Chapter 2 Cells, Tissues, and Organs of the Immune System
• Most cells of the immune system derive from hemopoietic stem cells. The primary lymphoid organs in mammals are the thymus and bone marrow, where lymphocyte differentiation occurs.
• Phagocytic cells are found in the circulation as monocytes and granulocytes. Monocytes differentiate into macrophages that reside in tissues (e.g. Kupffer cells in the liver). Neutrophils are short-lived phagocytes present in high numbers in the blood and at sites of acute inflammation.
• Eosinophils, basophils, mast cells, and platelets, together with cytokines and complement, take part in the inflammatory response.
• NK cells recognize and kill virus-infected cells and certain tumor cells by inducing apoptosis.
• Antigen-presenting cells link the innate and adaptive immune systems and are required by T cells to enable them to respond to antigens.
• Lymphocytes are heterogeneous phenotypically, functionally, and morphologically.
• B lymphocytes and T lymphocytes express specific antigen receptors called the B cell receptor (BCR) and T cell receptor (TCR) respectively.
• There are three major subpopulations of T cells which have helper, cytotoxic and regulatory activities (TH, TC and Treg).
• B cells can differentiate into antibody-secreting plasma cells and memory cells.
• T cells developing in the thymus are subject to positive and negative selection processes.
• Mammalian B cells develop mainly in the fetal liver and from birth onwards in the bone marrow. This process continues throughout life. B cells also undergo a negative selection process at the site of B cell generation.
• Lymphocytes migrate to, and function in, the secondary lymphoid organs and tissues.
• Secondary lymphoid organs and tissue protect different body sites – the spleen responds to blood borne organisms; the lymph nodes respond to lymph-borne antigens; and the mucosa-associated lymphoid tissue (MALT) protects the mucosal surfaces.
• Most lymphocytes recirculate around the body; there is continuous lymphocyte traffic from the blood stream into lymphoid tissues and back again into the blood via the thoracic duct and right lymphatic duct.
Cells of the immune system
There is great heterogeneity in the cells of the immune system, most of which originate from hematopoietic stem cells in the fetal liver and in the postnatal bone marrow – mainly in the vertebrae, sternum, ribs, femur and tibia (Fig. 2.1). This morphological heterogeneity reflects the fact that cells of the immune system are called on to provide a wide variety of functions including:
Cells of the innate immune system include monocytes/macrophages, polymorphonuclear granulocytes, NK cells, mast cells, and platelets
Phagocytic cells of the innate immune system belong to the myeloid lineage and include:
• the monocytes: circulating blood cells;
• the macrophages: differentiated from monocytes and residing in various tissues;
• the polymorphonuclear granulocytes (polymorphonuclear neutrophils [PMNs], basophils, and eosinophils): circulating blood cells.
All phagocytic cells are mainly involved in defense from extracellular microbes.
Mast cells and platelets are pivotal in inducing and maintaining inflammation.
Microbes express various cell surface and intracellular molecules called pathogen-associated molecular patterns (PAMPs). Cells of the innate system recognize microbes through their receptors to PAMPs called pattern recognition receptors (PRR). PRR have broad specificity and a non-clonal distribution, features which distinguish them from the specific antigen receptors of the adaptive immune system (see Chapter 6).
Antigen-presenting cells (APCs) link the innate and adaptive immune systems
A specialized group of cells termed antigen-presenting cells (APCs) link the innate and adaptive immune systems by taking up and processing antigens so they can be recognized by T cells, and by producing cytokines. APCs enhance innate immune cell function and they are essential for activation of T cells (Fig. 2.2).
Adaptive immune system cells are lymphocytes
Lymphocytes (T and B cells) recognize antigens through clonally expressed, highly specific antigen receptors (see Chapters 3 and Chapter 5). T cells are produced in the thymus (see Fig. 2.1) and require antigen to be processed and presented to them by specialized APCs.
• the thymus – the site of T cell development;
• the fetal liver and postnatal bone marrow – the sites of B cell development.
Myeloid cells
Mononuclear phagocytes and polymorphonuclear granulocytes are the two major phagocyte lineages
• the neutrophils, also called polymorphonuclear neutrophils (PMNs), are most numerous and constitute the majority of leukocytes (white blood cells) in the blood stream (around 60–70% in adults);
• the primary actions of eosinophils and basophils, which can both function as phagocytes, involve granule release (exocytosis).
The mononuclear phagocytes and polymorphonuclear granulocytes develop from a common precursor.
Mononuclear phagocytes are widely distributed throughout the body
Cells of the mononuclear phagocytic system are found in virtually all organs of the body where the local microenvironment determines their morphology and functional characteristics, e.g. in the lung as alveolar macrophages, in kidney as glomerular mesangial cells, and in the liver as Kupffer cells (Fig. 2.3 and see Fig. 1.2).
• is large (10–18 μm in diameter) relative to the lymphocyte;
• has a horseshoe-shaped nucleus;
• contains primary azurophilic (blue-staining) granules; and
• possesses ruffled membranes, a well-developed Golgi complex, and many intracytoplasmic lysosomes (Fig. 2.4).
Microbial adherence occurs through pattern recognition receptors (see Chapters 6 and 7), followed by phagocytosis. Coating microbes with complement components and/or antibodies (opsonization) enhances phagocytosis by monocytes/macrophages and is mediated by specialized complement receptors and antibody receptors expressed by the phagocytic cells (see Chapters 3 and 4).
There are three different types of polymorphonuclear granulocyte
• are released from the bone marrow at a rate of around 7 million per minute;
• are short-lived (2–3 days) relative to monocytes/macrophages, which may live for months or years.
Like monocytes, PMNs marginate (adhere to endothelial cells lining the blood vessels) and extravasate by squeezing between the endothelial cells to leave the circulation (see Fig. 1.17) to reach the site of infection in tissues. This process is known as diapedesis. Adhesion is mediated by receptors on the granulocytes and ligands on the endothelial cells, and is promoted by chemo-attractants (chemokines) such as interleukin-8 (IL-8) (see Chapter 6).
The importance of granulocytes is evident from the observation of individuals who have a reduced number of white cells or who have rare genetic defects that prevent polymorph extravasation in response to chemotactic stimuli (see Chapter 16). These individuals have a markedly increased susceptibility to bacterial and fungal infection.
Neutrophils comprise over 95% of the circulating granulocytes
Neutrophils have a characteristic multilobed nucleus and are 10–20 μm in diameter (Fig. 2.5). Chemotactic agents attracting neutrophils to the site of infection include:
• protein fragments released when complement is activated (e.g. C5a);
• factors derived from the fibrinolytic and kinin systems;
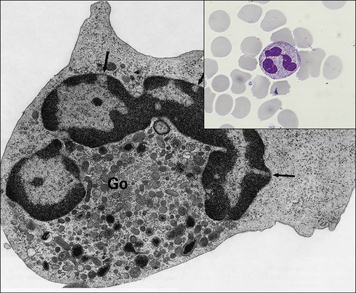
Fig. 2.5 Morphology of the neutrophil
(From Zucker-Franklin D, Grossi CE, eds. Atlas of blood cells: function and pathology, 3rd edn. Milan: Edi Ermes; 2003.)
• the primary (azurophilic) granules are lysosomes containing acid hydrolases, myeloperoxidase, and muramidase (lysozyme); they also contain the antimicrobial proteins including defensins, seprocidins, cathelicidins, and bacterial permeability inducing (BPI) protein; and
• the secondary granules (specific to neutrophils) contain lactoferrin and lysozyme (see Fig. 2.5).
Neutrophils can also release granules and cytotoxic substances extracellularly when they are activated by immune complexes (antibodies bound to their specific antigen molecules) through their Fc receptors. This is an important example of collaboration between the innate and adaptive immune systems, and may be an important pathogenetic mechanism in immune complex diseases (type III hypersensitivity, see Chapter 25).
Granulocytes and mononuclear phagocytes develop from a common precursor
Studies in which colonies have been grown in vitro from individual stems cells have shown that the progenitor of the myeloid lineage (CFU-GEMM) can give rise to granulocytes, monocytes and megakaryocytes (Fig. 2.6). Monocytes and neutrophils develop from a common precursor cell, the CFU-granulocyte macrophage cells (CFU-GMs) (see Fig. 2.6). Myelopoiesis (the development of myeloid cells) commences in the liver of the human fetus at about 6 weeks of gestation.
CFU-GEMMs mature under the influence of colony-stimulating factors (CSFs) and several interleukins (see Fig. 2.6). These factors, which are relevant for the positive regulation of hemopoiesis, are:
• derived mainly from stromal cells (connective tissue cells) in the bone marrow;
• also produced by mature forms of differentiated myeloid and lymphoid cells.
Neutrophils express adhesion molecules and receptors involved in phagocytosis
Other surface molecules acquired during the differentiation process include:
• adhesion molecules (e.g. the leukocyte integrins CD11a, b, and c, associated with CD18 β2 chains); and
• receptors involved in phagocytosis including complement and antibody Fc receptors.
Neutrophils constitutively express FcγRIII and FcγRII, and FcγRI is induced on activation.
Activation of neutrophils by cytokines and chemokines is also a prerequisite for their migration into tissues (see Chapter 9).
Eosinophils, basophils, mast cells and platelets in inflammation
Eosinophils are thought to play a role in immunity to parasitic worms
Eosinophils comprise 2–5% of blood leukocytes in healthy, non-allergic individuals. Human blood eosinophils usually have a bilobed nucleus and many cytoplasmic granules, which stain with acidic dyes such as eosin (Fig. 2.7). Although not their primary function, eosinophils appear to be capable of phagocytosing and killing ingested microorganisms.
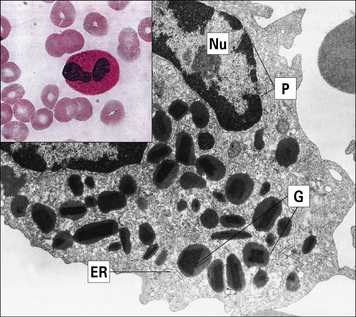
Fig. 2.7 Morphology of the eosinophil
(From Zucker-Franklin D, Grossi CE, eds. Atlas of blood cells: function and pathology, 3rd edn. Milan: Edi Ermes; 2003.)
The granules in mature eosinophils are membrane-bound organelles with crystalloid cores that differ in electron density from the surrounding matrix (see Fig. 2.7). The crystalloid core contains the major basic protein (MBP), which:
• is a potent toxin for helminth worms;
• induces histamine release from mast cells;
Other proteins with similar effects are found in the granule matrix, for example:
Release of the granules on eosinophil activation is the only way in which eosinophils can kill large pathogens (e.g. schistosomula), which cannot be phagocytosed. Eosinophils are therefore thought to play a specialized role in immunity to parasitic worms using this mechanism (see Fig. 15.13).
Basophils and mast cells play a role in immunity against parasites
Basophils are found in very small numbers in the circulation and account for less than 0.2% of leukocytes (Fig. 2.8).
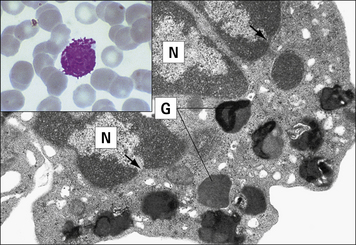
Fig. 2.8 Morphology of the basophil
(Adapted from Zucker-Franklin D, Grossi CE, eds. Atlas of blood cells: function and pathology, 3rd edn. Milan: Edi Ermes; 2003.)
The mast cell (Fig. 2.9), which is present in tissues and not in the circulation, is indistinguishable from the basophil in a number of its characteristics, but displays some distinctive morphological features (Fig. 2.10). Their shared functions may indicate a convergent differentiation pathway.
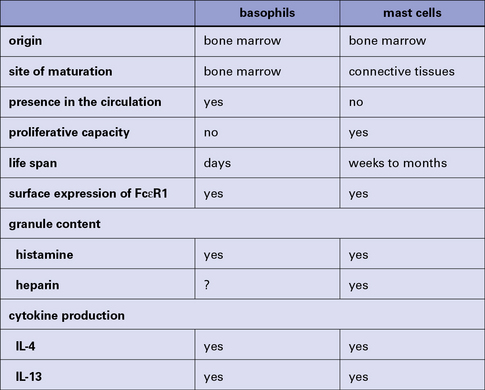
Fig. 2.10 Some distinctive and common characteristics of basophils and mast cells
Some distinctive and common characteristics of basophils and mast cells.
The stimulus for mast cell or basophil degranulation is often an allergen (i.e. an antigen causing an allergic reaction). To be effective, an allergen must cross-link IgE molecules bound to the surface of the mast cell or basophil via its high-affinity Fc receptors for IgE (FcεRI). Degranulation of a basophil or mast cell results in all contents of the granules being released very rapidly. This occurs by intracytoplasmic fusion of the granules, followed by discharge of their contents (Fig. 2.11).
Platelets have a role in clotting and inflammation
Blood platelets (Fig. 2.12) are not cells, but cell fragments derived from megakaryocytes in the bone marrow. They contain granules, microtubules, and actin/myosin filaments, which are involved in clot contraction. Platelets also participate in immune responses, especially in inflammation.
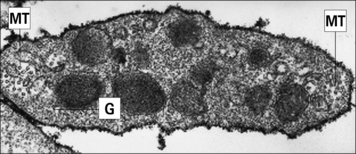
Fig. 2.12 Ultrastructure of a platelet
(Adapted from Zucker-Franklin D, Grossi CE, eds. Atlas of blood cells: function and pathology, 3rd edn. Milan: Edi Ermes; 2003.)
• receptors for clotting factors (e.g. factor VIII); and
• other molecules important for their function, such as the GpIIb/IIIa complex (CD41) responsible for binding to fibrinogen, fibronectin, vitronectin (tissue matrix), and von Willebrand factor (another clotting factor).
Both receptors and adhesion molecules are important in the activation of platelets.
NK cells
NK cells account for up to 15% of blood lymphocytes and express neither T cell nor B cell antigen receptors. They are derived from the bone marrow and morphologically have the appearance of large granular lymphocytes (see Fig. 2.19).
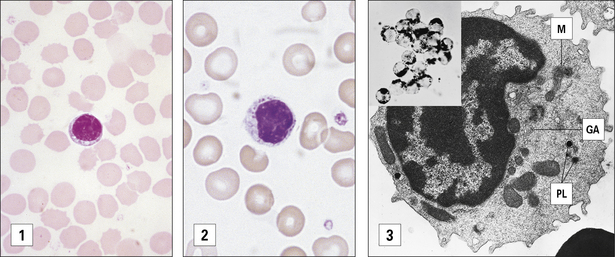
Fig. 2.19 Morphological heterogeneity of lymphocytes
((1) Adapted from Zucker-Franklin D, Grossi CE, eds. Atlas of blood cells: function and pathology, 3rd edn. Milan: Edi Ermes; 2003. (2) Courtesy of Dr A Stevens and Professor J Lowe.)
Nevertheless many surface markers are shared with T cells, monocytes/macrophages or neutrophils.
CD16 and CD56 are important markers of NK cells
Resting NK cells also express the β chain of the IL-2 receptor, and the signal transducing common γ chain of IL-2 and other cytokine receptors (see Fig. 8.18). Therefore, direct stimulation with IL-2 activates NK cells.
The function of NK cells is to recognize and kill virus-infected cells (Fig. 2.13) and certain tumor cells by mechanisms described in chapter 10.
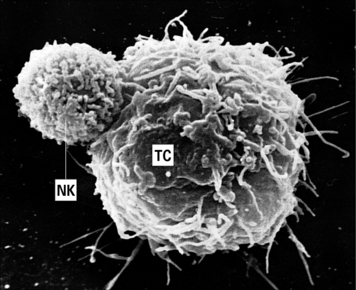
Fig. 2.13 An NK cell attached to a target cell
An NK cell (NK) attached to a target cell (TC). × 4500.
(Courtesy of Dr G Arancia and W Malorni, Rome.)
Classical and non-classical MHC class I molecules (see Fig. 5.15) are ligands for inhibitory receptors on the NK cells which prevent killing and this explains why normal body cells (all of which normally express MHC class I molecules) are not targeted by NK cells.
Antigen presenting cells
APCs are a heterogeneous population of leukocytes that are important in innate immunity (see Fig. 2.2) and play a pivotal role in the induction of functional activity of T helper (TH) cells.
Both macrophages and B cells are rich in membrane MHC class II molecules, especially after activation, and are thus able to process and present specific antigens to (activated) T cells (see Chapter 8).
Somatic cells other than immune cells do not normally express class II MHC molecules, but cytokines such as IFNγ and tumor necrosis factor-α (TNFα) can induce the expression of class II molecules on some cell types, and thus allow them to present antigen (non-professional APCs). This induction of ‘inappropriate’ class II expression might contribute to the pathogenesis of autoimmune diseases and to prolonged inflammation (see Chapter 20).
Dendritic cells are derived from several different lineages
Functionally, dendritic cells (DC) are divided into those that both process and present foreign protein antigens to T cells – ‘classical’ dendritic cells (DCs) – and a separate type that passively presents foreign antigen in the form of immune complexes to B cells in lymphoid follicles – follicular dendritic cells (FDCs; Fig. 2.14).
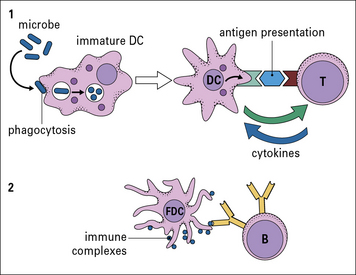
Fig. 2.14 Different kinds of antigen-presenting cells (APCs)
There are two main types of dendritic cells – classical DC and follicular dendritic cells (FDCs). (1) Immature DCs are derived from bone marrow and interact mainly with T cells. They are highly phagocytic, take up microbes, process the foreign microbial antigens into small peptides, and become mature APCs carrying the processed antigen (a peptide) on their surface with specialized MHC molecules. Specific T cells recognize the displayed peptide in a complex with MHC and, in the presence of cytokines produced by the mature DC, proliferate and also produce cytokines. (2) FDCs are not bone marrow derived and interact with B cells. In the B cell follicles of lymphoid organs and tissues they bind small immune complexes (IC, called iccosomes). Antigen contained within the IC is presented to specific B cells in the lymphoid follicles. This protects the B cell from cell death. The B cell then proliferates and with T cell help can leave the follicle and become a plasma cell or a memory cell (see Fig. 2.48).
Most DCs derive from one of two precursors:
• a myeloid progenitor (DC1) that gives rise to myeloid DCs, otherwise called bone-marrow derived or bm-DCs; and
• a lymphoid progenitor (DC2) that develops into plasmacytoid DCs (pDCs).
A summary of the main properties of myeloid and plasmacytoid dendritic cells is shown in Figure 2.15.
BM-DCs express various receptors that are involved in antigen uptake:
• C-type lectin receptors – for glycosyl groups, e.g. macrophage mannose receptor (MMR) family;
• receptors for heat shock protein–peptide complexes;
• receptors for apoptotic corpses;
Before DCs take up antigen (become loaded) they are called immature DCs and express various markers characteristic for this, resting, stage, the most important being chemokine receptors CCR1, CCR5 and CCR6. DCs are attracted to the infection site by chemokines through these receptors (see Chapter 6).
Mature DCs loaded with antigen down-regulate expression of CCR1, 5, 6 and up-regulate CCR7. This encourages their migration from various tissues into peripheral lymphatics, where CCR7 interacts with secondary lymphoid tissue chemokine SLC (CCL21) expressed on vascular endothelium (see Fig. 6.15).
Langerhans’ cells and interdigitating dendritic cells are rich in MHC class II molecules
Langerhans’ cells in the epidermis and in other squamous epithelia migrate via the afferent lymphatics into the paracortex of the draining lymph nodes (Fig. 2.16). Here, they interact with T cells and are termed interdigitating cells (IDCs, Fig. 2.17). These DCs are rich in class II MHC molecules, which are important for presenting antigen to helper T cells.
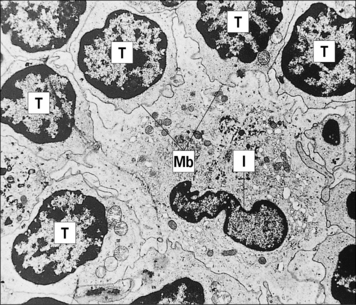
Fig. 2.17 Ultrastructure of an interdigitating dendritic cell (IDC) in the T cell area of a rat lymph node
(Courtesy of Dr BH Balfour.)
BM-DCs are also present within the germinal centers (GCs) of secondary lymphoid follicles (i.e. they are the MHC class II molecule-positive germinal center DCs [GCDCs]). In contrast to FDCs, they are migrating cells, which on arrival in the GC interact with germinal center T cells and are probably involved in antibody class switching (see Chapter 9).
The thymus is of crucial importance in the development and maturation of T cells. In thymus there are cortical DCs and IDCs which are especially abundant in the medulla (see Fig. 2.16). They participate in two important stages in T cell maturation/differentiation in thymus positive and negative selection respectively (see below).
FDCs lack class II MHC molecules and are found in B cell areas
Unlike the APCs that actively process and present protein antigens to T cells, FDCs have a passive role in presenting antigen in the form of immune complexes to B cells. They are therefore found in the primary and secondary follicles of the B cell areas of the lymph nodes, spleen, and MALT (see Fig. 2.16). They are a non-migratory population of cells and form a stable network (a kind of web) by establishing strong intercellular connections via desmosomes.
FDCs lack class II MHC molecules, but bind antigen via complement receptors (CD21 and CD35), which attach to complement associated with immune complexes (iccosomes; Fig. 2.18). They also express Fc receptors. The FDCs produce chemokines that are important in homing of B cells to the follicular areas in lymphoid tissues. They are not bone marrow derived, but are of mesenchymal origin.
Lymphocytes
Lymphocytes are phenotypically and functionally heterogeneous
Many mature lymphoid cells are long-lived, and persist as memory cells for many years.
Lymphocytes are morphologically heterogeneous
Two distinct morphological types of lymphocyte are seen in the circulation as determined by light microscopy and a hematological stain such as Giemsa (Fig. 2.19):
• the first type is relatively small, is typically agranular and has a high nuclear to cytoplasmic (N:C) ratio (Fig. 2.19[1]);
• the second type is larger, has a lower N:C ratio, contains cytoplasmic azurophilic granules, and is known as the large granular lymphocyte (LGL).
Most T helper (TH) cells (approximately 95%) and a proportion (approximately 50%) of cytotoxic T cells (TC or CTL) have the morphology shown in Figure 2.19(1).
The LGL morphological pattern displayed in Figure 2.19(2) is shown by less than 5% of TH cells and by about 30–50% of TC cells. These cells display LGL morphology with primary lysosomes dispersed in the cytoplasm and a well-developed Golgi apparatus, as shown in Figure 2.19(3).
Most B cells, when resting, have a morphology similar to that seen in Figure 2.19(1) under light microscopy.
Lymphocytes express characteristic surface and cytoplasmic markers
Lymphocytes (and other leukocytes) express a large number of different functionally important molecules mostly on their surfaces but also in their cytoplasm, which can be used to distinguish (‘mark’) cell subsets. Many of these cell markers can be identified by specific monoclonal antibodies (mAb) and can be used to distinguish T cells from B cells (Fig. 2.20).
• one integrin subfamily (the β2-integrins) uses CD18 as the β chain, which can be associated with CD11a, CD11b, CD11c, or αd – these combinations make up the lymphocyte function antigens LFA-1, Mac-1 (CR3), p150, 95, and αdβ2 surface molecules respectively – and are commonly found on leukocytes;
• a second subfamily (the β1-integrins) has CD29 as the β chain, which again is associated with various other peptides and includes the VLA (very late activation) markers.