35 Cerebrovascular disease
Introduction
Cerebral infarcts become swollen after a few days because of osmotic activity. Some become large enough to produce distance effects by causing subfalcal or tentorial herniation of the brain in the manner of a tumor (Ch. 4).
Anterior Circulation of the Brain
Clinicians refer to the ICA and its branches as the anterior circulation of the brain, and the vertebrobasilar system (including the posterior cerebral arteries) as the posterior circulation. The anterior and posterior circulations are connected by the posterior communicating arteries (Figure 35.2).
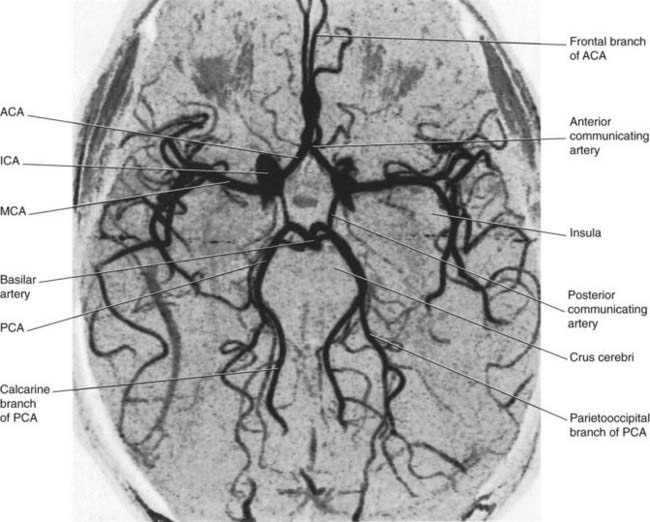
Figure 35.2 Circle of Willis and its branches. This is an magnetic resonance (MR) angiogram based on the principle that flowing blood generates a different signal to that of stationary tissue, without injection of a contrast agent. Conventional angiograms, e.g. those in Chapter 5, require arterial perfusion with a contrast agent. The vessels shown here are contained within a single thick MR ‘slice’. Some, e.g. the calcarine branch of the posterior cerebral artery, could be followed further in adjacent slices. ACA, anterior cerebral artery; ICA, internal carotid artery; MCA, middle cerebral artery; PCA, posterior cerebral artery.
(From a series kindly provided by Professor J. Paul Finn, Director, Magnetic Resonance Research, Department of Radiology, David Geffen School of Medicine at UCLA, California, USA.)
About 75% of cerebrovascular accidents (CVAs) originate in the anterior circulation.
Internal capsule
The following details supplement the account of the arterial supply of the internal capsule in Chapter 5.
The blood supply of the internal capsule is shown in Figure 35.3. The three sources of supply are the anterior choroidal, a direct branch of the internal carotid; the medial striate, a branch of the anterior cerebral, and lateral striate (lenticulostriate) branches of the middle cerebral artery.
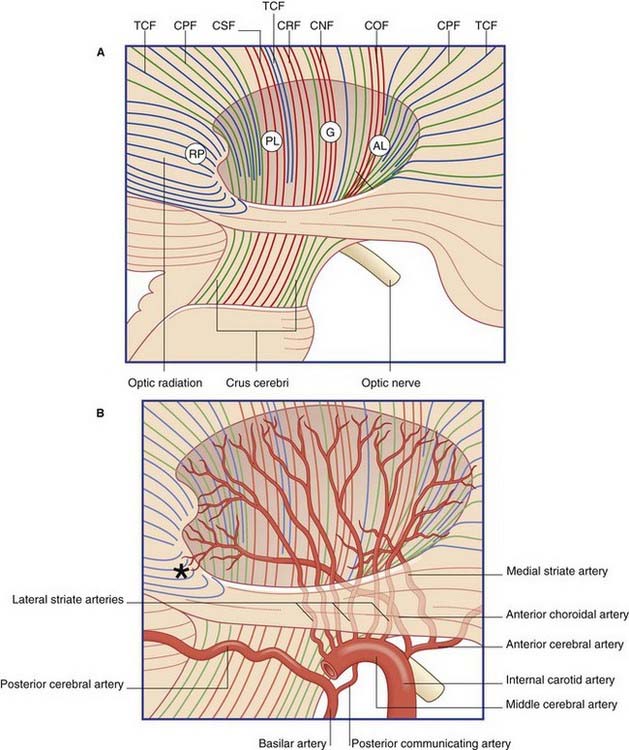
Figure 35.3 Internal capsule.
(A) Pathways. Lateral view of the right cerebral hemisphere, showing the oval depression in the white matter following removal of the lentiform nucleus. The internal capsule occupies the floor of the depression. CNF, corticonuclear fibers; COF, cortico-oculomotor fibers; CPF, corticopontine fibers; CRF, corticoreticular fibers; CSF, corticospinal fibers; TCF, thalamocortical fibers. Other abbreviations as in Figure 35.4.
The contents of the internal capsule are shown in Figure 35.4. The anterior choroidal branch of the internal carotid artery supplies the lower part of the posterior limb and the retrolentiform part of the internal capsule, and the inferolateral part of the lateral geniculate body. Some of its branches (not shown) supply a variable amount of the temporal lobe of the brain and the choroid plexus of the inferior horn of the lateral ventricle.
Posterior Circulation of the Brain
Additional information is confined to the stem branches of the posterior cerebral artery shown in Figure 35.5.
Transient Ischemic Attacks
Clinical Anatomy of Vascular Occlusions
In the Clinical Panels, the term occlusion encompasses all causes of regional arterial failure other than aneurysms. Symptoms of occlusions within the anterior circulation are summarized in Clinical Panels 35.1–35.4, within the posterior circulation in Clinical Panel 35.5, specifically within the terrirory of the posterior cerebral artery in Clinical Panel 35.6. Subarachnoid hemmorrhage is considered in Clinical Panel 35.7.
Clinical Panel 35.1 Anterior choroidal artery occlusion
A complete anterior choroidal artery syndrome is produced by occlusion of the proximal part of the artery, compromising the lower part of the posterior limb and retrolentiform part of the internal capsule. The clinical picture is one of contralateral hemiparesis, hemisensory loss of cortical type (Ch. 29), and hemianopia. Damage to the (crossed) cerebellothalamocortical pathway may add evidence of intention tremor in the contralateral upper limb, yielding so-called ataxic hemiparesis.
Clinical Panel 35.2 Anterior cerebral artery occlusion