjustable hydrocephalus shunts. J Neurosurg Pediatr. 2008;2:222.
77 Malm J, Lundkvist B, Eklund A, et al. CSF outflow resistance as predictor of shunt function. A long-term study. Acta Neurol Scand. 2004;110:154.
78 Williams MA, Razumovsky AY, Hanley DF. Evaluation of shunt function in patients who are never better, or better than worse after shunt surgery for NPH. Acta Neurochir Suppl. 1998;71:368.
79 Eklund A, Koskinen LO, Malm J. Features of the Sinushunt and its influence on the cerebrospinal fluid system. J Neurol Neurosurg Psychiatry. 2004;75:1156.
80 Black PM, Ojemann RG, Tzouras A. CSF shunts for dementia, incontinence, and gait disturbance. Clin Neurosurg. 1985;32:632.
81 McGirt MJ, Woodworth G, Coon AL, et al. Diagnosis, treatment, and analysis of long-term outcomes in idiopathic normal-pressure hydrocephalus. Neurosurgery. 2005;57:699.
82 Keong N, Wilby M, Owler BK, et al. Profiles of shunt-responders in normal pressure hydrocephalus. Proceedings of the 148th Meeting of the Society of British Neurological Surgeons. Br J Neurosurgery. 2006;20:437.
83 Zemack G, Romner B. Adjustable valves in normal-pressure hydrocephalus: a retrospective study of 218 patients. Neurosurgery. 2002;51:1392.
84 Raftopoulos C, Deleval J, Chaskis C, et al. Cognitive recovery in idiopathic normal pressure hydrocephalus: a prospective study. Neurosurgery. 1994;35:397.
85 Savolainen S, Hurskainen H, Paljarvi L, et al. Five-year outcome of normal pressure hydrocephalus with or without a shunt: predictive value of the clinical signs, neuropsychological evaluation and infusion test. Acta Neurochir (Wien). 2002;144:515.