24 Cervical Stenosis: Radiculopathy – Review of Concepts, Surgical Techniques, and Outcomes
KEY POINTS
Introduction
Cervical spondylosis is a progressive degenerative process resulting in pathologic changes in the intervertebral discs and surrounding structures1. Such changes include intervertebral disc protrusion, osteophyte formation, and hypertrophy of the lamina, ligaments, and hypophyseal joints.2,3 Clinical onset can begin as early as the third decade, with progression continuing into the eighth decade.1 Radiographic signs of spondylosis are evident in 50% of the population by the fifth decade, while prevalence is estimated at 98% for people over 70.4 Secondary myelopathy is considered to be the most common cause of spinal cord dysfunction in patients over 55.5
The effects of spondylosis can range from subclinical symptoms to primary root impingement (radiculopathy) and myelopathy. Proper diagnosis depends on meticulous history-taking, thorough physical examination, and the use of appropriate imaging, neurophysiologic, and laboratory tests. The natural history of cervical spondylosis is not well understood6, and treatment of spondylosis includes both conservative medical management and surgical intervention. A variety of surgical procedures have been well described including anterior and posterior approaches with or without fusion. This chapter reviews the relevant anatomy, pathophysiology, symptomatology, diagnosis, natural history, and management of cervical spondylosis.
Regional Anatomy of the Cervical Spine
Osseous Components
The bony cervical spine is composed of seven vertebrae (Figure 24-1A-C). The lower five segments (C3-C7) are similar in morphology, while the first two segments (C1 and C2) are anatomically distinct. The first cervical segment, the atlas (C1), is ring-shaped and articulates primarily with the occipital condyles above and the superior facets of the second cervical segment below. The second cervical segment, the axis (C2), has a cone-shaped projection (the odontoid process) that articulates with the anterior arch of C1. The remaining cervical vertebrae (C3-C7) share similar architecture; the vertebral bodies are roughly cylindrical in shape and increase in size from rostral to caudal. From each body projects an uncinate process superiorly, which indents the posterolateral margins of its respective intervertebral disc. The transverse processes project anterolaterally and house the foramen through which the vertebral arteries pass. The spinal cord runs through the spinal canal, which is formed by the posterior elements including the pedicles, the facet joints, the lamina, and the spinous process. At each level, nerve roots exit the canal between vertically adjacent pedicles (Figure 24-1C,D)
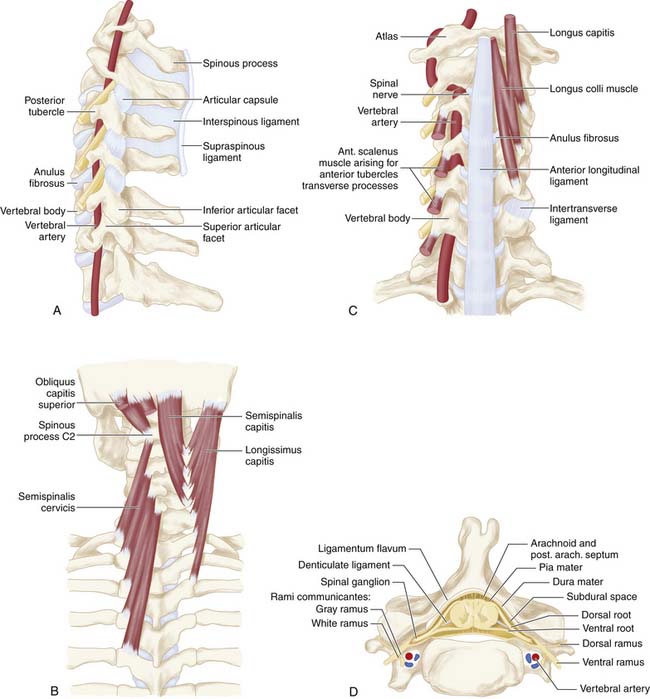
FIGURE 24-1 A, A lateral view of the cervical spine demonstrates the relationship of the cervical facets with the angled superior and inferior articulating facets and their encasing articular capsules. The anterior intervertebral discs and bodies are also seen. The spinous processes and dorsal arches are significantly stabilized by the dorsal interspinous and supraspinous ligaments. B, A posterior view of the cervical spine demonstrates the complex arrangement of the dorsal stabilizing musculoligamentous complex and its attendant musculature. C, An anterior view of the cervical spine demonstrates the anterior longitudinal ligament connecting the vertebral bodies at the level of the discs as well as the intertransverse ligaments between the transverse processes. The relationship of the vertebral arteries coursing rostrocaudally through the foramina transversaria is also shown. D, An axial view of a cervical segment shows the spinal canal containing the neural elements which are stabilized and held in place within the subdural space by the dentate ligaments. The nerve roots with their dorsal root, ventral roots, and spinal ganglia are shown as well. The dorsal arch of the ligamentum flavum lies directly below the neural elements. Again shown are the vertebral arteries within the foramina transversaria with their relationship to the nerve root and the uncinate joint.
The unique morphology of C1 and C2, as well as the increasing vertebral body size of the lower segments, may contribute to the pathogenesis of spondylosis. The anteroposterior diameter of the canal is generally larger at C1 and C2 when compared to the lower vertebrae; thus the spinal cord is estimated to occupy only one third of the atlantal ring at C1, while it occupies up to three fourths of the canal in the lower segments. This variability may account for the predisposition for spinal stenosis with symptomatology at the C4-C7 levels.7
Intervertebral Discs
The vertebrae are linked together by intervertebral discs, which provide a stable yet flexible architecture. The discs normally account for 22% of the height of the spinal column and function to spread axial loading forces. Each disc is composed of four elements: the nucleus pulposus, the anulus fibrosus, and the two cartilaginous endplates. The nucleus pulposus accounts for 40% of the cross-sectional area of the intervertebral disc and is located toward the posterior aspect of the disc. It consists of primarily of Type II collagen fibers and mucopolysaccharide ground substance. The anulus fibrosus is made of fibrocartilage that surrounds the nucleus pulposus in a concentric lamellar fashion. It is attached directly to the vertebral bodies via Sharpey fibers, which project from the outer lamella to the epiphyses. The anulus also attaches to the anterior and posterior longitudinal ligaments, but the posterior attachment weakens with age, which may lead to a predisposition toward central posterior disc protrusions. The endplates are cartilaginous structures situated between the nucleus pulposus and the trabecular bone of the vertebral bodies. Perforating fibers anchor the endplates to the nucleus and serve as a conduit for nutrients to enter the disc.
Ligaments and Joints
The cervical spine allows for greater mobility than the thoracic or lumbar regions. Various ligaments reinforce the cervical spine during flexion, extension, and rotational movement. The anterior and posterior longitudinal ligaments extend the entire length of the spine and provide stability to the intervertebral joints. The anterior longitudinal ligament attaches to the ventral aspect of the vertebral column and is apposed to the intervertebral discs, while the posterior longitudinal ligament courses along the dorsal aspect of the vertebral column and merges fibers with the annulus as well as the adjacent endplates (see Figure 24-1C). The ligamentum flavum attaches to the anterior surface of each vertebral arch and to the superior edge of each lamina, and covers each facet joint. Its function is to stabilize the neck during flexion (see Figure 24-1D). Due to its considerable elastic capacity, the ligamentum flavum can normally stretch during flexion without compromising the integrity of the spinal canal. Additional ligaments such as the supraspinous and interspinous ligaments serve see further stabilize the spinal column (see Figure 24-1B).
The superior and inferior facets comprise the articular pillars (see Figure 24-1A). In the cervix, these joints are angled obliquely and inferiorly and are oriented perpendicular to the vertebral bodies. Each superior facet articulates anteriorly to its inferior process, forming synovial joints, each with a fibrous capsule.
Vascular Supply
The cervical cord’s vascularity is provided primarily by the paired vertebral arteries, which arise from the subclavian arteries in the thorax and course superiorly to form the basilar artery at the base of the pons. Branches from the vertebral arteries form the anterior spinal artery, which courses along the ventral median fissure of the spinal cord, and is responsible for supplying the anterior two thirds of the cord. The vertebral arteries also give rise to the paired, posterior spinal arteries, which travel along the dorsal aspect of the cord (see Figure 24-1D).8 Cervical medullary arteries branching from the vertebral arteries create variable anastomoses within the canal and contribute additional vascular supplies.
Pathophysiology of Cervical Spondylosis
Cervical spondylosis is a multifaceted degenerative process that can affect all components of the spine including the intervertebral discs, facet joints, ligaments, spinal soft tissues, and bony elements. The initial pathological changes in spondylosis originate in the disc space. The proposed mechanism of disc degeneration occurs secondary to an alteration in the protein composition of the disc matrix. With aging, the molecular weight of the glycoproteins in the disc decreases along with the chondroitin sulfate content. The net effect is a change in the osmotic properties of the disc, with decreased inflow of fluid. Dehydration of the disc leads to loss of height, as well as loss of expansion capability under axial loading. As the disc progressively loses the ability to distribute normal loads of pressure, the nucleus pulposus becomes predisposed to fragmentation. Fragmentation of the nucleus combined with increasing weakness in the annulus with aging can result in herniation of disc material into the spinal canal (Figure 24-2A).
Loss of disc height not only contributes to disc herniation, but also results in osteophyte formation. As the annulus bulges under the impaired function of the damaged disc, the periosteum of the adjacent vertebral bodies undergoes reactive processes. Hyperostosis of the subperiosteal bone generates a spondylotic ridge or osteophyte, which can impinge on the ventral canal to cause cord compression. Spondylosis also leads to hyperostosis of the posterior elements. In the dorsolateral spinal column, decreased disc height causes pathological changes in the facets with destruction of the joints and resultant hypertrophy. Abnormal mobility of the spine contributes to osteophyte formation in the neural foramina, leading to peripheral nerve compression and radiculopathy. As spondylosis progresses, the ligamentum flavum becomes hypertrophic and loses elasticity. During hyperextension especially, the ligament tends to buckle inward, contributing additionally to canal compromise.10
The mechanism by which spondylosis leads to cord injury is not entirely understood; the pathophysiology is complicated by the common absence of symptoms in patients with radiographic evidence of significant disease.11 Spondylosis with disc herniation, osteophyte formation, and ligament hypertrophy clearly reduces the anterior-posterior diameter of the spinal canal. As expected, patients with congenitally narrow canals are therefore more prone to be symptomatic. Studies have suggested that the normal canal diameter is 17 to 18 mm (C3 -C7) and that a reduction in axial diameter to 11 to 13 mm is more likely to lead to myelopathy.10 Abnormal cervical motion and instability following degenerative changes may exacerbate cord injury. With neck flexion, the cord may move against the ventral spondylotic ridges causing cord damage. Extension may lead to cord strangulation between the folded ligamentum flavum posteriorly and osteophytes or herniated disc material anteriorly (Figure 24-2B).
There is considerable debate as to whether cord injury is due to direct compression of neural structures or secondary to extrinsic compromise of vascular supply. The vascular ischemia theory was first proposed in 1954 by Brain.2 Breig noted later that in cervical flexion, mechanical flattening of the spinal cord occurred, with consequent decreased patency of the anterior sulcal and transverse arteries.12 Other authors have noted that anterior-posterior compression of the spinal cord in both pathologic and experimental studies resulted in stretching of the transverse vessels and terminations of the anterior spinal artery with ischemia of the anterior two thirds of the cord.8,13,14,15 Clinically, Allen noted that the cervical spinal cord blanched during flexion in patients with spondylosis.16
Human pathologic studies of cervical spondylosis demonstrate that canal compromise with cord compression results in characteristic histological changes. Ono et al. found that compression of the cord is associated with extensive destruction of both gray and white matter, with consequent demyelinization.17 Interestingly, the areas of the cord most encroached upon tend to display histopathological evidence of severe infarction. Ogino et al. demonstrated an association between localized infarction of the gray matter and a decrease in the anterior-posterior canal ratio to below 20%.18 As cord injury secondary to spondylosis progresses, tissue destruction leads to gliosis, scarring, cystic degeneration, and neuronal cell loss.
Clinical Presentation of Cervical Spondylosis
Myelopathy is a common and severe manifestation of cervical spondylosis.5 Symptoms may be slowly progressive and associated with intermittent periods of remission and exacerbation.20 Clinical presentation generally consists of lower motor neuron involvement at the level of the lesion, with upper motor neuron signs at segments below. Upper extremity involvement is often unilateral, while that of the lower extremity is bilateral. Lower motor neuron findings include weakness and atrophy with progressive loss of dexterity, particularly at the level of the lesion. The lower extremities may demonstrate spasticity, clonus, hyperreflexia, or abnormal gait, with a positive Babinski sign. Sensory disturbances are poorly localized, generally affecting the lower extremities and trunk, while rarely involving cervical levels.20 Bowel and bladder impairment are rare, but indicate poor prognosis.
Due to the complex symptomatology of cervical degenerative disease, Crandall et al. described five clinical syndromes to aid in clustering various findings.21
Among other possible findings is the “numb, clumsy hand.”23 This condition involves a glove-like distribution of primary sensory loss combined with motor loss. Tandem spinal stenosis simultaneously affects the cervical and lumbar regions, presenting with a trio of symptoms: neurogenic claudication, gait abnormality, and mixed upper and lower motor neuron signs.24 Vertebral artery insufficiency can present with dizziness and unsteadiness when the head is rotated.25 Rarely, large osteophytes can cause dysphagia due to direct compression of the esophagus.5
It is important to consider other neurological conditions that may exhibit symptoms mimicking cervical spondylosis. Any mass lesion within the spinal canal that compresses the cord or nerve roots can manifest with such findings. Extradural, intradural, and osseous tumors of the spine, as well as infectious processes such as epidural abscesses, can compromise canal integrity. Fortunately, these conditions can generally be distinguished from cervical spondylosis via effective MRI. Multiple sclerosis is another condition commonly confused with cervical spondylosis, and a mixed picture of upper and lower motor neuron signs is a hallmark finding in amyotrophic lateral sclerosis. Correctly diagnosing cervical spondylosis depends on detailed history-taking, complete neurological examination, and diagnostic measures including imaging, neurophysiology, and laboratory tests.
Diagnostic Modalities
Neuroradiology
Plain film radiographs of the cervical spine are traditionally performed with a series of anteroposterior, lateral, and oblique films. Relevant findings on lateral films include the height of disc spaces and evidence of osteophytes protruding into the spinal canal. Also of import is the anteroposterior diameter of the canal, as it is highly indicative of disease severity in patients with symptomatic spondylosis.22 The diameter is determined to be the shortest distance from the dorsal aspect of the vertebral body (including any posteriorly projecting discs or spurs) to the spino laminar line, with 12 mm in the lower cervical region being the lowest normal value.26 This calculation, however, is manipulated by the magnification of the film — an obstacle circumvented by an alternative method described as Pavlov’s ratio.27 This number represents the ratio of the anteroposterior diameter of the spinal canal divided by the anteroposterior diameter of the corresponding vertebral body. A normal value is approximately 1, with values of 0.8 or less suggesting compression. This method allows quick appraisal of the integrity of the canal without being influenced by magnification.
Computed tomography allows for better assessment of the spinal canal than plain radiography.26 CT axial plane images have been shown to provide an accurate estimate of the canal diameter while also differentiating laterally projecting osteophytes and midline calcifications (i.e., as seen in OPLL).28 CT scans alone, however, poorly visualize the soft tissue structures within the spinal canal. With the addition of intrathecal contrast, CT myelography can allow for quantification of cord compression at every level. CT myelography has been effective in correlating symptomatic disease with cross-sectional area of the canal.29
Magnetic resonance imaging is the most recent advancement in the radiographic evaluation of cervical spondylosis, offering the advantages of imaging in multiple planes and improved definition of neural and ligamentous elements. Disc herniations are readily demonstrated and often have associated signal changes (see Figure 24-2C). MRI also distinguishes cervical spondylosis from disease processes that mimic it clinically, such as tumors, epidural masses, demyelination, and syrinx. The complete neuraxis can also be easily imaged if necessary. In comparison to CT myelography, MRI is a safer, less invasive procedure, making MRI the procedure of choice for initial evaluation of radiculopathy or myelopathy.28,30,31
Unlike conventional x-ray technology, MRI allows for the demonstration of pathological processes within the spinal cord parenchyma. Intramedullary signal intensity changes have been noted at segments adjacent to areas of spondylotic compression32 (see Figure 24-2D). In experimental models, histological confirmation of cord injury is found at levels demonstrating MRI signal change with maximum mechanical compression.33 The cause of signal change is attributed to myelomalacia, gliosis, and edema.32,34,35 Clinical data correlating the degree of signal change with outcome are confusing at best, but high-intensity lesions are thought to suggest poor prognosis.34,36,37,38
MRI still poses problems in diagnosing certain degenerative changes. Small, lateral osteophytes can be difficult to distinguish from lateral disc herniations.28 Also, midline calcifications seen in ossification of the posterior longitudinal ligament (OPLL) may be poorly visualized. In addition to these limitations, the high incidence of degenerative abnormalities imaged in asymptomatic individuals also proves problematic. Teresi et al. found disc protrusions in 57% and spinal cord impingement in 26% of patients over 65 years of age when clinical evidence of cervical spondylosis was absent.39 So although imaging techniques allow direct visualization of disease progression, determination of patient prognosis and indication for surgical intervention is not made by such modalities alone.
Neurophysiology
Neurophysiological evaluation of cervical spondylosis may prove a valuable supplement to other findings. Recent interest in these functional diagnostic modalities stems from the difficulty in interpreting common radiographic abnormalities in asymptomatic patients. Neurophysiological testing may also assist in predicting prognosis and measuring response to treatment. In evaluating cervical spondylosis, electromyography (EMG) allows differentiation of radiculopathy from neuropathy, and peripheral from central nerve entrapment.40 EMG also helps localize affected nerve roots by demonstrating conduction abnormalities in muscles innervated by adjacent cervical segments. This technique may assist in preoperative determination of levels requiring decompression. Somatosensory evoked potentials (SSEPs) involve electrical stimulation of peripheral sensory nerves while recording evoked activity from either the spinal cord or sensory cortex. Clinical studies showing spondylotic involvement of the posterior columns suggest that SSEPs may help appraise the functional status of the sensory system. Leblhuber et al. found that dermatomal SSEPs were altered at levels corresponding to cervical segments with degenerative changes.44 Yet these neurophysiologic and radiographic abnormalities were also found in asymptomatic patients. Although experimental models have found a temporal relationship between changes in SSEPs and the onset of neurological deficit,33 the diagnostic value of SSEPs has been challenged through studies that found median and ulnar nerve abnormalities in only a small percentage of patients with symptomatic cervical spondylotic myelopathy.42,43
Cortical motor evoked potential (MEP) recording has been suggested as a more sensitive test of spinal cord dysfunction than SSEPs,44 due to the predominance of motor findings in patients with cervical spondylosis.44 MEP abnormalities may also be detected in patients before the onset of clinical symptoms.43–45 In comparison, MEP can detect abnormalities in 84% of patients with radiographic cord compression, while SSEPs show dysfunction in only 25%.46
The role of electrophysiological studies in diagnosing cervical spondylosis or predicting outcome is not entirely clear at this time. Cusick suggests that combining MEP and SSEP recordings allows for evaluation of long tract function of both ascending and descending white matter. These two tests provide insight into the integrity of two spinal cord areas often affected by spondylosis. By integrating electrophysiological studies and radiographs, patient vulnerability to neurological deficit may be estimated, as well as optimal timing of surgical intervention for patients with subclinical disease.44
Natural History of Cervical Radiculopathy
Lees and Aldren-Turner classified cervical spondylosis as a relatively benign condition.19 The common course experienced by their patients was characterized by long periods of stable symptomatology interspersed with short bouts of deterioration; chronic, gradual deterioration was rare. They also found that myelopathy did not develop in a group of patients presenting solely with radiculopathy. In following studies, Nurick agreed with the findings of Lees and Aldren-Turner, and also found that patients who had undergone surgical laminectomy had no significant improvement over those with no treatment.3 Thirdly, he realized that age of onset served to significantly determine the prognosis for later deterioration.
Scoville found that the best outcome of surgery was in patients treated within one year of onset of symptoms.48 He further elaborated on Lees and Aldren-Turner’s model in claiming that patients should be treated surgically, shortly after mild disability was noticed, and before further progression. He did admit that mild cases were adequately treated conservatively.
Smith and Robinson more recently described the course of cervical spondylosis that is most accepted today.47 They found that motor complaints tended to be more permanent than neck, bladder, and sensory symptoms. Motor findings were also predominant in the lower extremities, and sensory in the upper. Although most of their patients followed an episodic but unpredictable pathway, one third of cases were found to be nonprogressive between acute episodes, while two thirds experienced a gradual increase in symptoms between intermittent, acute episodes of worsening. A minority of patients had a constant worsening in condition, and very few people enjoyed spontaneous improvement. Their conclusion was that although progression of the disease is usually slow, prognosis is poor, and improvement rare. They hypothesized that patients reporting improvement may simply be coping better, or may simply be reporting a slowing of progression.
Posterior Cervical Surgical Techniques
Laminoforaminotomy and laminectomy should be performed under general anesthesia, with the neck in a neutral position to prevent stretching of the spinal cord over any anterior protrusions within the canal. The patient can either sit or lie prone; the former allows a dry field, but increases the risk of an air embolism. After proper fixation of the head in pins and/or traction as noted above, radiographic assessment of the spinal alignment and confirmation of the neurological exam should be obtained prior to incision (Figure 24-3A). A midline incision is then made over the pathology in question. For occipitocervical fusions, this incision should typically extend from the inion on the skull to slightly above the C7 prominence. A more limited incision can be made for subaxial cases. Due to the narrowed interspaces and inferior inclination of the spinous processes, overdissection and stripping of the facets may lead to unwarranted fusion of uninvolved levels, leading to an excessive decrease in mobility.
The dissection is carried down sharply in the midline to the level of the posterior cervical musculature fascia. A midline raphe is formed from the union of the deep cervical fascia, prevertebral fascia, ligamentum nuchae, and the supraspinous ligaments (Figure 24-3B). By maintaining the exposure in this relatively avascular midline plane, blood loss can be minimized. Frequent palpation of the bony processes demarcating the midline is essential. Self-retaining retractors are placed to maintain the exposure, but excessive retraction can obscure the midline and lead to scything to either side.
After the ligamentum nuchae is encountered over the cervical spinous processes, a subperiosteal dissection is performed to mobilize the muscles off the processes. As the processes are often bifid, care should be taken to avoid accidental spinal canal entry. The exposure is then carried down to the lamina which are palpated and identified. Using gentle lateral retraction with a small Cobb elevator, electrocautery is used to dissect the muscles off the lamina (Figure 24-3C). Excessive downward pressure with the elevator must be avoided, as the cervical spine is highly mobile even in its normal state under anesthesia. The laminae of the cervical spine are angulated 45 degrees from medial to lateral and also in a cephalic direction. The interlaminar areas are also wide and should be exposed cautiously. It is here that the venous plexus overlying the vertebral artery is often encountered as the facet capsule is exposed. Bipolar cautery and gentle tamponade with Gelfoam are usually effective in obtaining hemostasis. For uninvolved levels, care should be taken to preserve the facet capsule (zygapophyseal joint). Self-retaining retractors to maintain exposure should be placed at or above the anteroposterior plane of the facets to avoid injury to the nerve roots and vertebral artery.
Alternatively, a minimally invasive paramedian tubular approach can be used as well. A stab incision is initially made approximately 1 cm off midline ipsilateral to and at the level of the pathology. Under fluoroscopic guidance, a small tubular dilator or pin is inserted through the posterior cervical musculature and fascia down to the facet or lateral mass of the target level. Although we have not routinely done so, anteroposterior radiographic images could be obtained to guarantee proper pin positioning (Figure 24-4A). Once the dilator has been docked on the facet in question, the skin incision is extended above and below the Steinmann pin for a total length of approximately 2.0 cm. The skin edges are retracted and the cervical fascia is incised using Metzenbaum scissors. Care should be taken not to cut muscle fibers during this procedure, as this can cause unnecessary blood loss. This sharp opening of the fascia allows for easier passage of the sequential dilating cannulas with a minimum of force (Figure 24-4B). A series of dilators is then sequentially inserted through the neck soft tissues, over which an 18mm tubular retractor is then inserted (Figure 24-4C). Real-time lateral radiographic images are obtained as often as needed to insure a proper working trajectory throughout this process (Figure 24-4A-C). The working channel (tubular retractor) is then attached to a flexible retractor affixed to the operating table side rail, and locked in position at the junction of the lamina and lateral mass (Figure 24-4D).
Once the standard or minimally invasive retractor is set in the desired position over the correct level as confirmed by fluoroscopic imaging, a Bovie cautery with a long tip is then used to remove the remaining muscle and soft tissue overlying the lateral mass and facet. Typically loupe magnification or an operating microscope is used for maximal visualization. With the bone well delineated, a small straight cervical curette is used to scrape the inferior edge of the superior lamina and the medial edge of the lateral mass/facet. This exposure is then carried underneath the lamina and facet with the use of a small angled curette. Proper placement of the curettes can be confirmed under fluoroscopy. Good dissection of the underlying flavum and dura from the bone defines the relevant anatomy and helps to prevent incidental dural tears. Bleeding from epidural veins and the edge of the flavum is controlled via long-tipped bipolar cautery. A small angled Kerrison rongeur is then utilized to begin the foraminotomy. Periosteal and bone bleeding is addressed with bone wax and cautery. In cases of marked facet arthropathy and enlargement, a drill with a matchstick-type bit is used to further thin the medial facet and lateral mass (Figure 24-5A,B). Dissection with an angled curette facilitates safe use of the Kerrison rongeur. In this fashion, the decompression is continued.
The laminoforaminotomy is completed when the nerve root has been well exposed along its proximal foraminal course. The adequacy of the decompression should be confirmed by palpating the root along its course with a small nerve hook (Figure 24-5C). In cases in which a herniated disc was present, either a nerve hook or small #4 Penfield elevator is used to mobilize the nerve root superiorly to expose the disc space and fragment. For this maneuver, additional exposure is obtained by drilling a small portion of the superomedial portion of the pedicle directly below the exiting nerve root. With the root retracted, the disc fragment is then removed in a standard fashion with curettes and long endoscopic pituitary rongeurs (Figure 24-5D). Additional osteophytes encountered in this region can also be drilled or curetted as needed. Upon completion of the discectomy and decompression, the nerve hook is again passed along the exiting root to confirm its free passage and a lateral fluoroscopic image is obtained.
Anterior Cervical Surgical Techniques
Another surgical option is the anterior approach, which allows for fusion of the vertebral column concurrent with removal of osteophytes and prolapsed discs protruding into the anterior space of the canal. This approach is preferred in cases involving compression at one or two levels (although it is rarely performed in cases with three or four), and is particularly useful for patients with cervical instability. Several variations upon this method have arisen since its inception, with none having a distinct advantage.
As with laminectomy, this procedure is performed under general anesthesia, with the neck in a neutral position. The patient is placed in supine position with a roll placed transversely between the scapulae in order to extend the neck (Figure 24-6A). Following palpation of landmarks, a 2 to 3 cm skin incision is made along a skin crease at the level of interest between the sternocleidomastoid (SCM) and slightly off the midline (M) (Figure 24-6B). Finally, ensuring the correct level of the skin incision also prevents difficulty in dissection. Palpable landmarks in the neck aid in identifying the approximate level. The inferior angle of the mandible corresponds to C2-3; the hyoid bone to C3; thyroid cartilage to C4-5; the cricoid cartilage to C6, and the carotid tubercle to C7. Alternatively, a longer oblique or longitudinal incision can be made if an extensive decompression is planned (i.e., greater than 3-level corpectomy). Injection of lidocaine with epinephrine into the skin prior to incising may help diminish superficial bleeding.
Incise the superficial fascia overlying the platysma along the skin incision. The subsequent surgical course is through a potential space through the trajectory demonstrated in Figure 24-6C. The fibers of the platysma muscle are then either incised longitudinally along the direction of its fibers or split transversely. The deep cervical fascia underneath is then identified. Next, palpate the medial border of the sternocleidomastoid muscle and carefully split the fascia longitudinally. This allows one to retract the SCM laterally. The laryngeal strap muscles (sternohyoid, sternothyroid) as well as the midline structures immediately deep to them (trachea [T] and esophagus [E]) are then retracted medially. Deep to the SCM muscle, identify the carotid sheath (C) as well as the pretracheal fascia overlying it. Carefully incise the fascia medial to the sheath while protecting the midline structures. The carotid sheath can now also be retracted laterally.
Using blunt dissection, develop a plane toward the midline until the prevertebral fascia directly anterior to the vertebral bodies can be visualized. Identify the midline of the vertebral bodies (corresponding to the white stripe of the anterior longitudinal ligament) as well as the longus colli on each side. Using electrocautery, incise the prevertebral fascia longitudinally to the desired length. Use a periosteal elevator to then subperiosteally uncover the vertebral bodies and intervening disc spaces (A). Place retractors under each longus colli muscle to protect surrounding structures. The view of the field is provided by an operative microscope, which minimizes risk of injury during cord decompression. Place a spinal needle in a disc space and obtain a cross-table lateral film of the cervical spine to confirm the level. An assistant pulling axially on wrist straps depresses the shoulders and allows for a better radiograph. After confirming the level, a discectomy and/or corpectomy can be performed as indicated. Distraction pins can be placed in the appropriate vertebral bodies, and general distraction applied to facilitate discectomy and decompression, particularly in heavily collapsed spaces (Figure 24-7A).
The prolapsed disc and osteophytes are removed, and a bone graft is inserted within the interspace to provide stability and promote fusion. Typically, straight and angled curettes are used to free the disc and cartilaginous portions of the endplate from the bony vertebral body surfaces. Fragments of disc are then removed with pituitary rongeurs (Figure 24-7B). This process is gradually advanced down toward the level of the posterior longitudinal ligament (PLL). At times, drilling with a matchstick-type high-speed drill bit is useful to flatten irregular endplate osteophytes as well as to remove anterior closing lip osteophytes. Particular attention, however, should be taken to avoid excessive damage to the bony endplates, especially if grafting will be done, to prevent excessive subsidence of the graft. The PLL is then opened sharply with a small 1- or 2- mm Kerrison rongeur. Initial opening can be facilitated with the use of a small nerve hook probe. The PLL is then sectioned to reveal the underlying dura as needed, depending on the exact extent and location of the neurological compression as determined by the preoperative imaging and the patient’s clinical symptoms. It is particularly important to extend the decompression rostrocaudally by undercutting the marginal endplate osteophytes in an hourglass shape to ensure that there is no residual canal or foraminal compression (Figure 24-8A). The majority of surgeons, therefore, choose to remove larger osteophytes from posterior and posterolateral canal surfaces. Some surgeons also choose to open and excise the posterior longitudinal ligament. Doing so allows prolapsed discs to be more easily identified for removal (which is useful in up to 35% of cases). Further, excision of the ligament provides prophylaxis against buckling, a possible source of postoperative pain.
Autologous bone grafts are usually taken from the iliac crest (over 70% of the time), with other options including the patient’s tibia or fibula, bone banks, and calf bone (Figure 24-8B). Artificial grafts are also possible using methylmethacrylate, hydroxyapatite, or biopolymer. Although such materials do not necessarily provide a better outcome, donor site pain can be eliminated. Benefits of fusion include reduced risk of repeated spinal cord injury and pain by minimizing abnormal movement, as well as prevention and treatment of residual or recurrent nerve root compression via loss of disc space height. Another advantage of fusion is increased resorption of osteophytes, although complete resorption may take several years. A variety of specific interbody grafting techniques including the Smith-Robinson impacted type, the Cloward dowel, and the Bailey-Badgely slot type grafts have been commonly employed over the years (Figure 24-8C).
Vertebrectomy with strut grafting is a more recent surgical tool used in treating patients suffering from severe disease at many levels. Significant improvement is reported in 70% to 80% of cases, and many authors feel that this procedure is superior to other surgical options. When performing vertebrectomy, a trench is drilled out along the axes of the affected vertebra and disc spaces to achieve decompression, and the column is reconstructed using a graft from the iliac crest, rib, or fibula. In cases requiring immediate stabilization, titanium or PEEK implants can be used to reinforce the vertebral column, while also providing magnetic resonance imaging compatibility for postoperative imaging.
Anterior cervical microforaminotomy is a well-established minimally-invasive anterior cervical procedure and has the advantage of good decompression of the nerve root and maintenance of spinal stability without the need for fusion.. Technically more demanding, decompression of the nerve root is achieved by removing the osteophytes from a lateral to medial trajectory with a focus on preserving the structural stability of the remaining joint and intervertebral disc (Figure 24-9A,B). Small slim retractors are docked at the junction of the lateral uncinate joint and the transverse process, immediately medial to the vertebral artery at the level of the disc and exiting nerve root (Figure 24-9C). With the vertebral artery retracted laterally, curettes and drill bits are used to expand the foramen and decompress the lateral aspect of the uncinate joint through the dorsolateral osteophyte (Figure 24-9D). Once exposed, the disc herniation is resected with micropituitary forceps (Figure 24-9E) to thereby ultimately decompress the neural foramen and exiting root (Figure 24-9F).
Surgical Outcomes
Assessing the results of surgery for cervical spondylosis has proved difficult for many reasons. As the disease is heterogeneous in presentation, studies include a variety of cases disparate in age, extent of vertebral involvement, and severity of symptoms. To date, no prospective, randomized experiments have been published. Currently available publications consist mostly of retrospective reviews of different procedures performed at the same institution. Furthermore, there is no standardized method of assessment. The Japanese Orthopedic Association proposed a scale involving four-limb function and bladder symptoms. The Odum scale grades from excellent to poor, and the Nurick system assesses ability to walk. Magnetic resonance has been used to determine the underlying cause of poor postoperative results. In examining 56 patients, Clifton found that only 12 patients had adequate decompression at the correct levels, whereas 32 cases had either residual compression at operated levels or untreated compression at additional levels.55 Batzdorf and Flannigan similarly found patients with residual cord compression.56Harada found that when significant decompression was not achieved, recovery was significantly worse.57 In light of these findings, the necessity of preoperative imaging for accurate determination of involved levels and best method of approach becomes apparent.
The effectiveness of posterior cervical laminoforaminotomy for decompression of the lateral recess and neural foramen has been well documented in numerous publications over the last four decades.48,49 When compared to standard anterior cervical techniques, the posterior approach via a “keyhole” type of osteotomy may provide better exposure for decompression of the exiting root and for removal of lateral osteophytes and discs. Previous work examining laminoforaminotomy has shown that adequate foraminal exposure can be accomplished without necessarily destroying the facet joint or causing iatrogenic instability.50 As long as less than 50% of the facets are removed, there is little compromise of the sheer biomechanical strength of the cervical spine.51 The posterior approach also avoids the additional risks of injury to the anterior structures of the neck including the trachea, esophagus, thyroid, thymus, carotid arteries, jugular veins, vagus nerve, recurrent laryngeal nerve, superior laryngeal nerve, ansa cervicalis, and thoracic duct. Lastly, cervical laminoforaminotomy is an operation that treats the offending pathology without necessitating a fusion. As longitudinal studies now demonstrate an increased incidence of adjacent level problems following cervical fusion, avoiding arthrodesis when possible seems particularly prudent.52 Overall, no statistically-significant difference in results between anterior and posterior approaches for the management of isolated cervical radiculopathy has been demonstrated.53 The overall clinical popularity of laminoforaminotomy was tempered by technical limitations including a limited surgical view, difficulty in resecting osteophytes, limited visualization of the distal foramen, and often generous epidural venous plexi and associated bleeding.54 Furthermore, the muscle dissection often needed to obtain an adequate surgical exposure has been associated with increased postoperative muscle spasm, neck pain, and recovery time. When considering laminectomy, reported results tend to disagree. With respect to age, some investigators find a significantly better outcome in patients under 50, while others do not. Regarding general overall improvement, some studies reported benefit in over 80% of cases, whereas others found no significant advantage over conservative therapy. Finally, most authors agree that shorter duration of preoperative symptoms is a good prognostic factor, while some deny any significance. Possible confounding factors include the consideration of cases where osteophyte removal, opening of the dura, or sectioning of the dentate ligaments was performed concurrently with laminectomy (which tends to cause more complications due to neural injury). Despite the glaring differences, it is generally believed that younger patients who have less neurological involvement and a shorter duration of disease enjoy greater improvement.
Overall, clinical outcomes associated with the surgical treatment of degenerative disc disease by anterior cervical discectomy (ACD) or anterior cervical discectomy and fusion (ACDF) have been excellent. According to a literature review from 1991,58 good clinical outcomes have been reported for 61% to 94% of ACDF cases and for 65% to 96% of ACD cases. These data showed that an interspace fusion is not mandatory for good clinical outcomes. The development of a fibrous union or pseudarthrosis has not been consistently associated with poor clinical outcomes. However, once pseudarthrosis is present, 67% of patients have associated symptoms.59 Since then, the question of whether an interbody fusion is required has been unresolved.60 Proponents of ACD favor its simplicity, low cost, and the absence of complications related to autograft harvest and interbody graft failure (e.g., graft extrusion, collapse, subsidence, and pseudarthrosis). Advocates of ACDF stress that foraminal decompression by interbody distraction, prevention of disc space collapse, and stabilization of cervical alignment are key advantages compared with ACD alone. The resorption of dorsal osteophytes has been attributed to fusion and immobilization of the segment. The postoperative incidence of neck pain has been reported to be smaller with fusion than without. Furthermore, the incidence of kyphotic deformity is thought to be higher if fusion is omitted. Comparative, prospective clinical studies between ACD and ACDF, however, failed to find a clinical benefit to ACDF.61–68
Despite these findings, the overall trend in the United States and Canada has been toward increased application of anterior interbody fusion for the treatment of cervical degenerative disc disease.69–71 Autograft from the iliac crest has usually been used for interbody fusion. However, its harvesting is associated with complications such as prolonged pain, cosmetic deformity, wound infection, hematomas, and peripheral nerve irritation or injury.
Unplated anterior cervical interbody fusion for degenerative disc disease has a higher tendency to fuse with autograft than allograft, sometimes with better clinical outcomes.72–74 In contrast, other studies failed to demonstrate significant differences in radiological or clinical outcomes between allograft and autograft.75–77 A meta-analysis of the literature comparing fusion outcomes of allograft and autograft for one- and two-level cervical interbody fusion without plating found a higher fusion rate for autograft and a lower incidence of graft collapse than for allograft. However, clinical outcomes were statistically similar.78 A review of the literature failed to find allograft to be an adequate equivalent to autograft for anterior cervical interbody fusion.79 However, the morbidity associated with autograft harvest was eliminated by the application of allograft. The possibility of transmitting infectious diseases like human immunodeficiency virus from tissues, including allograft, donated by a screened donor is exceptionally rare.75,76
A prospective study of ACDF comparing autograft and biocompatible osteoconductive polymers found significantly less graft protrusion and intersegmental kyphosis in the biocompatible osteoconductive polymer group. However, this study failed to demonstrate incorporation or biodegradation of biocompatible osteoconductive polymers.80 In a prospective, nonrandomized study, Senter et al. 81 compared the outcomes of autograft ACDF with ACDF with hydroxyapatite. Fusion with the latter was equal or superior to that with autograft alone. In prospective comparisons of autograft and xenograft, clinical and radiological data favored the use of autograft.82,83
Recent prospective clinical trials of fusion with interbody titanium cages have found promising clinical results and radiological outcomes, with low rates of implant failure (e.g., backout and subsidence) or pseudarthrosis when compared with allograft or autograft. Even the fusion rates for cages are superior to those associated with autograft or allograft fusion.84–86 Despite these recent data, some conclude that autograft remains superior to alternative interbody fusion materials.87
In the early 1960s, Bohler88 applied an anterior cervical plate and screw construct to treat traumatic instability of the spine. After his report, anterior cervical plate constructs were applied using bicortical, nonlocked, variable-angle screws for fixation.89,90 However, hardware failure was common,91 and a unilateral locked, fixed-angle plate-screw anterior system was introduced by Morscher et al.92 in 1986 (Figure 24-10A).
A variety of unicortical locked, dynamic, fixed, or hybrid plate-screw systems are now available for anterior cervical interbody fusion and plating93 to increase stability of the cervical fusion segment (Figure 24-10B,C). As a result, fusion rates have increased, and the rates of graft failure and pseudarthrosis have thereby decreased.94,95 Furthermore, anterior cervical plate fixation for degenerative disc disease maintains sagittal balance more effectively,96–98 thereby potentially limiting adjacent level biomechanical stress.99 Postoperative loss of lordosis and cervical kyphosis have been associated with ACD and ACDF without plating. Yet, again, prospective randomized studies comparing single-level ACD, ACDF, and ACDF with plating have failed to show a clinical benefit associated with either procedure,66,100 although a clinical benefit was found for two-level procedures.101 Moreover, concern has been expressed about the cost and complication of cervical plating for the treatment of degenerative disc disease. Hardware failure has been a source of early and delayed morbidity. In a prospective clinical trial, patients undergoing ACDF with plating tended to have a more frequent incidence of dysphagia than patients without plating. Yet, in the same study, more multilevel procedures were performed in the plating group, which could also account for these findings.102
In more recent surgical series, refinements in the design of anterior cervical plating and more surgical experience have lowered the incidence of plate-related complications.103–109 When all aspects, including possible reoperation and time to return to work, are considered, overall costs decrease when anterior cervical plating is added to fusion.110 Plating also may increase fusion rates with allograft and thereby obviate the need for autograft.111The complications associated with autograft harvest would be decreased without compromising fusion rates. Again, experts are divided on the need for plating, in particular, for single-level disease.
Complications of Surgery
The complications of these procedures are similar to those of any other type of surgery, with a mortality rate of less than 1.5%. Cardiac disorders, thrombophlebitis (with possible pulmonary embolism), and infection top the list. Vessels exposed to injury are the vertebral arteries, the carotid arteries, and the jugular vein; extradural or superficial hematomas may also occur. Nerves at risk include the paravertebral sympathetic chains, the superficial and recurrent laryngeal nerves, along with the spinal cord and nerve roots. Soft tissue complications may involve surgical injury to the trachea, esophagus, thoracic duct, and cervical pleura (Figure 24-11A). Often, the recurrent laryngeal nerve is injured from a combination of the anterior cervical retractor blades compressing the nerve against the endotracheal tube itself, thereby resulting in injury to the mucosal portion (Figure 24-11B). Infection runs the gamut from easily treated to discitis (leading to severe, lingering pain) or meningitis (also a complication of dural injury).
Emerging Technologies: Artificial Disc Replacement
After cervical spinal fusion, increased motion has been documented at adjacent levels,112 and radiological data have shown degenerative disc disease to occur at adjacent cervical segments on long-term follow-up113 (Figure 24-12A). Whether the increased incidence of adjacent level degeneration (ALD) found in the cervical spine after fusion is caused by increased mechanical stresses on adjacent cervical segments or whether the observed degenerative cervical changes merely represent the natural history of degenerative disc disease of the cervical spine is unknown. Clinical studies estimate that the incidence of symptomatic degeneration above a fusion ranges from 2% to 3% per year above the expected natural history114 (Figure 24-12B). The rationale underlying the use of artifical discs is to maintain physiologic segmental cervical motion after ACD and decompression of the neural structures. By maintaining cervical segmental motion, adjacent level motion is decreased.115 Theoretically, this decrease should eliminate or reduce the incidence of ALD. Several artificial discs have been designed and applied clinically. Short-term follow-up data have shown equivalent clinical outcomes when cervical degenerative disc disease is treated with conventional fusion or an artificial cervical disc.116–118 Earlier designers of artificial cervical joints showed a more frequent incidence of hardware failure when compared with more recent clinical evaluations of cervical artificial joints. 116–118 Placement of these discs is technically very similar to that of standard anterior cervical interbody grafting techniques. Authors describe the need for wider interbody exposure, discectomy, and more meticulous endplate preparation. Specific implantation techniques vary according to the specific design of the artificial disc. Whether these devices will be associated with superior clinical outcomes compared with standard surgical treatment options for cervical degenerative disc disease will only be determined when sufficient long-term follow-up data have been gathered from ongoing clinical trials in the United States and Europe.
The BRYAN cervical disc (Medtronics; Minneapolis, MN) trial was published in 2009119 (Figure 24-13A). This was a multicenter study in which patients with single-level cervical DDD were randomized to receive either the BRYAN cervical disc (n=242) or anterior cervical discectomy and fusion (n=221).14 The main study hypothesis was that the outcomes from disc replacement would be at least equivalent to fusion. 465 patients were followed for two years. Initially, investigators and patients were blinded to the procedure. However, postoperatively, the investigational group was treated with a two-week course of nonsteroidal antiinflammatory drugs and was allowed to resume nonstrenuous activities as they pleased. Because of these postoperative differences, “further blinding was not practical or ethical.” At 24-month follow-up there was a 91.6% retention rate. At 24 months, both groups had improvements in the clinical outcomes. Overall success in the intervention group was 82.6% compared with 72.7% in the fusion group (p=0.005). Neck Disability Index (NDI) scores were 16.2 in the intervention group and 19.2 in the control group (p=0.025). NDI success (defined as greater than 15 point improvement in the NDI) was 86% in the intervention group versus 78.9% in the fusion group (p=0.001). Improvements in SF-36 scores were comparable, as were measures of neck and arm pain. Patients who received the BRYAN artificial disc returned to work about two weeks earlier than those who had fusion. Given that this was a non-inferiority trial, an as-treated analysis was the primary analysis (versus an intention-to-treat analysis). There were 12 patients in the study who were randomly assigned to receive the artificial disc but who received the control treatment because of anatomic or technique difficulties during the surgery. Another important limitation is that 117 patients were randomly assigned but declined participation once receiving the assigned treatment, many because of dissatisfaction with the assigned treatment.
The Prestige trial (Medtronics; Minneapolis, MN) was published in 2007120 (Figure 24-13B). This was a multicenter trial in which 541 patients with single-level cervical DDD were randomized to cervical disc arthroplasty with the Prestige disc (n=276) or cervical fusion (n=265). Participants were not blinded. Overall success at 24 months was actually higher in the intervention group than in the control group. The NDI scores and NDI success were not statistically significantly different. The rate of neurologic success was greater in the intervention group. SF-36 scores and neck pain were improved more in the intervention group. There were fewer secondary surgeries in the intervention group.
The ProDisc-C (Synthes Inc; Paoli, PA) trial was published in 2009 and is the study upon which FDA approval was based121 (Figure 24-13C). This was a multicenter study at 13 clinical sites. A total of 209 patients were randomized to receive either the ProDisc artificial disc replacement (n=103) or ACDF (n=106). All participants had single-level cervical disc disease that was unresponsive to conservative treatment. All participants were blinded until after the surgery. The main study hypothesis was that the outcomes from disc replacement would be at least equivalent to fusion. A secondary hypothesis was that disc replacement was superior to fusion. All of the patients were followed for 2 years. The primary outcomes of overall success and NDI success were comparable in the two groups. Overall success was 72.3% in the intervention group versus 68.3% in the control group. The results of the secondary outcomes were comparable. Fewer secondary surgeries were required in the intervention group. Few patients in the disc replacement group required narcotics at follow-up (10.1% vs. 18.5%; p = 0.073). At 24-month follow-up, safety and efficacy were comparable in those who had disc replacement compared with those who got fusion.
Conclusion
The clinical presentation of spondylosis can range from no symptoms to radiculopathy or severe myelopathy. Diagnostic imaging modalities include plain radiography and magnetic resonance imaging. Computed tomography with intrathecal contrast may play a role in evaluating certain disease processes poorly imaged by MRI. The role of neurophysiological testing with motor and somatosensory evoked potentials is not clearly defined, but may prove beneficial in measuring subclinical disease and predicting outcome following surgery. Conservative medical management is indicated for patients with minimal symptoms, with surgical intervention reserved for patients with significant disability and/or a progressive clinical course. Both anterior and posterior approaches with or without grafting and fusion have been described. Surgical outcome is variable and significant prognostic factors are not yet identified. The association between cervical degenerative disease and age suggests an increasing prevalence of cervical spondylosis as the elderly population expands. Understanding of cervical spondylosis is still limited and further research is necessary to better define criteria for patient selection for surgery, appropriate surgical procedures, prognostic factors, and outcome measures.
1. Lestini W.F., Wiesel S.W. The pathogenesis of cervical spondylosis. Clin. Orthop.. 1989;239:69-93.
2. Brain R. Cervical spondylosis. Ann. Int. Med.. 1954;41:439.
3. Nurick S. The pathogenesis of the spinal cord disorder associated with cervical spondylosis. Brain. 1972;95:87-100.
4. Hunt W.E. Cervical spondylosis: natural history and rare indications for surgical decompression. In: Congress of Neurological Surgeons: Clinical neurosurgery. Baltimore: Williams and Wilkins; 1980.
5. Simeone F.A., Rothman R.H. Cervical disc disease. In: Rothman R.H., Simeone F.A., editors. The spine. Philadelphia: W.B. Saunders, 1982.
6. LaRocca H. Cervical spondylotic myelopathy: natural history. Spine. 1988;13:854-855.
7. Parke W.W. Correlative anatomy of cervical spondylotic myelopathy. Spine. 1988;13:831-837.
8. Turnbull I.M. Micro vasculature of the human spinal cord. J. Neurosurg.. 1971;35(2):141-147.
9. Breig A. Adverse mechanical tension in the central nervous system, second ed. New York: John Wylie & Sons, 1978.
10. Bohlman H.H., Emery S.E. The pathophysiology of cervical spondylosis and myelopathy,. Spine. 1988;13:843-846.
11. Cusick J.F. Monitoring of cervical spondylotic myelopathy. Spine. 1988;13:877-880.
12. Breig A. Adverse mechanical tension in the central nervous system, second ed. New York: John Wylie & Sons, 1978.
13. Breig A., Turnbull I.M., Hassler O. Effects of mechanical stresses on the spinal cord in cervical spondylosis: a study of fresh cadaver material. J. Neurosurg.. 1966;25(1):45-56.
14. Gooding M.R. Pathogenesis of myelopathy in cervical spondylosis. Lancet. 1974;2(7980):1180-1181.
15. Gooding M.R., Wilson C.B., Hoff J.T. Experimental cervical myelopathy: effect of ischemia and compression of the canine cervical spinal cord. J. Neurosurg.. 1975;43(1):9-17.
16. Allen K.L. Neuropathies caused by bony spurs in the cervical spine with special reference to surgical treatment. J. Neurol. Neurosurg. Psychiatr.. 1952;15:20.
17. Ono K., Ota H., Tada K., Yamamoto T. Cervical myelopathy secondary to multiple spondylotic protrusions: a clinico-pathologic study. Spine. 1977;2:109-125.
18. Ogino H., Tada K., Okada K., Yonenobu K., Yamamoto Y., Ono K., Namiki H. Canal diameter, anteroposterior compression ratio, and spondylotic myelopathy of the cervical spine. Spine. 1983;8:1-15.
19. Lees F., Aldren-Turner J.W. Natural history and prognosis of cervical spondylosis. BMJ. 1963;92:1607-1610.
20. Epstein J.A., Epstein N.E. The surgical management of cervical spinal stenosis, spondylosis, and myeloradiculopathy by means of the posterior approach. In: The Cervical Spine Research Society. Philadelphia: J.B. Lippincott Company; 1989.
21. Crandall P.H., Batzdorf U. Cervical spondylotic myelopathy. J. Neurosurg.. 1966;25:57-66.
22. Friedenberg Z.B., Broder H.A., Edeiken J.E., Spencer H.N. Degenerative disc disease of cervical spine. JAMA. 1960;174:375-380.
23. Good D.C., Couch J.R., Wacaser L. “Numb, clumsy hand” and high cervical spondylosis. Surg. Neurol.. 1984;22:285-291.
24. Dagi T.F., Tarkington M.A., Leech J.J. Tandem lumbar and cervical spinal stenosis. J. Neurosurg.. 1987;66:842-849.
25. Giroux J. Vertebral artery compression by cervical osteophytes. Adv. Otorhinolaryngol.. 1982;28:111-117.
26. Alker G. Neuroradiology of cervical spondylotic myelopathy. Spine. 1988;13:850-853.
27. Torg JS, Pavlov H, Robie B, Jahre C: Pavlov’s ratio: A simplified, accurate and specific method for determining stenosis of the cervical spinal canal, Presented at the 15th annual meeting of the Cervical Spine Research Society, Washington D.C., December 5, 1987.
28. Freeman T.B., Martinez C.R. Radiological evaluation of cervical spondylotic disease: Limitations of magnetic resonance imaging for diagnosis and preoperative assessment. Perspect. Neurol. Surg.. 1992;3:34-54.
29. Penning L., Wilmink J.T., van Woerden H.H., Knol E. CT myelographic. findings in degenerative disorders of the cervical spine: clinical significance. AJNR Am. J. Roentgenol.. 1986;146(4):793-801.
30. Statham P.F., Hadley D.M., MacPherson P., Johnston R.A., Bone I., Teasdale G.M. MRI in the management of suspected cervical myelopathy. J. Neurol. Neurosurg. Psychiatry.. 1991;54:484-489.
31. Larsson E.M., Holtas S., Cronqvist S., Brandt L. Comparison of myelography, CT myelography and magnetic resonance imaging in cervical spondylosis and disc herniation. Acta. Radiol.. 1989;30:233-239.
32. Takahashi M., Sakamoto Y., Miyawaki M., Bussaka H. Increased signal intensity secondary to chronic cervical cord compression. Neuroradiology. 1987;29:550-556.
33. Al-Mefty O., Harkey H.L., Marawi I., Haines D.E., Peeler D., Wilner H.I., Smith R.R., Holaday H.R., Haining J.L., Russell W.F., et al. Experimental chronic compressive cervical myelopathy. J. Neurosurg.. 1993;79:550-561.
34. Takahashi M., Yamashita Y., Sakamoto Y., Kojima R. Chronic cervical cord compression: Clinical significance of increased signal intensity on MR images. Radiology. 1989;173:219-224.
35. Matsuda Y., Miyazaki K., Tada K., Yasuda A., Nakayama T., Murakami H., Matsuo M. Increased MR signal intensity due to cervical myelopathy. J Neurosurg. 1991;74:887-892.
36. Mehalie T.F., Pezzuti R.T., Applebaum B.L. Magnetic resonance imaging and cervical spondylotic myelopathy. Neurosurgery. 1990;26(2):217-226. Discussion 226-227
37. Yone K., Sakou T., Yanase M., Ljiri K. Preoperative and postoperative magnetic resonance image evaluations of the spinal cord in cervical myelopathy. Spine. 1992;17:S387-S392.
38. Al-Mefty O., Harkey L.H., Middleton T.H., Smith R.R., Fox J.L. Myelopathic cervical spondylotic lesions demonstrated by magnetic resonance imaging. J Neurosurg. 1988;68:217-222.
39. Teresi L.M., Lufkin R.B., Reicher M.A., Moffit B.J., Vinuela F.V., Wilson G.M., Bentson J.R., Hanafee W.N. Asymptomatic degenerative disc disease and spondylosis of the cervical spine: MR imaging. Radiology. 1987;164:83-88.
40. Clements D.H., O’Leary P.F. Anterior cervical discectomy and fusion for the treatment of cervical radiculopathy. In: Camins M.B., O’Leary P.F., editors. Disorders of the cervical spine. Baltimore: Williams & Wilkins, 1992.
41. Leblhuber F., Reissecker F., Boehm-Jurkovic H., Witzmann A., Deisenhammer E. Diagnostic value of different electrophysiologic tests in cervical disc prolapse. Neurology. 1988;38:1879-1881.
42. Yu Y.L., Jones S.J. Somatosensory evoked potentials in cervical spondylosis. Brain. 1985;108:273-300.
43. De Noordhoot M., Remade J.M., Pepin J.L. Magnetic stimulation of the motor cortex in cervical spondylosis. Neurology. 1991;41:75-80.
44. Di Lazzaro V., Restucia D., Colosimo C., Tonali P. The contribution of magnetic stimulation of the motor cortex to the diagnosis of cervical spondylotic myelopathy: correlation of central motor conduction to distal and proximal upper limb muscles with clinical and MRI findings. Electroencephalogr. Clin. Neurophysiol.. 1992;85:311-320.
45. Travlos A., Pant B., Eisen A. Transcranial magnetic stimulation for detection of preclinical cervical spondylotic myelopathy. Arch. Physiol. Med. Rehab.. 1992;73:442-446.
46. Dvorak J., Herdmann J. Janssen, Theiler R, Grob D: Motor-evoked potentials in patients with cervical spine disorders. Spine. 1990;15:1013-1016.
47. Smith G.W., Robinson R.A. The treatment of certain cervical-spine disorders by anterior removal of the intervertebral disc and interbody fusion. J. Bone. Joint Surg.. 1958;40:607-624.
48. Scoville W.B., Dohrman G.J., Corkill G. Late results of cervical disc surgery. J. Neurosurg.. 1976;45:203-210.
49. Murphey F., Simmons J.C.H., Brunson B. Cervical treatment of laterally ruptured cervical discs: review of 648 cases, 1939-1972. J. Neurosurg.. 1973;38:679-683.
50. Raimondi A.J. Pediatric neurosurgery: theoretical principles, art of surgical techniques. New York: Springer, 1987.
51. Raynor R.B., Pugh J., Shapiro I. Cervical facetectomy and the effect on spine strength. J. Neurosurg.. 1985;63:278.
52. Hunter L.Y., Braunstein E.M., Bailey R.W. Radiographic changes following anterior cervical spine fusions. Spine. 1980;5:399-401.
53. Dillin W, Booth R, Cuckler J, et al: Cervical radiculopathy: a review, Spine 11(1986) 988-991.
54. Ebersolf M.J., Raynor R.B., Bovis G.K., et al. Cervical laminotomy, laminectomy, laminoplasty, and foraminotomy. In: Benzel E.C., editor. Spine surgery: techniques, complication avoidance and management. Philadelphia: Churchill Livingstone, 1999.
55. Clifton A.G., Stevens J.M., Whitear P. BE Kendall: Identifiable causes for poor outcome in surgery for cervical spondylosis: post-operative computed myelography and MR imaging. Neuroradiology. 1990;32(6):450-455.
56. Batzdorf U., Flannigan B.D. Surgical decompressive procedures for cervical spondylotic myelopathy: a study using magnetic resonance imaging. Spine. 1991;16:123-127.
57. Harada A., Mimatsu K. Postoperative changes in the spinal cord in cervical spondylotic myelopathy demonstrated by magnetic resonance imaging. Spine. 1992;17:1275-1280.
58. Grote W., Kalff R., Roosen K. Surgical treatment of cervical intervertebral disc displacement. Zentralbl Neurochir. 1991;52:101-108.
59. Phillips F.M., Carlson G., Emery S.E., et al. Anterior cervical pseudarthrosis: Natural history and treatment. Spine. 1997;22:1585-1589.
60. Sonntag V.K., Klara P. Controversy in spine care: Is fusion necessary after anterior cervical discectomy? Spine. 1996;21:1111-1113.
61. Abd-Alrahman N., Dokmak A.S., Abou-Madawi A. Anterior cervical discectomy (ACD) versus anterior cervical fusion (ACF), clinical and radiological outcome study. Acta. Neurochir. (Wien). 1999;141:1089-1092.
62. Bärlocher C.B., Barth A., Krauss J.K., et al. Comparative evaluation of microdiscectomy only, autograft fusion, polymethylmethacrylate interposition, and threaded titanium cage fusion for treatment of single-level cervical disc disease: A prospective study in 125 patients. Neurosurg Focus. 2002. 12 (Article 4)
63. Dowd G.C., Wirth F.P. Anterior cervical discectomy: Is fusion necessary? J. Neurosurg. Spine. 1999;90:8-12.
64. Martins A.N. Anterior cervical discectomy with and without interbody bone graft. J. Neurosurg.. 1976;44:290-295.
65. Rosenorn J., Hansen E.B., Rosenorn M.A. Anterior cervical discectomy with and without fusion: A prospective study. J. Neurosurg.. 1983;59:252-255.
66. Savolainen S., Rinne J., Hernesniemi J. A prospective randomized study of anterior single-level cervical disc operations with long-term follow-up: surgical fusion is unnecessary. Neurosurgery. 1998;43:51-55.
67. van den Bent M.J., Oosting J., Wouda E.J., et al. Anterior cervical discectomy with or without fusion with acrylate: a randomized trial. Spine. 1996;21:834-839.
68. Wirth F.P., Dowd G.C., Sanders H.F., et al. Cervical discectomy: a prospective analysis of three operative techniques. Surg. Neurol.. 2000;53:340-346.
69. Angevine P.D., Arons R.R., McCormick P.C. National and regional rates and variation of cervical discectomy with and without anterior fusion, 1990–1999. Spine. 2003;28:931-939.
70. Drew B., Bhandari M., Orr D., et al. Surgical preference in anterior cervical discectomy: a national survey of Canadian spine surgeons. J. Spinal Disord Tech.. 2002;15:454-457.
71. Zeidman S.M., Ducker T.B., Raycroft J. Trends and complications in cervical spine surgery: 1989–1993. J. Spinal Disord.. 1997;10:523-526.
72. An H.S., Simpson J.M., Glover J.M., et al. Comparison between allograft plus demineralized bone matrix versus autograft in anterior cervical fusion: a prospective multicenter study. Spine. 1995;20:2211-2216.
73. Bishop R.C., Moore K.A., Hadley M.N. Anterior cervical interbody fusion using autogeneic and allogeneic bone graft substrate: a prospective comparative analysis. J. Neurosurg.. 1996;85:206-210.
74. Fernyhough J.C., White J.I., LaRocca H. Fusion rates in multilevel cervical spondylosis comparing allograft fibula with autograft fibula in 126 patients. Spine. 1991;16:S561-S564.
75. Rish B.L., McFadden J.T., Penix J.O. Anterior cervical fusion using homologous bone grafts: a comparative study. Surg. Neurol.. 1976;5:119-121.
76. Savolainen S., Usenius J.P., Hernesniemi J. Iliac crest versus artificial bone grafts in 250 cervical fusions. Acta. Neurochir. (Wien). 1994;129:54-57.
77. Young W.F., Rosenwasser R.H. An early comparative analysis of the use of fibular allograft versus autologous iliac crest graft for interbody fusion after anterior cervical discectomy. Spine. 1993;18:1123-1124.
78. Floyd T., Ohnmeiss D. A meta-analysis of autograft versus allograft in anterior cervical fusion. Eur. Spine J.. 2000;9:398-403.
79. Wigfield C.C., Nelson R.J. Nonautologous interbody fusion materials in cervical spine surgery: how strong is the evidence to justify their use? Spine. 2001;26:687-694.
80. Madawi A.A., Powell M., Crockard H.A. Biocompatible osteoconductive polymer versus iliac graft: a prospective comparative study for the evaluation of fusion pattern after anterior cervical discectomy. Spine. 1996;21:2123-2129.
81. Senter H.J., Kortyna R., Kemp W.R. Anterior cervical discectomy with hydroxylapatite fusion. Neurosurgery. 1989;25:39-42.
82. Lowery G.L., McDonough R.F. The significance of hardware failure in anterior cervical plate fixation: patients with 2- to 7-year follow-up. Spine. 1998;23:181-186.
83. Rawlinson J.N. Morbidity after anterior cervical decompression and fusion: the influence of the donor site on recovery, and the results of a trial of surgibone compared to autologous bone. Acta. Neurochir. (Wien). 1994;131:106-118.
84. Hacker R.J., Cauthen J.C., Gilbert T.J., et al. A prospective randomized multicenter clinical evaluation of an anterior cervical fusion cage. Spine. 2000;25:2646-2654.
85. Moreland D.B., Asch H.L., Clabeaux D.E., et al. Anterior cervical discectomy and fusion with implantable titanium cage: initial impressions, patient outcomes and comparison to fusion with allograft. Spine J.. 2004;4:184-191.
86. Thomé C., Krauss J.K., Zevgaridis D. A prospective clinical comparison of rectangular titanium cages and iliac crest autografts in anterior cervical discectomy and fusion. Neurosurg. Rev.. 2004;27:34-41.
87. Wigfield C.C., Nelson R.J. Nonautologous interbody fusion materials in cervical spine surgery: how strong is the evidence to justify their use? Spine. 2001;26:687-694.
88. Bohler J. Immediate and early treatment of traumatic paraplegias [German]. Z. Orthop. Ihre. Grenzgeb.. 1967;103:512-529.
89. Caspar W., Barbier D.D., Klara P.M. Anterior cervical fusion and Caspar plate stabilization for cervical trauma. Neurosurgery. 1989;25:491-502.
90. Orozco Delclos R., Llovet Tapies J. Osteosintesis en las fracturas de raquis cervical: nota de tecnica, Rev. Ortop. Traumatol. Ed. Lat. Am.. 1970;14:285-288.
91. Paramore C.G., Dickman C.A., Sonntag V.K. Radiographic and clinical follow-up review of Caspar plates in 49 patients. J. Neurosurg.. 1996;84:957-961.
92. Morscher E., Sutter F., Jenny H., et al. Anterior plating of the cervical spine with the hollow screw-plate system of titanium. Chirurg. 1986;57:702-707.
93. Haid R.W., Foley K.T., Rodts G.E., et al. The Cervical Spine Study Group anterior cervical plate nomenclature. Neurosurg. Focus. 2002. 12 (Article 15)
94. Caspar W., Geisler F.H., Pitzen T., et al. Anterior cervical plate stabilization in one- and two-level degenerative disease: overtreatment or benefit? J. Spinal Disord.. 1998;11:1-11.
95. Wang J.C., McDonough P.W., Endow K.K., et al. Increased fusion rates with cervical plating for two-level anterior cervical discectomy and fusion. Spine. 2000;25:41-45.
96. Geer C.P., Selden N.R.W., Papadopoulos S.M. Anterior cervical plate fixation in the treatment of single-level cervical disc disease (abstract, paper #722). J. Neurosurg.. 1999;90:410A.
97. Katsuura A., Hukuda S., Imanaka T., et al. Anterior cervical plate used in degenerative disease can maintain cervical lordosis. J. Spinal Disord.. 1996;9:470-476.
98. Troyanovich S.J., Stroink A.R., Kattner K.A., et al. Does anterior plating maintain cervical lordosis versus conventional fusion techniques? A retrospective analysis of patients receiving single-level fusions. J. Spinal. Disord. Tech.. 2002;15:69-74.
99. Katsuura A., Hukuda S., Saruhashi Y., et al. Kyphotic malalignment after anterior cervical fusion is one of the factors promoting the degenerative process in adjacent intervertebral levels. Eur. Spine J.. 2001;10:320-324.
100. Zoega B., Karrholm J., Lind B. One-level cervical spine fusion: a randomized study, with or without plate fixation, using radiostereometry in 27 patients. Acta Orthop. Scand.. 1998;69:363-368.
101. Zoega B., Karrholm J., Lind B. Plate fixation adds stability to two-level anterior fusion in the cervical spine: A randomized study using radiostereometry. Eur. Spine J.. 1998;7:302-307.
102. Bazaz R., Lee M.J., Yoo J.U. Incidence of dysphagia after anterior cervical spine surgery: a prospective study. Spine. 2002;27:2453-2458.
103. Baskin D.S., Ryan P., Sonntag V., et al. A prospective, randomized, controlled cervical fusion study using recombinant human bone morphogenetic protein-2 with the CORNERSTONE-SR allograft ring and the ATLANTIS anterior cervical plate. Spine. 2003;28:1219-1225.
104. Bose B. Anterior cervical arthrodesis using DOC dynamic stabilization implant for improvement in sagittal angulation and controlled settling. J. Neurosurg. Spine. 2003;98:8-13.
105. Connolly P.J., Esses S.I., Kostuik J.P. Anterior cervical fusion: outcome analysis of patients fused with and without anterior cervical plates. J. Spinal Disord.. 1996;9:202-206.
106. Grob D., Peyer J.V., Dvorak J. The use of plate fixation in anterior surgery of the degenerative cervical spine: a comparative prospective clinical study. Eur. Spine J. 2001;10:408-413.
107. Kaiser M.G., Haid R.W.Jr., Subach B.R., et al. Anterior cervical plating enhances arthrodesis after discectomy and fusion with cortical allograft. Neurosurgery. 2002;50:229-236.
108. Madawi A.A., Powell M., Crockard H.A. Biocompatible osteoconductive polymer versus iliac graft: a prospective comparative study for the evaluation of fusion pattern after anterior cervical discectomy. Spine. 1996;21:2123-2129.
109. Shapiro S., Connolly P., Donnaldson J., et al. Cadaveric fibula, locking plate, and allogeneic bone matrix for anterior cervical fusions after cervical discectomy for radiculopathy or myelopathy. J. Neurosurg. Spine. 2001;95:43-50.
110. McLaughlin M.R., Purighalla V., Pizzi F.J. Cost advantages of two-level anterior cervical fusion with rigid internal fixation for radiculopathy and degenerative disease. Surg. Neurol.. 1997;48:560-565.
111. Lowery G.L., Reuter M.W., Sutterlin C.E. Anterior cervical interbody arthrodesis with plate stabilization for degenerative disc disease (abstract). Orthop. Trans.. 1994;18:345.
112. Wigfield C., Gill S., Nelson R., et al. Influence of an artificial cervical joint compared with fusion on adjacent-level motion in the treatment of degenerative cervical disc disease. J. Neurosurg. Spine. 2002;96:17-21.
113. Gore D.R., Sepic S.B. Anterior discectomy and fusion for painful cervical disc disease: a report of 50 patients with an average follow-up of 21 years. Spine. 1998;23:2047-2051.
114. Hilibrand A., Carlson G., Palumbo, et al. Radioculopathy and myelopathy at segments adjacent to the site of a previous anterior cervical arthrodesis. J. Bone Joint. Surg.. 1999;81A:519-528.
115. Wigfield C., Gill S., Nelson R., et al. Influence of an artificial cervical joint compared with fusion on adjacent-level motion in the treatment of degenerative cervical disc disease. J. Neurosurg. Spine. 2002;96:17-21.
116. Cummins B.H., Robertson J.T., Gill S.S. Surgical experience with an implanted artificial cervical joint. J. Neurosurg.. 1998;88:943-948.
117. Goffin J., Casey A., Kehr P., et al. Preliminary clinical experience with the Bryan cervical disc prosthesis. Neurosurgery. 2002;51:840-845.
118. Jollenbeck B., Hahne R., Schubert A., et al. Early experiences with cervical disc prostheses. Zentralbl Neurochir. 2004;65:123-127.
119. Heller J.G., Sasso R.C., Papadopoulos S.M., et al. Comparison of BRYAN cervical disc arthroplasty with anterior cervical decompression and fusion: clinical and radiographic results of a randomized, controlled, clinical trial. Spine (Phila Pa 1976). 2009;34(2):101-107.
120. Mummaneni P.V., Robinson J.C., Haid R.W.Jr. Cervical arthroplasty with the PRESTIGE LP cervical disc. Neurosurgery. 2007;60(4 Suppl. 2):310-314. discussion 314-315
121. Murrey D., Janssen M., Delamarter R., et al. Results of the prospective, randomized, controlled multicenter Food and Drug Administration investigational device exemption study of the ProDisc-C total disc replacement versus anterior discectomy and fusion for the treatment of 1-level symptomatic cervical disc disease. Spine J.. 2009;9(4):275-286.