11 Causes of Premature Aging of the Spine
KEY POINTS
Introduction
Premature aging of the spine is a salient concern, in that if it achieves clinical relevance associated with symptoms and impaired function, it has the potential to incur severe socioeconomic consequences. To identify such degenerative changes, advanced imaging, such as magnetic resonance imaging (MRI), has been a popular mainstay in the armamentarium of the physician for diagnostic and therapeutic interventions. However, numerous studies have also documented that the severity of radiological changes is not always associated with clinical symptoms (Figure 11-1).1 Nonetheless, it is essential to determine whether degenerative changes of the spine are a part of the natural evolution of the spine because of age, or if they result from a disease process (Figure 11-2) heralded by risk factors, possibly preventable, that prematurely change the spine. In this chapter, the authors will discuss the numerous factors that may contribute to premature aging of the spine.
Premature Aging Factors
Biochemical
In humans, the notochordal cells in the nucleus pulposus (NP) dramatically decrease after birth, and they eventually disappear, probably through apoptosis, and are replaced by chondrocyte-like cells by the first decade. The reduction in notochordal cell population with maturity could decrease proteoglycan production and contribute to the degenerative process. Furthermore, the naturally occurring cellular senescence, via telomere shortening, plays a role in disc aging as well as degeneration. However, degenerated discs are prone to increased cell senescence as the exposure to various factors, such as interleukin-1 (IL-1), reactive oxygen species, and mechanical load, further accelerate disc degeneration. Therefore exposure to such factors could induce premature senescence of the disc.2
In addition, degenerated discs have been shown to have higher concentrations and activities of degradative enzymes than normal discs, which could be due to the phenotypic changes of disc cells in response to various stimuli such as chemical mediators and mechanical loading. The alteration in disc cell phenotype leads to a cascade of biochemical changes that include the following: (1) decrease in matrix synthesis (e.g., aggrecan, decorin, type II and type IX collagens); (2) downregulation in the expression of growth factors and their receptors, which impairs the regenerative processes; and (3) upregulation of the catabolic metabolism through the increase in concentration and activity of matrix metalloproteinases (MMPs), reduction in tissue inhibitors of metalloproteinases (TIMP) levels, as well as the increase in proinflammatory cytokines and their receptor levels. Among all the cytokines, interleukin-1β in particular seems to play a central role, because it suppresses matrix synthesis and also stimulates the production of other inflammatory mediators, which further enhances matrix catabolism.2,3
Biomechanical
Clinically, disc degeneration is generally more prevalent and severe in the lower lumbar discs, suggesting that higher mechanical loading in this region may be a strong causative factor. Mechanical insults to the disc could induce fatigue failures in the endplates or annulus, which accelerate the catabolic cascade. However, mechanical loading is not a deleterious factor in itself as loading within physiological range stimulates disc matrix turnover and enhances anabolic factors, such as proteoglycan synthesis and TIMP production, whereas loading outside this range (less or more than optimal) is detrimental to disc metabolism. In vivo animal studies showed that high magnitudes or frequencies of dynamic and static compression induced cell apoptosis, structural failure and increased catabolism, whereas downregulation of anabolic gene expressions has been shown in the discs exposed to immobilization.4–7
A cadaveric investigation by Videman et al8 reported that history of occupational physical loading was related to disc degeneration and pathological changes of the lumbar spine, but a clear linear dose-dependent relationship was not established. In former elite athletes, more spinal degenerative findings were presented in those who engaged in heavier loading exercises versus light exercises, yet it only accounted for less than 10% of variability of MRI findings, despite the extreme difference in loading conditions.9 In fact, heavier lifetime physical loading, involving both occupational and leisure time activities, accounted only for small amounts of variance in disc degeneration in MRI, being 7% over T12-L4 and 2% over L4-S1.10 Moreover, when accounting for individual anthropometric parameters, such as body weight, lifting strength, and axial disc area in relation to disc degeneration, although all with modest effect, the lifelong continuous loading associated with these parameters was more influential than extrinsic physical loading related to occupation and leisure activities.11
Atherosclerosis
The blood supply to the lumbar spine is derived from the abdominal aorta, which gives off branches to supply regional vertebral segments (Figure 11-3).12 The nutritional supply to the intervertebral disc (IVD) depends on the diffusion potential through the endplates of the vertebral bodies. Therefore disc nutrition could be impeded by factors that diminish blood flow to the vertebrae, by defects or calcification of the endplates, or a combination of the three. An autopsy study by Kauppila et al.13 determined that the severity of disc degeneration was significantly associated with the grade of stenosis of the segmental arteries supplying the disc, and this association was stronger in the upper three lumbar levels than the lower two levels. Moreover, the degree of disc degeneration also increased in line with the complexity of the atherosclerotic lesions in the abdominal aorta. In accordance to the cadaveric studies by Kauppila et al.13 in vivo MRI studies have confirmed the association between impaired lumbar artery blood flow and diminished disc diffusion in both healthy subjects14 and patients with low back symptoms.15 It is generally thought that the reduced disc diffusion could in turn cause disc degeneration, but no definite answer to this hypothesis is available. Furthermore, clinical low back symptoms were also found to be associated with the presence of lumbar arterial stenosis.16–18 An atherosclerotic contribution to back symptoms is further substantiated in a long-term prospective study by Leino-Arjas et al.19 in which high baseline serum total cholesterol and triglyceride levels were associated with incident radiating low back pain among Finnish industrial employees. The increased risk of abnormal levels of cholesterol and triglycerides on radiating pain was independent of other potential risk factors, such as age, gender, occupational class, work history, exercise habits, smoking, and body mass index (BMI). The mechanism through which atherosclerosis and occlusion of arteries affect disc degeneration may be directly related to diminished blood flow, and hence decreased nutritional supply to the IVD, and also by the systemic inflammatory effect associated with atherosclerosis.
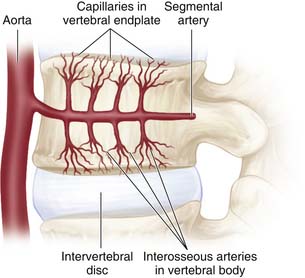
FIGURE 11-3 The segmental artery provides blood supply to the vertebral body.
(Adapted from Raj PP. Intervertebral disc: anatomy-physiology-pathophysiology-treatment. Pain Pract 2008;8:18-44.)
In a long-term follow-up study of 98,407 female nurses, cardiovascular risk factors, such as smoking, diabetes, hypertension, high cholesterol, obesity, and family history of myocardial infarction before the age of 60 years, were significantly associated with an increased risk of lumbar disc herniation. Moreover, after adjustment for other cardiovascular risk factors, the increased risk of symptomatic disc herniation exhibited a dose-dependent relationship with smoking (p = .003) and overweight (p = .01).20 However, in an observational study of 270 Japanese elderly with a mean age of 68.4 years, high-levels of LDL cholesterol was only associated with increased risk of disc degeneration at L4/5 (adjusted odds ratio = 2.65; 95% confidence interval = 1.33-5.52), but not at other levels. In the Japanese study, increased disc degeneration was not associated with their atherosclerosis index or with hyperglycemia.21 However, the presence or severity of atherosclerosis at lumbar arteries was not properly assessed, and therefore the general interpretation of “atherosclerosis” in this study could be problematic. Nevertheless, the cited studies highlight the complexity of cardiovascular risk factors and their role upon disc degeneration.
Lifestyle Factors
Disc degeneration had been previously regarded as an accelerated aging process caused by mechanical insults, such as encountered in physically demanding occupations or sports. However, lifestyle factors, such as smoking and obesity are regarded nowadays as risk factors of low back pain on the basis of meta-analyses.22 In recent decades, a series of exposure-discordant twin studies using MRI to assess the association between disc degeneration and environmental risk factors exposure has been conducted. By comparing the differences of interest between twin siblings in these studies, the effects of genetics and early childhood exposures between subjects were better understood, providing a new perspective regarding disc degeneration.23 On the basis of these twin studies, detrimental lifestyle factors may have negligible effect upon disc degeneration.