Chapter 13 Circadian Rhythm of Restless Legs Syndrome
Circadian Variation in Restless Legs Syndrome
One of the defining features of the restless legs syndrome (RLS) is that it shows a notable circadian rhythm with symptoms intensifying later in the day (see Chapter 15). This holds true at least for those who have normal sleep-wake schedules. Those who do shift work, have irregular sleep-wake schedules, or those adjusting to jet lag may not show this pattern. This circadian pattern was noted by Willis in his presumptive case1 and established as a diagnostic feature by Ekbom.2
However, it was not initially clear that this circadian pattern was due to a specific circadian factor rather than merely reflecting the fact that, later in the day, individuals typically reduce activity and begin to relax. This changed state could itself be the cause of the circadian pattern. In two studies, Trenkwalder, Hening, and colleagues3,4 showed that there was a true circadian factor to RLS (Fig. 13-1). These investigators used a semiconstant routine study in which hospitalized patients were observed approximately every 3 hours during which they attempted to stay motionless while seated in bed for 1 hour or longer (a modified suggested immobilization test [mSIT]).5–7 Both subjective (intensity of symptoms) and objective (periodic limb movements [PLM] or motor restlessness) features were measured during 2 days of normal sleep-wake cycles followed by 36 hours of sustained wakefulness. During all days as clock time increased from morning (9:00 A.M.) to early morning the following day (2:00 A.M.), both subjective and objective measures of RLS increased. During continued sleep deprivation, the patients’ discomforts and measures of PLM and motor activity decreased during the day (9:00 A.M. to 3:00 p.m.), before beginning to increase again (6:00 P.M.). Based on these findings, it was concluded that the symptoms and associated motor findings of RLS follow a quite similar circadian pattern with maximum symptoms occurring during the early part of the night.
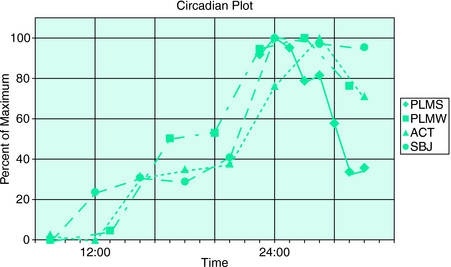
FIGURE 13-1. Circadian plot. Normalized values of the RLS measures are plotted against clock time from 8:00 A.M. through midnight (24:00) to 8:00 A.M. ACT, activity counts (measure of recorded motor activity); PLMS, periodic limb movements in sleep; PLMW, periodic limb movements when awake; SBJ, subjective leg discomfort measured with visual analog scale. Values were derived from modified suggested immobilization tests when subjects were awake (PLMW, ACT, SBJ) and from sleep recordings during sleep (PLMS). Hours represent beginning of an SIT period or beginning of an hour of enumerating PLMS. Data were obtained from two studies carried out over 72 hours during constant monitoring.3,4 Hourly averages from the first study3 from 11:00 P.M. to 7:00 A.M. are plotted as a percentage of the maximum (94.9/hr in the period from midnight to 1:00 A.M.). Mean value for 8 subjects and 2-night PSG. Other mV measures are plotted as percent from minimum (0%) to maximum (100%). PLMW is from the first study3; ACT and SBJ are from the second study.4 PLMW is minimum of 13.8/hr (9:00 A.M.), maximum of 91.5/hr (2:00). ACT is minimum of 124.9 counts (12:00), maximum of 447.8 (3:00). SBJ is minimum of 2.96 (on 0-to-10 scale averaged over five determinations per 1-hour mSIT) at 9:00 A.M., maximum of 6.04 (at midnight [24:00]). The values of PLMW, ACT, and SBJ were taken by averaging daytime SITs (or mSITs) from the first 2 days of the study; values from hours between 11:00 P.M. and 6:00 A.M. were taken from the third night of total sleep deprivation. There were nine subjects whose measures were averaged in the second study.4
The patients in these two studies were also monitored with rectal thermometers to establish the timing of their internal rhythms. The nadir of this rhythm—which occurs at approximately 4:00 A.M. to 7:00 A.M., shortly before waking in most individuals—is denominated as circadian time 0 or phase 0 degrees.8 The timing of the temperature minimum in these subjects occurred on average between 4:00 A.M. and 5:00 A.M. in clock time, not notably different from normal subjects of the same age. This made a major deficit in the system that regulates circadian phase unlikely. Comparing the temperature cycle to that of RLS features, it was evident that for these patients, RLS severity was greatest during the falling phase of core temperature, and that around the time of temperature nadir, their RLS began to decrease.
Michaud and colleagues9 confirmed the same circadian pattern of RLS severity. They noted—using a similar protocol and repeated episodes of constrained rest—that even normal subjects seemed to show a pattern of discomfort and movement that paralleled that of RLS patients, although to a considerably lesser degree. This observation echoes the finding that pain perception, too, is most intense during nighttime hours.10 In addition to level of discomfort, the rapidity with which symptoms develop shows the same circadian pattern in RLS patients: when symptoms peak, they develop more rapidly.11
These studies have indicated that RLS does have a true circadian rhythm, and that RLS is most severe before circadian time 0 (core temperature nadir). In addition, there is no evidence to date for an abnormality of circadian timing in RLS and even normal persons may show a similar rhythm of sensory discomfort and motor activity at rest. These findings have led to the questions of how RLS symptoms are related to the circadian mechanism and which features of downstream (circadian output) control are disordered in RLS.
It has been noted that both iron and dopamine (see Chapters 9, 10, and 11), potential mediators of RLS, have a circadian rhythm in which circulating levels are lowest during the evening and night-time hours when RLS symptoms are most intense.
Endocrine Markers of Circadian Phase in Restless Legs Syndrome
Potential abnormalities of circadian function have also been evaluated by means of investigation of the endocrine function across the 24-hour cycle. It is known that the pattern of secretion of many hormones across the day and night follows a circadian pattern.12 Furthermore, the effects of neuroendocrine secretion on sleep and, conversely, of sleep deprivation on endocrine secretion have also long been established.12 Thus, it can be expected that the circadian pattern of secretion of certain hormones, particularly of those that are markers of circadian rhythms or are directly influenced by dopaminergic mechanisms, might be altered in RLS. We briefly outline in the following paragraphs the circadian patterns of secretion of several hormones in RLS, both in the untreated and treated conditions as well as after treatment.
Melatonin and Restless Legs Syndrome
As the main marker of the circadian phase, the role of melatonin in RLS has been studied by investigators. Tribl and colleagues13 analyzed the urinary excretion of 6-OH melatonin-sulfate both during the daytime (7:00 A.M. to 10:00 P.M.) and the nighttime (10:00 P.M. to 7:00 A.M.) in 15 patients with idiopathic RLS and 11 control subjects by means of radioimmunoassay. For both groups, nighttime excretion was greater than daytime excretion. However, no difference between patients and control subjects could be found either in the daytime or in the nighttime. This study had major limitations, because of the poor time resolution achieved by urinary metabolites.
Michaud and colleagues9 investigated the circadian pattern of salivary melatonin in a study that involved seven patients with RLS and seven age- and gender-matched healthy control subjects. The experimental protocol consisted of an all-night sleep study followed by a 28-hour modified constant routine procedure.9 Because it was not possible to keep RLS patients in bed for 28 consecutive hours, the constant routine protocol was divided into 14 episodes of 2 hours. During the first 20 minutes, subjects had to first evaluate their subjective vigilance and provide a salivary sample before they were free to walk around (free-moving period). Afterward, they were confined to a reclining chair for 40 minutes, followed by another 20-minute free-moving period, and then by a 40-minute suggested immobilization test (SIT).5 As described previously by the same group,14 during the SIT, subjects were reclined at a 45-degree angle with their legs outstretched and were instructed to avoid voluntary movements for the duration of the test and to quantify their level of leg discomfort every 5 minutes on a visual analog scale. The SIT was performed under dim light condition. A significant circadian variation in leg discomfort and PLM was found for both groups. However, no differences were seen in the circadian rhythm of melatonin between patients and control subjects, although the profiles of leg discomfort and PLM were significantly correlated with those of subjective vigilance, core body temperature, and salivary melatonin (Fig. 13-2). However, the changes in melatonin secretion were the only ones that preceded the increases in sensory and motor symptoms in RLS patients. Although the RLS symptoms started to worsen at the time of onset of melatonin secretion, the acrophase of RLS symptoms was reached approximately 2 hours after the peak of melatonin secretion. Accordingly, the authors suggested that melatonin secretion might be driving the increase of RLS symptoms in the evening and during the night, thus raising the possibility that melatonin would play a direct role in the pathophysiology of RLS. Physiological concentrations of melatonin are known to exert an inhibitory effect on dopamine secretion in several areas of the mammalian central nervous system.15 Furthermore, melatonin is also known to suppress postsynaptic N-methyl-D-aspartate receptor–mediated excitatory responses of striatal neurons to glutamate.16 On the basis of these findings, it is reasonable to think that the increase in melatonin secretion in the evening may facilitate the occurrence of RLS symptoms by decreasing the activity of the central dopamine systems.
The reverse is also possible, as dopaminergic medication might affect melatonin function, a key element of the circadian system. Thus, the effects of dopaminergic treatment on plasma melatonin secretion was the subject of an exploratory study by Garcia-Borreguero and colleagues.17 Eight previously untreated patients diagnosed with idiopathic RLS were treated under open conditions for 3 weeks with 400 mg L-DOPA (+100 mg carbidopa), and dim light melatonin onset (DLMO), a marker of circadian phase, was determined before and after treatment. Compared with baseline, treated patients had an earlier time of DLMO (6:50 P.M. +/− 0:55 vs. 9:00 P.M. +/− 1:20; p < .05) (Fig. 13-3). Interestingly, despite the brevity of the treatment period, some of the patients showed early signs of augmentation and the anticipation of time of DLMO was more marked in this subgroup. A positive correlation was observed between changes of DLMO, sleep latency, and time of onset of symptoms following treatment with L-DOPA. Taken together, preliminary evidence shows no major differences between untreated patients and healthy control subjects in the patterns of secretion of melatonin. However, this work suggests that dopaminergics can cause an advance in the time of onset of RLS symptoms. Furthermore, this work confirms that the time of onset of RLS symptoms is related to the time of DLMO.
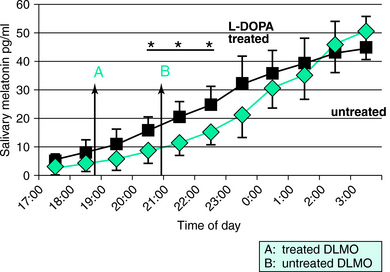
FIGURE 13-3. Salivary levels of melatonin in a group of eight restless legs syndrome (RLS) patients in untreated and in L-DOPA–treated conditions. The study was done under semicontrolled circadian conditions between 5:00 P.M. and 3:00 A.M.17 Differences between both groups were statistically significant at 8:00 P.M., 9:00 P.M., and 10:00 P.M. Arrows A and B show the time of dim light melatonin onset (DLMO), which is the time at which a salivary concentration of 10 pg/ml is reached. Taken together, the data suggest an earlier secretion of melatonin in treated RLS patients (see text for discussion). *p < .05.
Circadian Variations in Dopaminergic Activity
Although the pathophysiology of RLS is incompletely known, it seems likely that a dopaminergic dysfunction plays a central role in the cause of RLS18,19 (see also Chapter 31). Accordingly, the circadian variations of sensory and motor symptoms of RLS should be paralleled by a circadian variation in dopaminergic function.20 Both animal21,22 and human23,24 studies suggest the existence of circadian variations in dopaminergic activity.25–31 Human data show a distinct circadian variation, with a pattern characterized by an increase in the morning and a nadir in the late evening/night.29–31
Alternatively, the circadian pattern might not be controlled by the dopaminergic system itself but rather by some other process that modulates it. Thus, tetrahydrobiopterin (TH-biopterin), a cofactor of tryptophan and tyrosine hydroxylase,32 is decreased in Segawa’s disease,33 another neurological disorder with a distinct circadian pattern of severity. TH-biopterin brain levels show a diurnal pattern that parallels dopamine production.22,34 So far, cerebrospinal fluid (CSF) levels of TH-biopterins have been found to be higher in RLS patients than in control subjects when collected in the morning, whereas the circadian changes in this factor as well as in the HVA/5-HIAA ratio and in 3-O-methyldopa levels showed much greater changes in the RLS patients than control subjects with all levels higher in the morning.35,36 This finding suggests that the dopamine abnormality in RLS may include an exaggeration of the normal circadian rhythm of dopamine system function. Iron, another cofactor of tyrosine hydroxylase,32 is low in the CSF of RLS patients; this is particularly true of those with early-onset RLS.37,38 Younger age at onset is associated with lower CSF ferritin values, and ferritin levels also vary with time of day in RLS patient CSF.38 Serum iron shows a marked circadian variation with a low point in the evening and early night,39 a time that coincides with maximal severity of symptoms. Thus, circadian variation in serum iron parallels the circadian variation of dopamine.
Neuroendocrine Variations
If dopaminergic tuberoinfundibular systems are involved in RLS, circadian changes in dopaminergic function should be followed by parallel changes in the endocrine output under dopaminergic control. Thus, Wetter and colleagues40 measured plasma levels of prolactin (PRL), growth hormone (GH), and cortisol every 20 minutes for 24 hours in 10 male treatment-naive RLS patients with mild to moderate RLS and in 8 age-matched healthy control subjects. No differences in plasma levels or amplitude or frequency of the pulses of any of these hormones were seen between both groups; neither were there differences in sleep parameters between the groups apart from a higher PLMS arousal index in RLS patients compared with the control subjects. Because cortisol is considered to be a stable marker of the sleep-wake cycle,12 the absence of a phase advance or any other circadian dysfunction in the rhythm of cortisol was considered to be in line with the lack of dysfunction in the circadian rhythm of temperature in untreated RLS patients. However, the major limitation in this study was the 20-minute sampling interval, as it could be too long for adequate detection of pulses. The authors concluded that a dysfunction of the dopaminergic system in RLS affecting the release of PRL and human GH from the pituitary gland was unlikely.
Neuroendocrine responses to dopaminergic drugs provide an additional perspective to investigate the function of the dopamine system. Thus, PRL and GH responses to various dopaminergic drugs have been used in the past to investigate the sensitivity of dopaminergic receptors to various disorders, particularly in Parkinson’s disease and multiple system atrophy.41–44 Under normal circumstances, the administration of dopaminergic drugs exerts inhibitory effects on the release of PRL45,46 and enhances the release of GH.47,48 However, in order to test possible changes in endocrine changes by means of dopaminergic stimulation, Garcia-Borreguero and colleagues17 performed an L-DOPA neuroendocrine challenge on 12 treatment-naive RLS patients and 12 healthy control subjects. The L-DOPA neuroendocrine challenge was performed on two occasions on each subject (11:00 A.M. and 11:00 P.M.), in a randomized order. On each occasion, subjects took 200 mg of L-DOPA (plus 50 mg carbidopa) by mouth. Blood was drawn 20 minutes and 5 minutes before administration of the drug, as well as at 15-minute intervals after the administration. Prechallenge DOPA plasma values of GH or PRL did not differ in the two subject groups. After the nighttime administration of L-DOPA, RLS patients selectively presented with a more pronounced inhibition of PRL release (Fig. 13-4) and increase in GH secretion compared with control subjects. PRL plasma levels were significantly correlated to the periodic limb movement index on the polysomnography. These results suggest the presence of hypersensitive dopamine postsynaptic receptors at night, which have been interpreted in the context of an increased amplitude of the circadian variation of dopaminergic function in RLS subjects compared with healthy control subjects (Fig. 13-4).
The L-DOPA neuroendocrine challenge was also performed on 22 patients with idiopathic RLS who had been treated for 15 months with L-DOPA at a mean dosage of 149 mg/day as their initial RLS therapy and on 14 control subjects.49 These patients also underwent an SIT, followed by a sleep study both before and after this course of treatment. At baseline, PRL plasma levels showed a more pronounced reduction following acute administration of L-DOPA in RLS patients than in control subjects. However, following long-term treatment with L-DOPA, patients showing augmentation showed a blunted PRL and GH response compared with baseline, suggesting a decrease in dopamine receptor sensitivity in RLS patients following long-term treatment with L-DOPA. This effect was particularly pronounced in patients undergoing augmentation. Thus, augmentation would appear to lead to a decreased circadian variation in dopaminergic function.
1. Willis T. Two Discourses Concerning the Soul of Brutes. London: Dring, Harper, and Leigh, 1683.
2. Ekbom KA. Restless legs: A clinical study. Acta Med Scand Suppl. 1945;158:1-122.
3. Trenkwalder C, Hening WA, Walters AS, et al. Circadian rhythm of periodic limb movements and sensory symptoms of restless legs syndrome. Mov Disord. 1999;14:102-110.
4. Hening WA, Walters AS, Wagner M, et al. Circadian rhythm of motor restlessness and sensory symptoms in the idiopathic restless legs syndrome. Sleep. 1999;22:901-912.
5. Michaud M, Paquet J, Lavigne G, et al. Sleep laboratory diagnosis of restless legs syndrome. Eur Neurol. 2002;48:108-113.
6. Michaud M. Is the suggested immobilization test the “gold standard” to assess restless legs syndrome? Sleep Med. 2006;7:541-543.
7. Michaud M, Dumont M, Paquet J, et al. Circadian variation of the effects of immobility on symptoms of restless legs syndrome. Sleep. 2005;28:843-846.
8. Moore-Ede MC, Czeisler CA, Richardson GS. Circadian timekeeping in health and disease. Part 1. Basic properties of circadian pacemakers. N Engl J Med. 1983;309:469-476.
9. Michaud M, Dumont M, Selmaoui B, et al. Circadian rhythm of restless legs syndrome: Relationship with biological markers. Ann Neurol. 2004;55:372-380.
10. Gobel H, Cordes P. Circadian variation of pain sensitivity in pericranial musculature. Headache. 1990;30:418-422.
11. Allen RP, Dean T, Earley CJ. Effects of rest-duration, time-of-day and their interaction on periodic leg movements while awake in restless legs syndrome. Sleep Med. 2005;6:429-434.
12. Van Cauter E. Diurnal and ultradian rhythms in human endocrine function: A minireview. Horm Res. 1990;34:45-53.
13. Tribl GG, Waldhauser F, Sycha T, et al. Urinary 6-hydroxy-melatonin-sulfate excretion and circadian rhythm in patients with restless legs syndrome. J Pineal Res. 2003;35:295-296.
14. Michaud M, Lavigne G, Desautels A, et al. Effects of immobility on sensory and motor symptoms of restless legs syndrome. Mov Disord. 2002;17:112-115.
15. Zisapel N. Melatonin-dopamine interactions: From basic neurochemistry to a clinical setting. Cell Mol Neurobiol. 2001;21:605-616.
16. Zisapel N, Laudon M. Inhibition by melatonin of dopamine release from rat hypothalamus: Regulation of calcium entry. Brain Res. 1983;272:378-381.
17. Garcia-Borreguero D, Serrano C, Larrosa O, et al. Circadian effects of dopaminergic treatment in restless legs syndrome. Sleep Med. 2004;5:413-420.
18. Paulus W, Schomburg ED. Dopamine and the spinal cord in restless legs syndrome: Does spinal cord physiology reveal a basis for augmentation? Sleep Med Rev. 2006;10:185-196.
19. Clemens S, Rye D, Hochman S. Restless legs syndrome: Revisiting the dopamine hypothesis from the spinal cord perspective. Neurology. 2006;67:125-130.
20. Garcia-Borreguero D, Larrosa O, de la Llave Y. Circadian aspects in the pathophysiology of the restless legs syndrome. Sleep Med. 2002;3(suppl):S17-S21.
21. Perlow MJ, Gordon EK, Ebert ME, et al. The circadian variation in dopamine metabolism in the subhuman primate. J Neurochem. 1977;28:1381-1383.
22. Shade R, Vick K, Ott T, et al. Circadian rhythms of dopamine and cholecystokinin in nucleus accumbens and striatum of rats—Influence of dopaminergic stimulation. Chronobiol Int. 1995;12:87-99.
23. Doran AR, Labarca R, Wolkowitz OM, et al. Circadian variation of plasma homovanillic acid levels is attenuated by fluphenazine in patients with schizophrenia. Arch Gen Psychiatry. 1990;47:558-563.
24. Sowers JR, Vlachakis N. Circadian variation in dopamine levels in man. J Endocrinol Invest. 1984;7:341-345.
25. Andre MH, Grignon S, Bruguerolle B. Circadian phase dependent pharmacokinetics of L-dopa, its main metabolites (3-OMD, HVA, DOPAC) and carbidopa in rats. Fundam Clin Pharmacol. 1996;10:350-355.
26. Andretic R, Hirsh J. Circadian modulation of dopamine receptor responsiveness in Drosophila melanogaster. Proc Natl Acad Sci U S A. 2000;97:1873-1878.
27. Ahlers L, Pastorova B, Solar P, et al. Daily rhythm in rat pineal catecholamines. Physiol Res. 1999;48:231-234.
28. Lal S, Tesfaye Y, Thavundayil JX, et al. Effect of time-of-day on the yawning response to apomorphine in normal subjects. Neuropsychobiology. 2000;41:178-180.
29. Kawano Y, Kawasaki T, Kawazoe N, et al. Circadian variations of urinary dopamine, norepinephrine, epinephrine and sodium in normotensive and hypertensive subjects. Nephron. 1990;55:277-282.
30. Lal S, Thavundayil J, Nair NP, et al. Effect of sleep deprivation on dopamine receptor function in normal subjects. J Neural Transm. 1981;50:39-45.
31. Hagan MM, Havel PJ, Seeley RJ, et al. Cerebrospinal fluid and plasma leptin measurement: Covariability with dopamine and cortisol in fasting humans. J Clin Endocrinol Metab. 1999;84:3579-3585.
32. Cooper JR, Bloom FE, Roth RH. The Biochemical Basis of Neuropharmacology. New York: Oxford University Press, 1991.
33. Segawa M, Hosaka A, Miyagawa F, et al. Hereditary progressive dystonia with marked diurnal fluctuations. Adv Neurol. 1976;14:215-233.
34. Mandel AJ, Bullard WP, Yellin JB, et al. The influence of d-amphetamine on rat brain striatal reduced biopterin concentration. J Pharmacol Exp Ther. 1980;213:574.
35. Earley CJ, Hyland K, Allen RP. CSF dopamine, serotonin, and biopterin metabolites in patients with restless legs syndrome. Mov Disord. 2001;16:144-149.
36. Earley CJ, Hyland K, Allen RP. Circadian changes in CSF dopaminergic measures in restless legs syndrome. Sleep Med. 2006;7:263-268.
37. Earley CJ, Connor JR, Beard JL, et al. Abnormalities in CSF concentrations of ferritin and transferrin in restless legs syndrome. Neurology. 2000;54:1698-1700.
38. Earley CJ, Connor JR, Beard JL, et al. Ferritin levels in the cerebrospinal fluid and restless legs syndrome: Effects of different clinical phenotypes. Sleep. 2005;28:1069-1075.
39. Scales WE, Vander AJ, Brown MB, et al. Human circadian rhythms in temperature, trace metals, and blood variables. J Appl Physiol. 1988;65:1840-1846.
40. Wetter TC, Trenkwalder C, Stiasny K, et al. Treatment of idiopathic and uremic restless legs syndrome with L-dopa—A double-blind cross-over study. Wien Med Wochenschr. 1995;145:525-527.
41. Friess E, Kuempfel T, Winkelmann J, et al. Increased growth hormone response to apomorphine in Parkinson disease compared with multiple system atrophy. Arch Neurol. 2001;58:241-246.
42. Llau ME, Durrieu G, Tran MA, et al. A study of dopaminergic sensitivity in Parkinson’s disease: Comparison in “de novo” and levodopa-treated patients. Clin Neuropharmacol. 1996;19:420-427.
43. Kostic VS, Susic V, Przedborski S, et al. Sleep EEG in depressed and nondepressed patients with Parkinson’s disease. J Neuropsychiatry Clin Neurosci. 1991;3:176-179.
44. Fabbrini G, Braun A, Mouradian MM, et al. Dopamine D-1 receptor agonist stimulation of prolactin secretion in man. J Neural Transm. 1988;71:159-163.
45. Chihara K, Kato Y, Maeda K, et al. Suppressive effect of L-DOPA on human prolactin release during sleep. Acta Endocrinol (Copenh). 1976;81:19-27.
46. Benker G, Jaspers C, Hausler G, et al. Control of prolactin secretion. Klin Wochenschr. 1990;68:1157-1167.
47. Martin JB. Neural regulation of growth hormone secretion. Med Clin North Am. 1978;62:327-336.
48. Muller EE. Nervous control of growth hormone secretion. Neuroendocrinology. 1973;11:338-369.
49. Garcia-Borreguero D, Egatz RSM, et al. Changes in dopamine receptor sensitivity during restless legs syndrome augmentation [abstract]. Sleep Med. 2005;6:S1.