Chapter 140 Cervical Spine Construct Design
Fundamental Concepts
Benzel1 described an excellent method for preoperative mapping of thoracic and lumbar instrumentation procedures, using a “construct blueprint.” This approach is practical in this region of the spine, because the choice of implant components that may be applied here is vast. The design of thoracolumbar constructs entails the selection of the longitudinal member, cross-fixation mechanism, and implant-bone junction fixators. Each element may be different at various levels of a long construct, adding to the complexity of the system.
Additionally, the modes of possible construct application for the thoracolumbar spine are extensive. This refers to the desired forces that are applied by the surgeon at the implant-bone junction. Constructs may be placed in compression, distraction, neutral, translation, flexion, extension, and lateral-bending modes.2 In a single thoracolumbar construct, several modes of application may be required, depending on the structural demands at any given level. A systematic approach to the formulation of an operative plan is essential when designing constructs with this degree of complexity. The construct blueprint is a concise format capable of communicating complicated surgical strategies to all members of the surgical team.
Indications for Cervical Construct Application
White and Hirsch3 outline four general indications for spinal stabilization: (1) to restore clinical stability to a spine in which the structural integrity has been compromised, (2) to maintain alignment after correction of a deformity, (3) to prevent progression of a deformity, and (4) to alleviate pain. Cervical spinal instrumentation may be applied in conjunction with a bone fusion in all of these scenarios. In rare instances, instrumentation may replace bone fusion as the principal means of cervical stabilization.
Optimally, internal fixation provides immediate postoperative stability to the region before the development of osseous fusion. This is beneficial in two respects. Instrumentation protects the neural elements from trauma and the spine from deformity until the bony fusion matures and can assume this role. Internal fixation also obviates, or at least significantly reduces, the requirement for postoperative external immobilization while the fusion mass heals. This technique improves patient comfort, which encourages accelerated mobilization after surgery. Additionally, this may enhance the probability of attaining successful bone fusion by ensuring compliance with postoperative immobilization.
Clinical Instability
The most frequent indication for cervical instrumentation is instability. To paraphrase an oft-quoted general definition, instability requires the loss of spinal biomechanical integrity such that the spine is unable to prevent initial or additional neurologic deficit, major deformity, or incapacitating pain under physiologic loads.3 The precise definition of spinal instability is difficult to establish and may vary according to the specific clinical setting.
Maintenance of Alignment
Prevention of spinal deformity may also be accomplished by the timely use of internal fixation. Progressive kyphosis or spondylolisthesis may result from spinal decompression procedures. If individuals at risk for this complication are identified preoperatively, cervical deformity may be preventable. Patients exhibiting a loss of the normal cervical lordotic configuration are prone to develop postlaminectomy kyphosis.4 This complication may be avoided by proper internal stabilization at the time of decompression. Similarly, operative resections that compromise principal load-bearing elements may render the spine incompetent to withstand physiologic loads. Progressive postoperative deformity may be prevented by spinal reconstruction, using bone graft and instrumentation to reconstitute the axial spine.
Construct Selection
General Considerations
In most cases cervical constructs are used to maintain clinical stability. This may be accomplished most efficiently by matching the implant with the major site of instability. That is, if the instability is primarily dorsal in location, a dorsal construct should be considered for stabilization. Similarly, ventral instability, created by incompetence of the anterior longitudinal ligament (ALL), vertebral body, or intervertebral disc complex, is most effectively treated by the application of a ventral construct. It is unreasonable to expect that a construct will function with optimal stability when implanted in a biomechanically disadvantageous position.
Internal fixation systems provide immediate postoperative stability to the instrumented region but do not provide long-term stability due to the “plastic” properties of bone at the implant-bone interface. As with most biologic materials, bone deforms and reforms in response to stress.5 Eventually, even the most rigid construct allows a small degree of motion. Repetitive loading gradually increases the amount of movement and can ultimately lead to implant failure, unless bony fusion occurs. The long-term stability of all constructs thus depends on osseous fusion. No internal fixation system currently available can compensate for a poorly designed bone graft.6
Cervical spine implants may be considered as rigid, semirigid, or dynamic.5 Rigid implants attempt to achieve complete immobilization of the instrumented motion segments. Ventral plate systems, with locking screws and dorsal screw-rod and hook-rod systems, provide rigid fixation. Luque rods and rectangles (Zimmer, Warsaw, IN), secured with segmental sublaminar or facet wires, and most lateral mass plate devices are examples of semirigid cervical implants. Rigid immobilization may be potentially detrimental to bone fusion because of stress shielding and stress-reduction osteopenia.5,7 This concern has led to the development of dynamic instrumentation, such as nonfixed moment arm cantilever beam screw-plate implants and axially dynamic ventral fixators.8
Modes of Application
The modes of application available for cervical constructs are more limited than those available for use in other spinal regions. Thoracolumbar implants may be placed in distraction, compression, neutral, translation, flexion, extension, and lateral-bending modes. In contrast, cervical spine constructs are generally applied in the neutral mode. This is not universally true, because certain cervical plate systems and wire constructs may provide a modest degree of compression. Theoretically, cervical rod-hook devices can be placed in the compression or distraction modes as well. However, the vast majority of cervical constructs in clinical use are applied in the neutral mode at the time of surgery. Biomechanical conditions change as the spine is loaded after surgery. Most “neutral” implants must resist axial compression when the upright posture is assumed. These constructs then function in a distraction mode.5
Cervical construct designs are also more limited in their mechanism of load bearing than their thoracolumbar counterparts. Generally, cervical constructs conform to one of five fundamental load-bearing types: (1) distraction fixation, (2) tension band fixation, (3) three-point bending, (4) fixed moment arm cantilever beam, and (5) nonfixed moment arm cantilever beam fixation.2 Applied moment arm cantilever beam fixation, a technique occasionally applied in the thoracolumbar spine, is not used in the cervical spine. Assigning an implant to one of these fundamental load-bearing types is somewhat artificial, because a given construct may exhibit features of several mechanical types. However, it permits classification of implants by their principal biomechanical attributes.
Simple Distraction
Simple distraction fixation occurs when a distraction force is applied by a cervical construct, usually from a ventral, interbody location.2 Interbody strut grafts, with or without ventral plate instrumentation, are examples of this type of fixation. These devices principally resist axial loads. Dorsally applied distraction fixation is rarely used because it is prone to create a kyphotic deformity.
Tension Band Fixation
Tension band fixation is accomplished by any device that reconstitutes the ventral or dorsal tension band, thereby preventing distraction, and also possibly angulation, in the opposite direction. This type of fixation may be applied dorsally with interspinous wires or cables, sublaminar wires or cables, facet wires or cables, interlaminar clamps, or lateral mass plates. A hook-rod construct, applied in compression, also produces tension band fixation. These dorsal devices resist flexion most efficiently, because the flexion moment is coupled with dorsal distraction. Ventral tension band fixation is accomplished principally with ventral cervical plate systems. These implants reconstitute the ventral tension band, thereby resisting ventral distraction and providing sound biomechanical stabilization of extension injuries.6
Three-Point Bending
Three-point bending fixation occurs when forces are applied to the spine at three or more sites along the length of the construct.2 Dorsally directed forces are applied at the rostral and caudal ends of the construct. An equal but opposite ventrally directed force is applied at the fulcrum, usually in the center of the construct. Three-point bending instrumentation is most often utilized dorsally in the cervical spine and includes fixation of multiple motion segments. Three-point bending forces may be applied with Luque rods and rectangles secured with sublaminar wires or cables, hook-rod implants, and, to a lesser degree, with lateral mass screw-plate or screw-rod instrumentation.
Cantilever Beam Fixation
A cantilever is formed by a projecting beam supported at one end only.2 When the cantilever is rigidly attached to the supporting longitudinal member, a fixed moment arm cantilever beam is created. This variety of load bearing is accomplished by ventral cervical plate systems secured with locking screws and rigid lateral mass rod-screw instrumentation. A fixed moment arm cantilever beam device contributes some axial load-sharing properties to the construct. Nonfixed moment arm cantilever beam fixation employs a dynamic attachment of the cantilever to the longitudinal member. Lateral mass plates and nonfixed moment arm cantilever beam screw-plate implants and axially dynamic ventral fixators are representative of this type of load bearing.
The classification of spinal implants by a mechanism of load bearing is somewhat artificial. In practice a single implant may function by using several of the fundamental load-bearing mechanisms simultaneously. For example, the lateral mass plate is capable of stabilization by three such mechanisms. Dorsal tension band, three-point bending, and nonfixed moment arm cantilever beam fixation are all accomplished by this device.
Construct Materials
Bone Grafts
Autograft and allograft bone have both been used extensively in spinal stabilization. Some studies report that fusion rates with allograft bone are comparable to those obtained with autograft bone.9–11 Other studies have maintained that autograft bone is superior.3,12,13 This is particularly evident with dorsal cervical constructs, in which the bone graft is not placed under compression. Certainly, fusion rates with autograft bone meet or exceed those reported with allograft. The use of autograft bone eliminates the concern of infectious disease transmission (including HIV and hepatitis virus transmission) that may be associated with allograft bone.
The iliac crest provides a versatile and abundant source of bone graft material for incorporation into cervical spine constructs. Favorable attributes of this type of graft include ease of procurement in both the supine and prone positions, strength, and relative expendability of the donor site.3,14 The tricortical structure of the iliac crest is responsible for much of the strength inherent in this graft, thereby providing excellent axial load-bearing capability. The abundant cancellous bone provides ample substrate for osseous remodeling. Although all commonly used configurations of iliac crest grafts can sustain high compressive loads, the Smith-Robinson–type graft is probably superior to other styles of grafts in this respect.15 The principal disadvantage associated with iliac crest harvest is donor site morbidity, which may be substantial. Complications include pain, wound hematoma, infection, meralgia paresthetica, hip dislocation, and fracture of the anterior superior iliac spine.
Fibula is another commonly used site for graft material. It is particularly well suited for multilevel ventral reconstruction procedures, because the thick cortical bone in this graft resists high axial compressive loads. The relatively small amount of cancellous bone present in the fibula graft may delay bone remodeling, however. This may be partially overcome by packing additional cancellous bone in the center of the graft, as well as surrounding the outer cortical surface with the cancellous bone. Donor site morbidity arising from graft harvest may be significant, because one sixth of body weight is borne by the fibula.5 This may be principally a theoretical concern, however, as fibular bone has been used quite successfully in many cases of spinal reconstruction.
Rib grafts have also been used, particularly with dorsal cervical constructs. The native configuration of rib is advantageous because it conforms well to the cervical lordotic curve. There is minimal morbidity in harvesting rib compared with iliac crest. This is an excellent graft to use for dorsal fusions.16
Implants
Regardless of the material used, compatibility of the implanted components is essential. All metal implants should be made of the same material. This eliminates the theoretical possibility of internal current generation that may cause corrosion. The size of implanted components should also be compatible. Fixators at the implant-bone junction should be of appropriate diameter, length, and configuration to match the longitudinal member.6
The integrity of the patient’s native bone is an important factor. Bone quality can affect construct selection, the biomechanical stability of a construct, and the need for postoperative external immobilization. Osteoporosis is detrimental to all forms of spinal fixation. It influences systems that rely on screw fixation most substantially. Hooks and sublaminar wires are less prone to pull-out than screws and thus may be more suited for use in the osteoporotic patient.1 Poor bone quality may necessitate incorporation of additional levels into a construct to promote load sharing and enhance stability.
Construct Application
Cervical spine integrity may be restored by either ventral or dorsal stabilization techniques. The application of both may be indicated in cases of severe instability creating a “360-degree” construct. The rationale for selecting one approach over another is case dependent and relies on the degree and extent of instability. If the site of major instability is ventral, a ventral construct should be created to restore structural integrity to the ventral spine. Dorsal instability is treated most effectively through dorsal stabilization. This general rule is valid for all causes of cervical instability. The underlying disease process does influence the selection of specific construct components and the method by which they are applied.
Ventral Constructs
Interbody Strut Graft
By definition, a simple interbody strut graft implanted after a ventral cervical discectomy constitutes a ventral cervical construct. Larger grafts are often used for vertebral body replacement after corpectomy for trauma, neoplasia, and spondylotic disease. Ventral strut grafts function predominantly in the simple distraction mode, reconstituting the ventral load-bearing column of the cervical spine. This construct offers excellent resistance to axial compressive loads (Fig. 140-1). It also imparts some stability in flexion, extension, axial rotation, and lateral bending.13 In most cases, however, immediate postoperative stability is not provided with a simple strut graft.
Some means of fixation, whether external or internal, is usually required to provide temporary stability until osseous fusion occurs. The extent of supplemental fixation is dictated by the degree of instability that remains after placement of the bone graft. The instability created by a single-level ventral cervical discectomy may be managed adequately with interbody strut graft placement and immobilization in a cervical collar. More significant instability requires more rigid fixation while the fusion matures. This may be accomplished internally with instrumentation or externally with an orthosis. In the setting of multilevel corpectomy, some studies suggest that ventral corpectomy with instrumentation be supplemented with dorsal instrumentation to prevent postoperative graft and instrumentation complications.17,18 However, this is a point of controversy; others have advocated ventral cervical fixation as sufficient for multilevel corpectomy up to four levels.19
Ventral Cervical Plate and Screw Constructs
Ventral cervical plate and screw constructs were developed to provide immediate internal stability before osseous integration of a strut graft. When used in this context, these devices often eliminate the need for postoperative external bracing. All ventral plate constructs reconstitute the ventral tension band, thereby providing the most stabilization in extension.20 Some of these devices also provide fixed moment arm cantilever beam fixation, thereby sharing some of the axial load with the strut graft. Rigid implants with fixed-angle locking screw mechanisms function in this capacity. Plating systems that use variable-angle screws or translationally mobile screws are more dynamic implants and provide less axial load sharing. These devices act as nonfixed moment arm cantilever beam fixators in addition to their tension band attributes (Fig. 140-2). Dynamic implants theoretically allow for the graft to be exposed to continuous axial loading, which may facilitate bone fusion.8
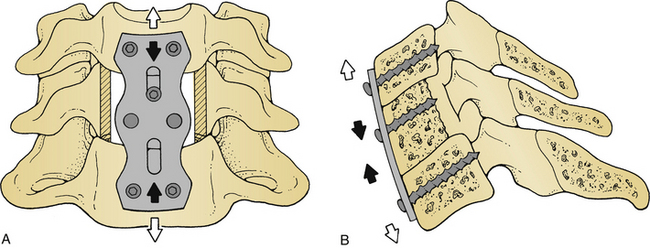
FIGURE 140-2 Coronal (A) and lateral (B) views of a ventral cervical plate (bicortical, unlocked) construct. The plate-screw device reconstitutes the ventral tension band (black arrows), thereby resisting ventral distraction and extension (white arrows). Axial compressive forces (not shown) are resisted by the strut graft, as depicted in Figure 140-1.
Biomechanical studies have demonstrated that ventral plates can restore stability to the injured spine in essentially all motion planes, although this is most significant in flexion and extension.13,21 An interbody bone graft must supplement the instrumentation to effectively stabilize an injured motion segment. The load-bearing capacities of ventral cervical plates are temporary, so all plated segments must be fused to achieve long-term stability.
Ventral cervical plates are affixed with screws at the implant-bone junction. Some devices use screws with bicortical purchase, whereas others use unicortical fixation. Bicortical screw purchase confers greater holding strength to the construct.11 Placement of bicortical screws is slightly more perilous than implantation of unicortical screws, because the dorsal cortex of the vertebral body must be drilled to accept these screws. To avoid drilling into the spinal canal and traumatizing the spinal cord, bicortical screw placement must be monitored using fluoroscopy.22
Ventral cervical plate systems are extremely versatile. They provide substantial immediate postoperative stability, limit the extent of instrumentation, and facilitate aggressive reconstruction of the ventral spine. They enhance fusion rates and permit early patient mobilization. As with all devices that use screw fixation, the performance of ventral plating systems is Y-fixation. Supplemental fixation or additional bracing may be necessary in the setting of osteoporotic bone.
Cervical cages are another means of stabilizing the anterior column. These devices may be made of titanium, carbon, or other materials. Threaded cages appear to provide greater initial stiffness than the nonthreaded devices.23–25 The results from acute biomechanical testing may be misleading since subsequent subsidence may lead to a subsequent decrease in stiffness.
Dorsal Constructs
Wire Constructs
Dorsal stabilization with wire or braided cables usually entails incorporation of the spinous processes or articular facets, with or without bone autograft. These constructs function primarily by reconstituting the dorsal tension band (Fig. 140-3). Dorsal wire constructs provide some stability in flexion, minimal stability in extension, and add little to rotatory or translatory stability.26 If translational instability exists, dorsal tension band fixation implants may be inadequate to prevent the “parallelogram effect.”3 This may result in translatory displacement and further spinal deformity. Wiring alone does not provide sufficient immediate internal stability in most cases. It must be supplemented with bone graft, methyl methacrylate, or external bracing to augment the construct until bony fusion occurs. Still, this is an inexpensive, rapid, and relatively safe method to reconstitute the dorsal tension band, particularly in cases of isolated dorsal ligamentous injury.
Interlaminar Clamps
The dorsal tension band may also be re-created by application of interlaminar clamps. These devices are used rarely because they are somewhat unwieldy to apply and may be hazardous.5 These clamps function by reconstituting the dorsal tension band and may be adequate to restrict flexion. No stability is provided in extension or axial rotation. Extension is prevented by placing the bone graft between the spinous processes. Interlaminar clamps require intact laminae at the levels to be instrumented. They are also prone to experience the parallelogram effect if translatory instability is present.
Luque L-Rods and Rectangles
Originally used for thoracolumbar instability, Luque L-rods and rectangles may also be incorporated into dorsal cervical constructs. These devices are usually applied over multiple spinal segments and are secured with sublaminar or facet wires. Alternatively, braided cables may be used to affix the construct at the implant-bone junction. They act principally as semirigid implants, reconstructing the dorsal tension band. Additionally, they provide a significant degree of three-point bending fixation (Fig. 140-4). These implants stabilize in flexion, extension, and lateral bending modes.
Lateral Mass Fixation
Dorsal cervical stabilization has been revolutionized by the development of lateral mass screw fixation systems. These include screws fixed to plates and screws fixed to rods. These devices provide a high degree of immediate internal stability, often eliminating the need for postoperative external immobilization or bracing. Lateral mass plates are dynamic implants and behave primarily as nonfixed moment arm cantilever beam fixators. They also provide some dorsal tension band fixation (Fig. 140-5). Biomechanical studies have demonstrated the ability of these devices to restore stiffness to the injured spine in flexion, extension, and torsion.27,28 Similar to other constructs that restore the dorsal tension band, lateral mass plates are probably weakest in extension.5,24 Lateral mass screw-rod systems are rigid devices and as such provide more stability than screw-plate systems.
Lateral mass fixation may be used to treat instability from C2 to T1. Posttraumatic instability provides the most common indication for the use of lateral mass plates.26,29 Dorsal ligamentous injury and irreducible unilateral or bilateral facet dislocations may be stabilized effectively with this type of construct after reduction of malalignment. In these cases instrumentation across the affected segment alone is usually adequate to restore stability. A longer construct may be required if instability of a single motion segment is severe, or if the dorsal elements at the level of instability are not intact. Multiple segments of instability may also mandate instrumentation of additional levels to achieve an adequate biomechanical advantage.
Lateral mass fixation may also be used to reestablish stability after spinal tumor resection. The extent of instrumentation depends on the size and site of the tumor, but in general, several segments above and below the affected levels should be incorporated.6 Lateral mass screws should be placed only in bone that is free of disease.
Excellent results have been reported using lateral mass plates without fusion.26,29 However, long-term structural stability is augmented by incorporating bone graft into the construct. This may be accomplished by denuding the articular processes at the unstable level(s) and packing cancellous bone graft into the joint space. Often, adequate material for bone autograft may be obtained from adjacent spinous processes or from bone removed during decompression.
Cervical hook-rod systems have many of the same advantages of lateral mass plates but may be less easy to apply. Hook constructs are probably not the best choice in the setting of stenosis since the hooks require some space within the canal. Polyaxial screws allow the lateral mass screws to be applied in the appropriate trajectory, with the appropriately conformed rod applied after screw application. This is in contrast to lateral mass plates, in which the shape of the plate and placement of screw holes can occasionally dictate screw placement. As mentioned earlier, these systems provide rigid fixation that is biomechanically superior to that of the semirigid lateral mass plates.30
Cervical pedicle fixation has also been shown to provide appropriate dorsal stabilization for the cervical spine.31–33 However, cervical pedicle screws are significantly more difficult to place than lateral mass screws, and they carry a higher risk of injury to the nerve root and the vertebral artery. Lateral mass screws are probably equally effective in biomechanical stabilization and are safer in most situations.
360-Degree Constructs
A methyl methacrylate strut may be used as a vertebral body replacement. More commonly, fibular strut grafts are used ventrally to span multiple vertebral levels. Struts function in a simple distraction mode, effectively resisting compressive axial loads (Fig. 140-6A). This can be supplemented by threaded Kirschner wires (K-wires), which are embedded into the vertebral bodies above and below the involved region. These devices prevent strut migration and provide some resistance to translatory forces.
A dorsal polymethylmethacrylate (PMMA) or fibular strut construct should be applied, in conjunction with ventral stabilization, which completes the 360-degree construct. Several techniques have been described for application of this material to the dorsal cervical spine. With one such method, K-wires are passed through the spinous processes, are bent, and then are encased in PMMA. These wires provide an increased surface area for interdigitation junction bonding between bone, wire, and cement.3 The net result is an increase in the tensile load-bearing capacity of the construct.
The dorsal methyl methacrylate-wire construct functions primarily as a dorsal tension band fixator (Figs. 140-6B and 104-6C). Some three-point bending fixation is also provided. When applied in this manner, 360-degree methyl methacrylate constructs provide a substantial degree of immediate internal stability in virtually all motion planes.34 It must be remembered that, in contrast to an osseous fusion, the strength of a methyl methacrylate construct is maximal initially and decreases over time. This type of construct is generally reserved for treatment of cervical instability associated with progressive spinal malignancy. This is a purely palliative procedure and is not intended for long-term stabilization. It should be used only in patients with a limited life expectancy. Methyl methacrylate has been used for the treatment of traumatic instability,34 although this material probably should be avoided because other constructs are superior in this context.
Ventral and dorsal cervical plating systems may be applied concurrently in conjunction with appropriate bone grafting. In most cases these devices should confer an optimal biomechanical advantage to the construct, providing immediate internal stability in all motion planes. Other constructs may be devised if screw fixation is contraindicated by poor bone quality. These situations are rare and necessitate individualized management. However, fundamental construct design concepts should guide the selection and application of hardware systems, just as in less complex problems of instability.
Economic Considerations
Historically, economic considerations have not been major determinants in the process of construct design. This is no longer true because rising health care costs and declining reimbursement mandate some fiscal responsibility. Material costs represent only a fraction of the expense accrued with spinal instrumentation. Surgeons’ fees, operative time, anesthesia support, and fluoroscopy costs (if required) all reflect the complexity of a stabilization procedure. Thousands of dollars may be expended to apply a single construct. With this in mind, it is financially irresponsible to implant an elaborate, costly system when a less expensive alternative could suffice. However, the structural integrity of a construct should not be compromised for purely economic concerns. The spine surgeon must use restraint in the construct design process, minimizing expenses when possible.
Supplementary External Immobilization
The need for postoperative external immobilization may be reduced or eliminated when internal fixation provides immediate stability to a construct. Orthoses are selected in accordance with the nature and extent of preoperative instability, quality of the construct, cause of instability, and extent of residual disease. Young patients with isolated dorsal instability can be treated with a soft cervical collar for 4 weeks after most instrumentation procedures. Patients with more severe instability or residual disease require more aggressive postoperative bracing. A hard cervical collar is probably adequate in most cases. Those with osteoporosis, severe preoperative instability, and/or biomechanically inferior constructs that do not provide sufficient immediate internal stability require a postoperative halo vest or Minerva immobilization.6 Regardless of the bracing method, all patients should be assessed often with serial examinations and radiographs until an osseous fusion is attained.
Albert T.J., Vacarro A. Postlaminectomy kyphosis. Spine. 1998;23:2738-2745.
Gill K., Paschal S., Corin J., et al. Posterior plating of the cervical spine. A biomechanical comparison of different posterior fusion techniques. Spine. 1988;13:813-816.
Roy-Camille R., Saillanr G., Mazel C. Internal fixation of the unstable cervical spine by posterior osteosynthesis with plates and screws. In: The Cervical Spine Research Society Editorial Committee, editor. The cervical spine. ed 2. Philadelphia: Lippincott-Raven; 1989:390-404.
Ryken T.C., Goel V.K., Clausen J.D., et al. Assessment of unicortical and bicortical fixation in a quasistatic cadaveric model. Role of bone mineral density and screw torque. Spine (Phila Pa 1976). 1995;20:1861-1867.
Traynelis V.C. Anterior and posterior plate stabilization of the cervical spine. Neurosurgery. 1992;2:59-76.
Traynelis V.C., Donaher P.A., Roach R.M., et al. Biomechanical comparison of anterior Caspar plate and three level posterior fixation techniques in a human cadaveric model. J Neurosurg. 1993;79:96-103.
Vaccaro A.R., Falatyn S.P., Scuderi G.J., et al. Early failure of long segment anterior cervical plate fixation. J Spinal Disord. 1998;11:410-415.
1. Benzel E.C. Construct design. In: Benzel E.C., editor. Biomechanics of spine stabilization: principles and clinical practice. New York: McGraw-Hill; 1995:163-172.
2. Benzel E.C. Mechanical (quantitative) attributes of spinal implants: construct types. In: Benzel E.C., editor. Biomechanics of spine stabilization: principles and clinical practice. New York: McGraw-Hill; 1995:151-162.
3. White A.A., Hirsch C. An experimental study of the immediate load bearing capacity of some commonly used iliac bone grafts. Acta Orthop Scand. 1971;42:482-490.
4. Albert T.J., Vacarro A. Postlaminectomy kyphosis. Spine (Phila Pa 1976). 1998;23:2738-2745.
5. Benzel E.C. Qualitative attributes of spinal implants. In: Benzel E.C., editor. Biomechanics of spine stabilization: principles and clinical practice. New York: McGraw-Hill; 1995:135-150.
6. Traynelis V.C. Anterior and posterior plate stabilization of the cervical spine. Neurosurgery. 1992;2:59-76.
7. McAfee P.C., Farey I.D., Sutterlin C.E., et al. Device-related osteoporosis with spinal instrumentation. Spine (Phila Pa 1976). 1989;14:919-926.
8. Haid R.W., Foley K.T., Rodts G.E., et al. The Cervical Spine Study Group anterior cervical plate nomenclature. Neurosurg Focus. 2002;12:E15.
9. Cloward R. Gas-sterilized cadaver bone grafts for spinal fusion operations. A simplified bone bank. Spine (Phila Pa 1976). 1980;5:4-10.
10. Nasca R.J., Whelchel J.D. Use of cryopreserved bone in spinal surgery. Spine (Phila Pa 1976). 1987;12:222-227.
11. Ryken T.C., Goel V.K., Clausen J.D., et al. Assessment of unicortical and bicortical fixation in a quasistatic cadaveric model. Role of bone mineral density and screw torque. Spine (Phila Pa 1976). 1995;20:1861-1867.
12. Stabler C.L., Eismont F.J., Brown M.D., et al. Failure of posterior cervical fusions using cadaver bone graft in children. J Bone Joint Surg [Am]. 1985;67:370-375.
13. Schulte K., Clark C.R., Goel V.K. Kinematics of the cervical spine following discectomy and stabilization. Spine (Phila Pa 1976). 1989;14:1116-1121.
14. White A.A., Hirsch C. An experimental study of the immediate load bearing capacity of some commonly used iliac bone grafts. Acta Orthop Scand. 1971;42:482-490.
15. Zhang Z.H., Yin H., Yang K., et al. Anterior intervertebral disc excision and bone grafting in cervical spondylotic myelopathy. Spine (Phila Pa 1976). 1983;8:16-19.
16. Sawin P.D., Traynelis V.C., Menezes A.H. A comparative analysis of fusion rates and donor-site morbidity for autogeneic rib and iliac crest bone grafts in posterior cervical fusions. J Neurosurg. 1998;88:255-265.
17. Riew K.D., Sethi N.S., Devney J., et al. Complications of buttress plate stabilization of cervical corpectomy. Spine (Phila Pa 1976). 1999;24:2404-2410.
18. Vaccaro A.R., Falatyn S.P., Scuderi G.J., et al. Early failure of long segment anterior cervical plate fixation. J Spinal Disord. 1998;11:410-415.
19. Fessler R.G., Barua M., Traynelis V.C. Anterior cervical plate stabilization following multilevel corpectomy. Monterey, CA: Presented at the Cervical Spine Research Society; November 2001.
20. de Oliveira J.C. Anterior plate fixation of traumatic lesions of the lower cervical spine. Spine (Phila Pa 1976). 1987;12:324-329.
21. Traynelis V.C., Donaher P.A., Roach R.M., et al. Biomechanical comparison of anterior Caspar plate and three level posterior fixation techniques in a human cadaveric model. J Neurosurg. 1993;79:96-103.
22. Caspar W. Anterior cervical fusion and interbody stabilization with the trapezoidal osteosynthetic plate technique. Tuttlingen, Germany: Aesculap-Werke-AG; 1986. Aesculap Scientific Information
23. Assietti R., Beretta F., Arienta C. Two level anterior cervical discectomy and cage-assisted fusion without plates. Neurosurg Focus. 2002;12(1):E3.
24. Hacker R.J., Cauthen J.C., Gilbert T.J., et al. A prospective randomized multicenter clinical evaluation of an anterior cervical cage. Spine (Phila Pa 1976). 2000;25:2646-2655.
25. Salame K., Ouaknine G.E.R., Razon N., et al. The use of carbon fiber cages in anterior cervical interbody fusion. Report of 100 cases. Neurosurg Focus. 2002;12(1):E1.
26. Roy-Camille R., Saillanr G., Mazel C. Internal fixation of the unstable cervical spine by posterior osteosynthesis with plates and screws. In: The cervical spine Research Society Editorial Committee, editor. The cervical spine. ed 2. Philadelphia: Lippincott-Raven; 1989:390-404.
27. Coe J.D., Warden K.E., Sutterlin C.E.III, et al. Biomechanical evaluation of cervical spinal stabilization methods in a human cadaveric model. Spine (Phila Pa 1976). 1989;14:1122-1131.
28. Gill K., Paschal S., Corin J., et al. Posterior plating of the cervical spine. A biomechanical comparison of different posterior fusion techniques. Spine (Phila Pa 1976). 1988;13:813-816.
29. Fehlings M.G., Cooper P.R., Errico T.J. Posterior plates in the management of cervical instability: long-term results in 44 patients. J Neurosurg. 1994;81:341-349.
30. Mummaneni P.V., Haid R.W., Traynelis V.C., et al. Posterior cervical fixation using a new polyaxial screw and rod system: technique and surgical results. Neurosurg Focus. 2002;12(1):E8.
31. Abumi K., Kaneda K. Pedicle screw fixation for nontraumatic lesions of the cervical spine. Spine (Phila Pa 1976). 1997;22:1853-1863.
32. Abumi K., Kaneda K., Shono Y., et al. One-stage posterior decompression and reconstruction of the cervical spine by using pedicle screw fixation systems. J Neurosurg. 1999;90(Suppl 1):19-26.
33. Abumi K., Shono Y., Ito M., et al. Complications of pedicle screw fixation in reconstructive surgery of the cervical spine. Spine (Phila Pa 1976). 2000;25:962-969.
34. Branch C.L., Kelly D.L., Davis C.H., et al. Fixation of fractures of the lower cervical spine using methylmethacrylate and wire: technique and results in 99 patients. Neurosurgery. 1989;25:503-513.