Chapter 10 Cell-mediated Cytotoxicity
• Cell-mediated cytoxicity is an essential defence against intracellular pathogens, including viruses, some bacteria and some parasites.
• CTLs and NK cells are the lymphoid effectors of cytotoxicity. Most CTLs are CD8+ and respond to non-self antigens presented on MHC class I molecules. Some virally infected and cancerous cells try to evade the CTL response by downregulating MHC class I. NK cells recognize these MHC class I negative targets.
• NK cells recognize cells that fail to express MHC class I. NK cells express a variety of inhibitory receptors that recognize MHC class I molecules. When these receptors are not engaged, the NK cell is activated. Killer Immunoglobulin-like Receptors (KIRs) recognize classical MHC class I molecules. CD94 interacts with HLA-E. LILRB1 recognizes a wide range of class I molecules.
• Cancerous and virally infected cells express ligands for the activating receptor NKG2D. Stressed cells, including cancerous and virally infected cells, upregulate ULBP1–3, MICA and MICB, which are ligands for NKG2D. This results in NK cell activation.
• NK cells can also mediate ADCC.
• The balance of inhibitory and activating signals determines NK cell activation.
• Cytotoxicity is effected by direct cellular interactions, granule exocytosis and cytokine production. Fas ligand and TNF can induce apoptosis in the target cell. Granules containing perforin and granzymes are also released. Perforin forms a pore in the cell membrane, allowing granzymes access to the cytosol. Granzymes trigger the cell’s intrinsic apoptosis pathways.
• Macrophages, neutrophils and eosinophils are non-lymphoid cytotoxic effectors. Macrophages and neutrophils usually destroy pathogens by phagocytosis, but can also sometimes release the contents of their granules into the extracellular environment. Eosinophils release cytotoxic granules in response to antibody-coated cells.
Cytotoxic lymphocytes
Several types of cells have cytotoxic potential, including:
CTLs and NK cells mediate cytotoxicity
T cells and NK cells belong to the lymphoid lineage, and are more closely related to each other than they are to B cells. CTLs and NK cells both induce apoptosis in their targets by production of TNF family molecules, cytokines and cytotoxic granules, but they recognize their targets in different ways. CTLs recognize foreign antigens being presented on MHC class I, whereas NK cells respond to cells that fail to express class I. NK cells are also able to recognize stressed or antibody-coated targets directly (Fig. 10.1).
CTLs recognize antigen presented on MHC class I molecules
Additional interactions may be required to stabilize the bond between a CTL and its target. Like CD4+ T cells, CD8+ CTLs form an immunological synapse with their target. Signaling molecules including the TCR and CD3 are found in the central zone of the supra-molecular activation cluster (cSMAC), while adhesion molecules segregate in the peripheral zone (pSMAC). In contrast to CD4+ T cells, the cSMAC of CTLs and NK cells is divided into signaling and secretory domains (Fig. 10.2). After signaling has occurred, the microtubule organizing center polarizes towards the synapse, directing cytotoxic granules to the secretory domain of the cSMAC. Early CTL signaling occurs within ten seconds of cell–cell contact and granule release follows some two minutes later.
Not all NK cells mediate cytotoxicity
• 90% of blood NK cells are CD56low (i.e. they have low expression of CD56). These cells contain cytotoxic granules and are effective killers;
• 10% of blood NK cells are CD56hi (i.e. they express high levels of CD56). These cells do not contain cytotoxic granules, but can respond to target cells by producing the TH1 cytokine IFNγ;
• NK cells present in the lymph nodes, liver, and lungs are also less cytotoxic than CD56low blood NK cells;
• NK cells found in the uterus do contain cytotoxic granules, but do not degranulate in response to target cells. Their function is likely to be the production of angiogenic factors and mediation of placental implantation;
• a recently described subset of NK-like cells found in MALT are not cytotoxic but do produce IL-22, which is important for mucosal integrity.
NK cell development
In mice, the bone marrow is essential for the production of NK cells, and a complete pathway of NK development has been described at this location. Human bone marrow does contain CD34+ hematopoietic progenitor cells that have the potential to differentiate into NK cells, but NK-committed progenitor cells have not been identified here. On the other hand, a complete scheme of NK cell development has been described in human, but not mouse, secondary lymphoid tissue. Circulating CD34+ cells are thought to be recruited from the blood to the lymph nodes, where they progress through an NK-committed immature stage to give rise to CD94+CD56hi mature NK cells, capable of effector functions (Fig. 10.w1). CD56hi cells can further develop into CD56low cells, which express KIRs and are more cytotoxic than CD56hi cells. A thymic pathway of NK cell development has also been described in both humans and mice. However, this pathway is clearly not required for NK cell production as athymic individuals have normal NK cell numbers and function.
For the acquisition of KIRs, which occurs at the final stage of development, as CD56hi NK cells become CD56low, contact with stromal cells is once again required. Defective Ly49 expression by NK cells in the Tyro/Axl/Mer triple knockout mouse suggests that this may be partially because there is a requirement for an interaction between Protein S or Gas6 on stroma and one of their receptors (Tyro, Axl or Mer) on developing NK cells. Stromal MHC class I molecules are also likely to be important for acquisition of KIR. Several of the genes that are required for immune recognition by NK cells are grouped together on the leukocyte receptor complex on the long arm of chromosome 19 (Fig. 10.w2).
NK cell receptors
NK cells recognize cells that fail to express MHC class I
NK cells express inhibitory receptors that bind to MHC class I molecules. When they encounter a target cell that is not expressing MHC class I, this inhibitory signal is lost and tonic activating signals cause the NK cell to degranulate or produce cytokines in response to the target cell. Interestingly, many of the inhibitory receptors expressed by NK cells also have activating counterparts, many of which recognize the same ligands but with lower affinity. The function of these activating receptors is not known (Fig. 10.3).
Killer immunoglobulin-like receptors recognize MHC class I
KIRs fall into two main subsets:
The KIRs are then further classified by whether they have a long (L) or short (S) cytoplasmic tail. KIRs with long tails are inhibitory and those with short tails are activating (Fig. 10.4). For example, the inhibitory receptor KIR2DL1 has two immunoglobulin domains and a long cytoplasmic tail. It binds alleles of HLA-C that have a lysine residue at position 80 (HLA-C2 alleles). Its activating counterpart, KIR2DS1 also binds HLA-C2 alleles, but with lower affinity. The ligands of most other activating KIRs are not yet known.
The lectin-like receptor CD94 recognizes HLA-E
There are at least six members of the NKG2 family (NKG2A-F), of which all except NKG2D associate with CD94. NKG2A-CD94 is an inhibitory receptor that blocks NK cell activation. By contrast, CD94-NKG2C is an activating receptor (Fig. 10.5). The ligand for both CD94-NKG2A and CD94-NKG2C is HLA-E, although the inhibitory CD94-NKG2A has greater affinity for HLA-E than its activating counterpart. The function of CD94-NKG2A is to allow NK cells to recognize and respond to cells, such as those that are virally infected or cancerous, that are expressing low levels of MHC class I molecules
The HLA-E gene locus encodes an MHC class I-like molecule. These are sometimes called non-classical class I molecules, or class Ib molecules, to distinguish them from the classical MHC molecules that present antigen to CTLs. The function of HLA-E is to present peptides from other MHC class I molecules. The leader peptides from other MHC molecules are transported to the endoplasmic reticulum and are loaded into the peptide binding groove of HLA-E molecules, stabilizing them and allowing them to be transported to the plasma membrane (Fig. 10.6). Cells lacking classical MHC class I molecules do not express HLA-E at the cell surface. Thus, surface HLA-E levels provide a sensitive mechanism for monitoring global MHC class I expression by the cell.
Q. Unlike the classical MHC class I molecules, HLA-E is not highly polymorphic. Additionally, the leader peptides of classical MHC class I molecules are very similar, regardless of the haplotype of the molecules. From these observations, what can you infer about immune regulation mediated by CD94-NKG2A?
NK cells are self-tolerant
• an NK cell lacking an inhibitory receptor that recognizes a self MHC class I molecule cannot carry out cytotoxicity or cytokine production in response to target cells;
• an NK receptor that has an activating receptor that recognizes a self MHC class I molecule is also unable to carry out effector functions. Such NK cells are referred to as ‘hyporesponsive’;
• hyporesponsive NK cells may still be able to carry out effector functions in some situations, such as when activated by IL-2.
Cancerous and virally-infected cells are recognized by NKG2D
Like other NKG2 receptors, NKG2D is a member of the C-type lectin receptor family, but unlike other NKG2 molecules, NKG2D does not associate with CD94, instead forming a disulphide-linked homodimer (Fig. 10.7). It is an activating receptor expressed by all circulating NK cells.
NK cells can also recognize antibody on target cells using Fc receptors
The Fc receptor CD16 (FcγRIII) is expressed by all CD56low NK cells, but not by CD56hi cells. CD16 binds antibody bound to target cells, activating the NK cell so that it degranulates, mediating antibody dependent cell-mediated cytotoxicity (ADCC) (Fig. 10.8). Historically, this was referred to as K cell activity, but this function may also be performed by other cell types with Fc receptors, including T cells. NK cell-mediated ADCC requires both an adaptive immune stimulus (cells coated with antibody) and an innate immune effector mechanism (NK cells) and is thus an example of cross-talk between the innate and adaptive immune systems.
The balance of inhibitory and activating signals determines whether an NK is activated
Both the KIRs and CD94-associated lectin-like receptors occur as inhibitory or activating receptors.
• Inhibitory receptors contain an immunoreceptor tyrosine inhibitory motif (ITIM) in their cytoplasmic tails. These recruit inhibitory phosphatases, which disrupt phosphorylation of activating receptors and intracellular signaling molecules, and prevent NK cell activation. All NK cell inhibitory receptors contain an ITIM.
• CD94-NKG2C and activating KIR associate with intracellular proteins that have an immunoreceptor tyrosine activation motif (ITAM). This allows them to phosphorylate and recruit tyrosine kinases including ZAP-70, which lead to cellular activation. CD16, NKp46, NKp44 and NKp30 also associate with ITAM-bearing intracellular molecules.
• Some other activating receptors recruit an intracellular adaptor that does not bear an ITAM. For example, NKG2D recruits the adaptor molecule DAP10, which bears an ITAM-like YXXM motif.
It is not yet known precisely how the balance of activation and inhibition is resolved. To degranulate, NK cells require a longer contact period with their targets than CTLs do. This is thought to reflect more complex processing that must occur to integrate activating and inhibitory signals at the NK cell immunological synapse (Fig. 10.9).
Cytoxicity
Cytotoxicity is effected by direct cellular interactions, granule exocytosis, and cytokines
CTLs and NK cells use a variety of different mechanisms to kill their targets. These include:
• direct cell–cell signaling via TNF family molecules;
• pore formation, which allows apoptosis-inducing proteins to access the target cell cytoplasm;
• the extrinsic pathway begins outside the cell. Recognition of pro-apoptotic proteins by specific receptors initiates the caspase cascade;
• the intrinsic pathway is initiated from within the cell. In response to DNA damage or other types of severe cell stress, pro-apoptotic proteins are released from the mitochondria and initiate the caspase cascade.
There are also caspase-independent pathways of apoptosis. All of these may be triggered by CTLs and NK cells. The target cell remains in control of its internal processes throughout apoptosis. Thus CTLs and NK cells effectively instruct their targets to commit suicide (Fig. 10.10).
CTL and NK cell granules contain perforin and granzymes
Activated CTLs and resting CD56low NK cells contain numerous cytoplasmic granules called lytic granules. Upon recognition of a target cell, these granules polarize to the site of contact, the immunological synapse, releasing their contents into a small cleft between the two cells (Fig. 10.11). The lytic granules contain the pore-forming protein perforin, as well as a series of granule-associated enzymes, called granzymes.
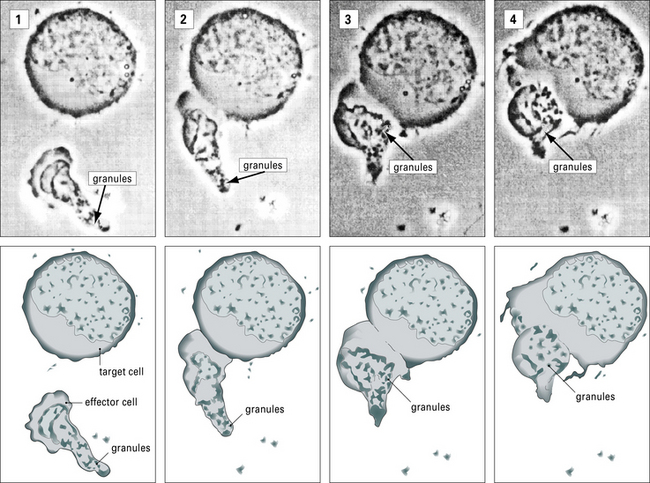
Fig. 10.11 Intracellular reorganizations during effector-target cell interaction
(Courtesy of Dr VH Engelhard.)
Perforin is inactive when located within the granules, but undergoes a conformational activation, which is Ca2+ dependent. Like C9, perforin is able to form homopolymers, inserting into the membrane to form a circular pore of approximately 16 nm diameter. Unlike C9, perforin is able to bind membrane phospholipids directly in the presence of Ca2+ (Fig. 10.12).
• granzyme B cleaves pro-caspases 3, 7, and 8, triggering apoptosis in the target cell. Granzyme B-deficient mice show delayed but not ablated cytotoxicity, illustrating the importance of other pathways;
• granzyme A triggers apoptosis via a caspase-independent pathway. It targets the ER-associated protein complex SET, activating DNAse, which nicks the target cell DNA. It also cleaves nuclear laminins, leading to loss of nuclear structure, and acts in the mitochondria to increase ROI production.
Some cell types are resistant to cell-mediated cytotoxicity
• both perforin and granzymes are synthesized as inactive precursors that need to be activated by cleavage;
• activation takes place only after perforin and granzymes have been released from the granules. Inside granules, pH, Ca2+ levels and the presence of proteoglycans that bind perforin and granzymes are thought to keep them inactive;
• during degranulation, a membrane-bound form of cathepsin B lines the granule membrane and cleaves perforin on the CTL or NK side of the synapse;
• CTL and NK express cFLIP, a protein that inhibits the cleavage of caspase 8 and prevents apoptosis via the caspase 8 pathway;
• they also express protease inhibitor 8 (PI-8), a serpin that can inhibit granzyme B activity.
• neurons, as well as cells in other immune privileged sites, such as the cornea and testes, express FasL. This induces apoptosis in Fas-expressing T and NK cells as they enter the tissue;
• tissue-resident NK cells are generally not efficient killers. In non-pathological situations, neurons, hepatocytes and most placental extravillous trophoblast cells are only exposed to tissue-resident, and not blood NK cells;
• placental villous trophoblast cells express no MHC class I molecules, and are in direct contact with peripheral blood NK cells. They form a syncytium, and this may confer some resistance to killing, but other mechanisms, as yet undefined, are also likely to be important;
• some placental extravillous trophoblast cells are exposed to peripheral blood NK cells. These express the classical MHC class I molecule HLA-C, and the non-classical class I molecules HLA-E and -G, which effectively inhibit NK cell activation.
Non-lymphoid cytotoxic cells
Macrophages and neutrophils primarily kill target cells by phagocytosis
• the production of reactive oxygen species, toxic oxidants, and nitric oxide;
• the secretion of molecules such as neutrophil defensins, lysosomal enzymes and cytostatic proteins (Fig. 10.13).
Eosinophils kill target cells by ADCC
Eosinophil degranulation can be triggered in a number of ways:
• binding to IgG-coated targets via FcγRII;
• binding to IgE-coated targets via FcεRII;
• by exposure to cytokines, including IL-3, IL-5, granulocyte-macrophage colony stimulating factor (GM-CSF), TNF, IFNβ and platelet-activating factor (PAF). The cytokines also enhance ADCC-mediated degranulation.
The components of the eosinophil specific granule include:
• major basic protein (MBP), which is the major component of the granules, forming the crystalloid core. It increases membrane permeability, causing damage to, and sometimes killing, parasites. It can also damage host cells;
• eosinophil peroxidase (EPO), a highly cationic heterodimeric hemoprotein distinct from the myeloperoxidase of neutrophils and macrophages. In the presence of H2O2, which is also produced by eosinophils, EPO will oxidize a variety of substrates, including halide ions and nitric oxide to produce highly toxic oxidant species. These may represent the eosinophil’s most potent killing mechanism for some parasites, but are also toxic to host cells;
• eosinophil cationic protein (ECP), an eosinophil-specific protein that is toxic to many parasites, particularly Schistosoma mansoni schistosomulae. It is a ribonuclease that binds avidly to negatively charged surfaces. It is possible that it forms membrane channels, which allow other mediators access to the target cell cytoplasm. Eosinophils also produce eosinophil-derived neurotoxin (EDN), another ribonuclease but with strong neurotoxic activity. The ribonuclease activity of these proteins is not required for their toxicity.
Critical thinking: Mechanisms of cytotoxicity (see p. 436 for explanations)
Treatment | Tumor line 1 | Tumor line 1 S |
---|---|---|
no effector cells | 1% | 2% |
CTLs | 84% | 2% |
NK cells | 15% | 88% |
anti-class I antibody | 2% | 1% |
CTLs + anti-class I antibody | 6% | 1% |
NK cells + anti-class I antibody | 46% | 85% |
1. Why is target cell DNA fragmentation a good indication of CTL and NK cell activity?
2. From the cultures with CTLs or NK cells alone, what can you deduce about Tumor lines 1 and 1 S?
3. How can you account for the observation that NK cells do cause some DNA fragmentation in Tumor line 1? How would you test your hypothesis?
4. The cultures were repeated including an antibody that recognizes MHC class I molecules, and blocks their interactions. How can you account for the effects on DNA fragmentation observed in Tumor line 1?
Cheent K., Khakoo S.I. Natural killer cells: integrating diversity with function. Immunology. 2009;126:449–457.
Colonna M. Interleukin-22-producing natural killer cell and lymphoid tissue inducer-like cells in mucosal immunity. Immunity. 2009;31:15–23.
Colucci F., Caligiuri M.A., Di Santo J.P. What does it take to make a natural killer? Nat Rev Immunol. 2003;3:413–425.
Cullen S.P., Martin S.J. Mechanisms of granule-dependent killing. Cell Death Differ. 2008;15:251–262.
Di Santo J.P. A defining factor for natural killer cell development. Nat Immunol. 2009;10:1051–1052.
Dustin M.L., Long E.O. Cytotoxic immunological synapses. Immunol Rev. 2010;235:24–34.
Joncker N.T., Raulet D.H. Regulation of NK cell responsiveness to achieve self-tolerance and maximal responses to diseased target cells. Immunol Rev. 2008;224:85–97. 2010
Kärre K. Natural killer cell recognition of missing self. Nat Immunol. 2008;9:477–480.
Lanier L.L. Up on the tightrope: natural killer cell activation and inhibition. Nat Immunol. 2008;9:495–502.
López-Larrea C., Suárez-Alvarez B., López-Soto A., et al. The NKG2D receptor: sensing stressed cells. Trends Mol Med. 2008;14:179–189.
Rothenberg M.E., Hogan S.P. The Eosinophil. Annu Rev Immunol. 2006;24:147–174.
Vivier E., Tomasello E., Baratin M., et al. Functions of natural killer cells. Nat Immunol. 2008;9:503–510.
Weninger W., Manjunath N., von Adrian U.H. Migration and differentiation of CD8+ T cells. Immunol Rev. 2002;186:221–233.