26 Chronic obstructive pulmonary disease
Chronic obstructive pulmonary disease (COPD) is a disease state characterised by airflow limitation that is not fully reversible. The airflow limitation is usually both progressive and associated with an abnormal inflammatory response of the lungs to noxious particles or gases (GOLD, 2009).
COPD has been defined (National Institute for Health and Clinical Excellence, 2010) as:
Epidemiology
COPD is the fifth leading cause of death in the UK and the fourth in the world. It is expected to rise to third position by 2020. It is estimated that over 3 million people have the disease in the UK, with 2 million having undiagnosed COPD (National Clinical Guideline Centre, 2010). COPD is the largest single cause of lost working days in the UK. It is accountable for more than 10% of all hospital admissions and directly costs the NHS around £491 m/year. The burden of COPD on the UK healthcare system exceeds that of asthma and is outlined in Table 26.1.
Table 26.1 Annual morbidity and mortality from COPD (National Clinical Guideline Centre, 2010)
Pathology
The major pathological changes in COPD affect four different compartments of the lung and all are affected in most individuals to varying degrees (American Thoracic Society/European Respiratory Society Task Force, 2004).
Aetiology
Not all smokers go on to develop clinically significant COPD; genetic factors seem to modify each individual’s risk. The age at which an individual begins smoking, total pack-years smoked and current smoking status are predictive of COPD mortality. Passive exposure to cigarette smoke may also contribute to respiratory symptoms and COPD by increasing the lungs’ total burden of inhaled particles and gases (GOLD, 2009). Tobacco exposure is quantified in ‘pack-years’:
Additional risk factors include the natural ageing process of the lungs. Males are currently more at risk of developing chronic bronchitis, but as the number of women who smoke increases, the incidence of chronic bronchitis in females will also rise. The major risk factors are summarised in Table 26.2.
Risk factor | Comment |
---|---|
Smoking | Risk increases with increasing consumption but there is also large interindividual variation in susceptibility |
Age | Increasing age results in ventilatory impairment; most frequently related to cumulative smoking |
Gender | Male gender was previously thought to be a risk factor but this may be due to a higher incidence of tobacco smoking in men. Women have greater airway reactivity and experience faster declines in FEV1, so may be at more risk than men |
Occupation | The development of COPD has been implicated with occupations such as coal and gold mining, farming, grain handling and the cement and cotton industries |
Genetic factors | α1-Antitrypsin deficiency is the strongest single genetic risk factor, accounting for 1–2% of COPD. Other genetic disorders involving tissue necrosis factor and epoxide hydrolase may also be risk factors |
Air pollution | Death rates are higher in urban areas than in rural areas. Indoor air pollution from burning biomass fuel is also implicated as a risk factor, particularly in underdeveloped areas of the world |
Socio-economic status | More common in individuals of low socio-economic status |
Airway hyper-responsiveness and allergy | Smokers show increased levels of IgE, eosinophils and airway hyper-responsiveness but how these influence the development of COPD is unknown |
Pathophysiology
The pathogenic mechanisms and pathological changes described above lead to the physiological abnormalities of COPD: mucus hypersecretion, ciliary dysfunction, airflow limitation and hyperinflation, gas exchange abnormalities, pulmonary hypertension and systemic effects (American Thoracic Society/European Respiratory Society Task Force, 2004).
Airflow limitation and hyperinflation
This progressive destructive enlargement of the respiratory bronchioles, alveolar ducts and alveolar sacs is referred to as emphysema. Adjacent alveoli can become indistinguishable from each other, with two main consequences. The first is loss of available gas exchange surfaces, which leads to an increase in dead space and impaired gas exchange. The second consequence is the loss of elastic recoil in the small airways, vital for maintaining the force of expiration, which leads to a tendency for them to collapse, particularly during expiration. Increased thoracic gas volume and hyperinflation of the lungs result. The causes of airflow limitation in COPD are summarised in Box 26.1.
Clinical manifestations
Diagnosis
A diagnosis of COPD should be considered in any patient who has symptoms of cough, wheeze, regular sputum production or exertional dyspnoea and/or a history of exposure to COPD risk factors (see Table 26.2). Spirometry is then used to confirm the diagnosis. There is no single diagnostic test for COPD.
Investigations
Airflow obstruction is defined as:
Both UK and international COPD guidelines use spirometry to categorise the severity of COPD. These are summarised in Table 26.3. Testing should be carried out after a dose of inhaled bronchodilator to prevent overdiagnosis or overestimation of severity.
FEV1 | Severity (NICE) | Severity (GOLD) |
---|---|---|
Greater than 80% predicted | Stage I: Mild | |
50–80% predicted | Mild | Stage II: Moderate |
30–49% predicted | Moderate | Stage III: Severe |
Less than 30% predicted | Severe | Stage IV: Very severe |
(adapted from National Institute for Health and Clinical Excellence, 2010; GOLD, 2009)
At diagnosis and evaluation, patients may receive other investigations as outlined in Table 26.4.
Table 26.4 Additional investigations at the diagnosis of COPD
Investigation | Note |
---|---|
Chest X-ray | To exclude other pathologies |
Full blood count | To identify anaemia or polycythaemia |
Serial domiciliary peak flow measurements | To exclude asthma if there is a doubt about diagnosis |
α1-Antitrypsin | Particularly with early-onset disease or a minimal smoking/family history |
Transfer factor for carbon monoxide | To investigate symptoms that seem disproportionate to the spirometric impairment |
CT scan of the thorax | To investigate symptoms that seem disproportionate to the spirometric impairment |
To investigate abnormalities seen on the chest X-ray | |
To assess suitability for surgery | |
ECG | To assess cardiac status if features of cor pulmonale |
Echocardiogram | To assess cardiac status if features of cor pulmonale |
Pulse oximetry | To assess need for oxygen therapy |
If cyanosis or cor pulmonale is present or if FEV1 <50% of predicted value | |
Sputum culture | To identify organisms if sputum is persistently present and purulent |
Treatment
Stable COPD
The aims of treatment for patients with COPD are shown in Box 26.2 and the common therapeutic problems associated with COPD in Box 26.3. Drug treatment itself can only relieve symptoms; it does not modify the underlying pathology. Most patients with COPD are considered to have irreversible obstruction, in contrast to patients with asthma, but a significant number do seem to respond to bronchodilators.
Smoking cessation
Smoking is the most important factor in the development of obstructive airways disease. All COPD patients who are still smoking, regardless of age, should be encouraged to stop and be offered help to do so, at every opportunity. Unless contraindicated, all patients who are planning to stop smoking should be offered nicotine replacement therapy (NRT), varenicline or bupropion, as appropriate along with a support programme (NRT) (National Institute for Health and Clinical Excellence, 2010). Individually targeted advice may prove to be more successful in persuading individuals to give up, especially in those who are well.
Bronchodilators
Bronchodilators in COPD are used to reverse airflow limitation. As the degree of limitation varies widely, their effectiveness should be assessed in each patient using respiratory function tests and by assessing any subjective improvement reported by the patient. Patients may experience improvements in exercise tolerance or relief of symptoms such as wheeze and cough. Treatment options for the use of bronchodilators and inhaled corticosteroids (ICSs) are outlined in Fig. 26.1.
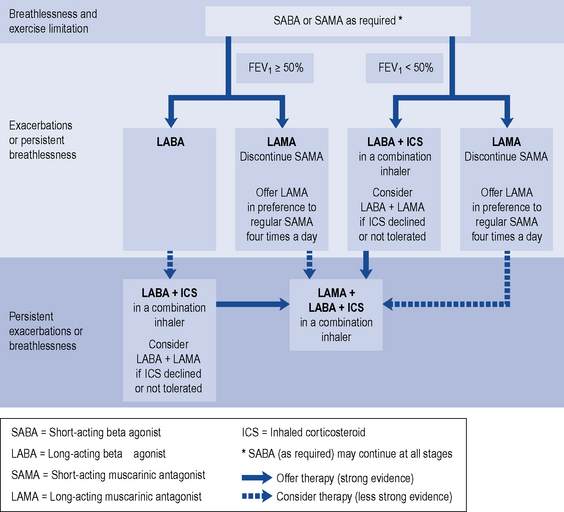
Fig. 26.1 Stepwise approach to the pharmacological management of chronic COPD (National Institute for Health and Clinical Excellence, 2010; with kind permission from National Institute for Health and Clinical Excellence).
Initial empiric therapy with short-acting bronchodilators, prescribed ‘when required’, for the relief of breathlessness and exercise limitation are recommended for initial use (National Institute for Health and Clinical Excellence, 2010). If patients remain breathless or have exacerbations despite short-acting bronchodilators then maintenance therapy is recommended, with:
Long-acting bronchodilators
Long-acting bronchodilators include LAMAs and LABAs. The only LAMA in the UK, tiotropium, has a 24-h duration of action and can reduce exacerbation rates, increase exercise tolerance and reduce rates of hospital admissions, though not the rate of decline in lung function. There is no strong evidence to favour either a LABA or LAMA for monotherapy (National Clinical Guideline Centre, 2010).
High-dose bronchodilators
Some patients with distressing or debilitating breathlessness despite maximal inhaled therapy may benefit from higher doses, either by inhaler or via a nebuliser. These patients should have their inhaled therapy optimised, possibly using a protocol as outlined in Box 26.4.
Box 26.4 Optimisation of high dose inhaled and nebulised therapy for patients with severe COPD
(adapted from Boe et al., 2001; National Institute for Health and Clinical Excellence, 2010; O’Driscoll, 1997)
Corticosteroids
Patients with COPD show a poor response to corticosteroids and have a largely steroid-resistant pattern of inflammation (Barnes, 2004). It is postulated that the oxidative stress of COPD inhibits the mechanism by which corticosteroids, acting through histone deacetylase, switch off activated inflammatory genes.
The long-term benefits of ICSs in COPD have only been shown in patients with moderate-to-severe disease, with an FEV1 less than 50% of predicted value and who are having exacerbations requiring treatment with antibiotics and oral corticosteroids (National Institute for Health and Clinical Excellence, 2010). A reduction in the number of exacerbations and a slowing of the decline in health status have been shown, but these have no effect on improving lung function and result in an increased risk of pneumonia.
Acute exacerbations of COPD
Patients with COPD suffer acute worsenings of the disease, referred to as acute exacerbations. These exacerbations can be spontaneous but are often precipitated by infection and lead to respiratory failure with hypoxaemia and retention of carbon dioxide. Many patients can be managed at home (see National Institute for Health and Clinical Excellence, 2010) but some will require admission to hospital.
Other treatment
Intravenous aminophylline can be considered, if there is an inadequate response to bronchodilators. The loading dose and maintenance dose required should be carefully chosen as these depend on various factors (see Chapter 25).
Oxygen therapy is necessary to improve hypoxia. In about a quarter of patients with COPD, a predisposition to carbon dioxide retention will be present. Administration of high concentrations of oxygen to these individuals can lead to an increase in retention of carbon dioxide and, thus, to respiratory acidosis. The widely held view that this effect is due to loss of hypoxic ventilatory drive has been questioned; a more complex process involving a mismatch between ventilation and perfusion is now thought to play a significant role. The goal is an initial oxygen saturation of between 88% and 92% to avoid respiratory acidosis but to allow enough oxygen to be administered to overcome potentially life-threatening hypoxia (O’Driscoll et al., 2008). The initial concentration used should be 24–28% and then titrated to oxygen saturation. Arterial blood gases should be monitored regularly.
Treatment of hypoxaemia and cor pulmonale
Domiciliary oxygen therapy
Continuous LTOT.
LTOT for at least 15 h/day has been shown to improve survival in patients with severe, irreversible airflow obstruction, hypoxaemia and peripheral oedema (MRC Working Party, 1981). LTOT can only be prescribed if specific conditions are met, as described in Box 26.5. The main aim of treatment is to achieve a PaO2 of at least 8 kPa without causing a rise in PaCO2 of more than 1 kPa, achieved by adjusting the oxygen flow rate. Before LTOT can be prescribed each individual must be assessed accordingly (see Box 26.5).
Patient care
Pulmonary rehabilitation
A multidisciplinary team should deliver these programmes and should include a minimum of 6 and a maximum of 12 weeks of physical exercise, disease education, psychological and social interventions (National Institute for Health and Clinical Excellence, 2010).
Stopping smoking
Members of the healthcare team can educate smokers about the dangers and actively encourage and motivate those who want to give up. Brief conversations between individuals and health professionals about stopping smoking are both effective and cost-effective in encouraging individuals to quit (National Institute for Health and Clinical Excellence, 2006).
Nicotine replacement
There is little research comparing the relative effectiveness of NRT products, but all seem to have similar success rates. Choice of product should be made on the number of cigarettes smoked (irrespective of the nicotine content), the smoker’s personal preference and tolerance to side effects. An individual is more likely to adhere to the cessation programme if using a product which suits him or her. The types of NRT available are summarised in Table 26.5.
Table 26.5 Comparison of selected nicotine replacement products
Formulation | Use and comments | Specific side effects |
---|---|---|
Patch: 24 h: 7, 14 and 21 mg; 16 h: 5, 10, 15 and 25 mg | One daily on clean, non-hairy, unbroken skin. Remove before morning (16 h) or next morning (24 h). Apply to fresh site or non-hairy skin, usually at the hip, trunk or upper arm. Should not be applied to broken skin | Local skin irritation and rashes, insomnia. Do not use with generalised skin disease |
Gum: 2 and 4 mg | Chew until taste is strong then rest gum between gum and cheek; chew again when taste has faded. Repeat this for 30 min or until taste dissipates. Avoid acidic drinks for 15 min before and during chewing the gum | Jaw ache, headache and dyspnoea. Mild burning sensation in the mouth and throat |
Sublingual tablet: 2 or 4 mg each | Rest under tongue until dissolved | |
Lozenge: 1, 2 or 4 mg each | Place between gum and cheek and allow to dissolve. Delivers slightly more nicotine than the equivalent gum | Nasal irritation, rhinorrhoea, sneezing, throat irritation and cough. This usually dissipates with continued use |
Inhalator : 10 mg per cartridge | Inhale as required. Helps to satisfy the hand-to-mouth ritual of using a cigarette which may help some people. The nicotine is absorbed through the mouth rather than the lungs. Use with caution in people with asthma | Nasal irritation, rhinorrhoea, sneezing, throat irritation and cough. This usually dissipates with continued use |
Nasal spray: 500 μcg per spray | One spray into each nostril as needed. More rapidly absorbed than other forms of NRT so often used for acute relief of cravings. Not recommended for people with nasal or sinus conditions, allergies or asthma |
Varenicline
Varenicline is an oral selective partial α4β2 agonist at the neuronal nicotinic acetylcholine receptor. Varenicline alleviates the symptoms of craving and withdrawal, whilst reducing the rewarding and reinforcing effects of smoking by preventing nicotine binding to α4β2 receptors. Trial evidence suggests use may result in a higher abstinence rate than either bupropion or NRT, although this may not be sustained after 12 months. Use is recommended as an option for smokers aged 18 or over wishing to quit, ideally in the context of a behavioural support programme (National Institute for Health and Clinical Excellence, 2007). Varenicline should be started 1–2 weeks before stopping smoking. The main side effects are nausea, vomiting, abnormal dreams and insomnia. Fears over a possible increased risk of depression and suicide have been allayed (Gunnell et al., 2009), although individuals who develop agitation, depressed mood or suicidal thoughts are required to seek prompt medical advice.
Use of inhaled therapy
Medication counselling needs to highlight the modes of action of the bronchodilators, particularly the more rapid onset of the β2-agonists to relieve breathlessness rather than the slower-acting anticholinergics. If inhaled steroids are prescribed, the importance of regular administration must be stressed. The incorrect use of any inhaler will lead to subtherapeutic dosing. The correct use of inhalers is, therefore, as vital in the management of COPD patients as it is for patients with asthma (see Chapter 25). The advantages and disadvantages of each type of inhaler device are summarised in Table 26.6.
Domiciliary oxygen therapy
Patients using domiciliary oxygen are followed up to provide education and support, to assess the oxygen saturation of the patient and to assess the suitability of the delivery device for ambulatory oxygen if provided. Suitable devices have been suggested (National Institute for Health and Clinical Excellence, 2010) depending on the amount of time required for use (Table 26.7).
Table 26.7 Oxygen delivery for ambulatory oxygen therapy (National Institute for Health and Clinical Excellence, 2010)
How long is the oxygen used by the patient? | Best type of delivery device |
---|---|
Less than 90 min | Small cylinder |
90 min to 4 h | Small cylinder with oxygen-conserving device |
More than 4 h | Liquid oxygen |
More than 30 min, with flow rates greater than 2 L/min | Liquid oxygen |
Answers
Answers
There is no evidence that use of mucolytics at this acute stage will be of benefit.
Answers
Another option would be to try varenicline. This may be more effective in achieving continuous abstinence (National Institute for Health and Clinical Excellence, 2007) than either nicotine replacement therapy or bupropion. Varenicline should be started 1–2 weeks before Mrs SS’s quit date and is continued for a total of 12 weeks, although an additional 12 weeks’ therapy may be required. Bupropion is contraindicated as this may increase Mrs SS’s risk of seizures.
In all cases, pharmacological therapy should also be offered as appropriate.
American Thoracic Society/European Respiratory Society Task Force. Standards for the Diagnosis and Management of Patients with COPD Version 1.2. New York: American Thoracic Society, 2004. [updated 8 September 2005]. Available at: http://www.thoracic.org/go/copd
Barnes P.J. Corticosteroid resistance in airway disease. Proc. Am. Thorac. Soc.. 2004;1:264-268.
Boe J., Dennis J.H., O’Driscoll B.R., for the European Respiratory Society Task Force. European Respiratory Society guidelines on the use of nebulizers. Eur. Respir. J.. 2001;18:228-242. Available at http://erj.ersjournals.com/cgi/content/full/18/1/228
GOLD. Global Strategy for the Diagnosis, Management, and Prevention of Chronic Obstructive Pulmonary Disease. Medical Communication Resources Inc, 2009. Available at: http://www.goldcopd.org/Guidelineitem.asp?l1=2&l2=1&intId=2003
Gunnell D., Irvine D., Wise L., et al. Varenicline and suicidal behaviour: a cohort study based on data from the general practice research database. Br. Med. J.. 2009;b3805:339.
MRC Working Party. Long-term domiciliary oxygen therapy in chronic hypoxic cor pulmonale complicating chronic bronchitis and emphysema. Lancet. 1981;1:681-686.
National Clinical Guideline Centre. Chronic Obstructive Pulmonary Disease: Management of Chronic Obstructive Pulmonary Disease in Adults in Primary and Secondary Care. London: National Clinical Guideline Centre, 2010. Available at: http://guidance.nice.org.uk/CG101/Guidance/pdf/English
National Institute for Health and Clinical Excellence. Brief Interventions and Referral for Smoking Cessation in Primary Care and Other Settings. London: NICE, 2006. Available at: http://www.nice.org.uk/nicemedia/pdf/SMOKING-ALS2_FINAL.pdf Public Health Intervention Guidance 1
National Institute for Health and Clinical Excellence. Varenicline for Smoking Cessation. London: NICE, 2007. Available at: http://www.nice.org.uk/TA123 Technology Appraisal 123
National Institute for Health and Clinical Excellence. Chronic Obstructive Pulmonary Disease. London: NICE, 2010. Available at: http://www.nice.org.uk/guidance/index.jsp?action=byID&o=13029 Clinical Guideline 101
O’Driscoll B.R. Nebulisers for chronic obstructive pulmonary disease. Thorax. 1997;52(Suppl. 2):S49-S52.
O’Driscoll B.R., Howard L.S., Davison A.G., on behalf of the British Thoracic Society Emergency Oxygen Guideline Development Group. Guideline for emergency oxygen use in adult patients. Thorax. 2008;63(Suppl. VI):vi69-vi73. doi:10.1136/thx.2008.102947
Barnes P., Shapiro S.D., Pauwels R.A. Chronic obstructive pulmonary disease: molecular and cellular mechanisms. Eur. Respir. J.. 2003;22:672-688.
Braman S. Chronic cough due to acute bronchitis: ACCP evidence-based clinical practice guidelines. Chest. 2006;129(Suppl. 1):95S-103S.
Henningfield J.E., Fant R.V., Buchalter A.R., et al. Pharmacotherapy for nicotine dependence. CA Cancer J. Clin.. 2005;55:281-299.
Molyneux M. ABC of smoking cessation: nicotine replacement therapy. Br. Med. J.. 2004;328:454-456.
Morgan M.D., Britton J.R. Chronic obstructive pulmonary disease 8: non-pharmacological management of COPD. Thorax. 2003;58:453-457.
Srivastava P., Currie G.P., Britton J. ABC of chronic obstructive pulmonary disease: smoking cessation. Br. Med. J.. 2006;332:1324-1326.