Cardiovascular and Pulmonary Anatomy
The heart lies in series with the lungs, constituting the cardiovascular and pulmonary unit, the central component of the oxygen transport pathway.1,2 Virtually all the blood returned to the right side of the heart passes through the lungs and is delivered to the left side of the heart for ejection into the systemic, coronary, and bronchopulmonary circulations. Because of this interrelationship, changes in lung function can exert changes in heart function and vice versa. A detailed understanding of the anatomy of the heart and lungs and how these organs work synergistically is essential to the practice of cardiovascular and pulmonary physical therapy.
This chapter presents the anatomy of the cardiovascular and pulmonary systems, including the skeletal features of the thoracic cavity; muscles of respiration; anatomy of the tracheobronchial tree; lung parenchyma; basic anatomy of the heart; and peripheral, pulmonary, and lymphatic circulations.3–11
Thorax
The bony thorax covers and protects the principal organs of respiration and circulation as well as the liver and the stomach (Fig. 3-1). The anterior surface is formed by the sternum and the costal cartilage. The lateral surfaces are formed by the ribs. The posterior surface is formed by the 12 thoracic vertebrae and the posterior part of the 12 ribs. At birth, the thorax is nearly circular, but during childhood and adolescence it becomes more elliptical. In adulthood, the transverse diameter of the chest wall is greater than the anteroposterior diameter.
Movements of the Thorax
The frequency of movement of the bony thorax joints is greater than that of almost any other combination of joints in the body. Two types of movements have been described: the pump-handle movement and the bucket-handle movement (Fig. 3-2).12 The upper ribs are limited in their ability to move. Each pair swings like a pump handle, with elevation thrusting the sternum forward. This forward movement increases the anteroposterior diameter and the depth of the thorax and is called the pump-handle movement. In the lower ribs, there is little anteroposterior movement. During inspiration, the ribs swing outward and upward, each rib helps to elevate the rib above it. This bucket-handle movement increases the transverse diameter of the thoracic cage. Thus during inspiration, the thorax increases its volume by increasing its anteroposterior and transverse diameters.
Muscles of Respiration
Inspiration
Diaphragm
The diaphragm is a large, dome-shaped muscle that separates the thoracic and abdominal cavities. Its upper surface supports the pericardium (with which it is partially blended), heart, pleurae, and lungs. Its lower surface is almost completely covered by the peritoneum and overlies the liver, kidneys, suprarenal glands, stomach, and spleen (Fig. 3-3). This large muscle can be divided into right and left halves. Each half is made up of three parts: sternal, lumbar, and costal. These three parts insert into the central tendon, which lies just below the heart. The sternal part arises from the back of the xiphoid process and descends to the central tendon. On each side is a small gap, the sternocostal triangle, which is located between the sternal and costal parts. It transmits the superior epigastric vessels and is often the site of diaphragmatic hernias. The costal parts form the right and left domes. They arise from the inner surfaces of the lower four ribs and the lower six costal cartilages, then interdigitate and transverse the abdomen to insert into the anterolateral part of the central tendon, the central part of the diaphragm. The lumbar part arises from the bodies of the upper lumbar vertebrae and extends upward to the central tendon. The central tendon is a thin, strong aponeurosis situated near the center of the muscle, somewhat closer to the front of the body. It resembles a trefoil leaf, with its three divisions or leaflets. The right leaflet is the largest, the middle is the next largest, and the left leaflet is the smallest.
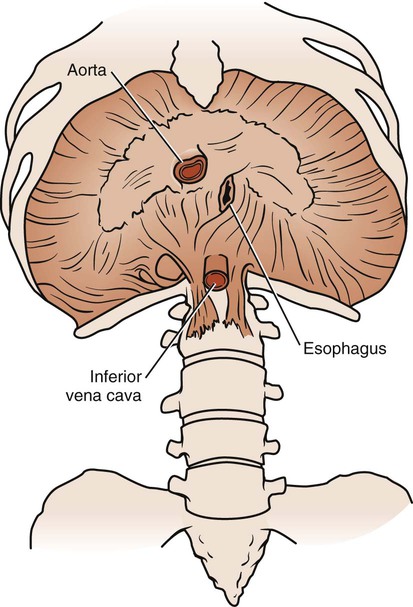
Major vessels traverse the diaphragm through one of three openings (see Fig. 3-3). The vena caval opening is located to the right of the midline in the central tendon and contains branches of the right phrenic nerve and the inferior vena cava. The esophageal opening is located to the left of the midline and contains the esophagus, the vagal nerve trunks, and branches of the gastric vessels. The aortic opening is located in the midline and contains the aorta, the thoracic duct, and sometimes the azygos vein. The diaphragm is also pierced by branches of the left phrenic nerve, small veins, and lymph vessels.
The position of the diaphragm and its range of movement vary with posture, the degree of distention of the stomach, size of the intestines, size of the liver, and obesity. Average movement of the diaphragm in quiet respiration is 12.5 mm on the right and 12 mm on the left. This can increase to a maximum of 30 mm on the right and 28 mm on the left during increased ventilation. An individual’s posture determines the position of the diaphragm. In the supine position, the resting level of the diaphragm rises. The greatest respiratory excursions during normal breathing occur in this position; however, the lung volumes are decreased because of the elevated position of the abdominal organs within the thoracic cavity. In a sitting or upright position, the dome of the diaphragm is pulled down by the abdominal organs, allowing a larger lung volume. For this reason, individuals who are short of breath are more comfortable sitting than reclining. In a side-lying position, the dome of the diaphragm on the lower side rises farther into the thorax than the dome on the upper side (Fig. 3-4). The abdominal organs tend to be displaced forward in a side-lying position, allowing greater excursion of the dome on the lower side. In contrast, the upper side moves little with respiration. On radiograph, the position of the diaphragm can indicate whether the film was taken during inspiration or expiration and may also indicate pathology in the lungs, pleurae, or abdomen.
Contraction of the diaphragm increases the thoracic volume vertically and transversely. The central tendon is drawn down by the diaphragm as it contracts. As the dome descends, abdominal organs are pushed forward as far as the abdominal walls will allow. When the dome can descend no farther, the costal fibers of the diaphragm contract to increase the thoracic diameter of the thorax. This occurs because the fibers of the costal part of the diaphragm run vertically from their attachment at the costal margin. Thus contraction of these fibers elevates and everts the ribs (Fig. 3-5). If the diaphragm is in a low position, it will change the angle of pull of the muscle’s costal fibers. Contraction of these fibers creates a horizontal pull, which causes the lateral diameter to become smaller as the ribs are pulled in toward the central tendon.
As the diaphragm descends, it compresses the abdominal organs, increasing intraabdominal pressure. At the same time, the intrathoracic pressure decreases as the lung volume is increased by the descending diaphragm. Inspiratory airflow occurs as a result of this decrease in intrathoracic pressure (see Chapter 4). The pressure gradient between the abdominal and thoracic cavities also facilitates the return of blood to the right side of the heart.
Intercostals
The external intercostals extend down and forward from the tubercles of the ribs (above) to the costochondral junction of the ribs (below), where they become continuous with the anterior intercostal membrane (Fig. 3-6). This membrane extends the muscle forward to the sternum. There are 11 external intercostal muscles on each side of the sternum. They are thicker posteriorly than anteriorly and thicker than the internal intercostal muscles. They are innervated by the intercostal nerves, and contraction draws the lower rib up and out toward the upper rib. This action increases the volume of the thoracic cavity.
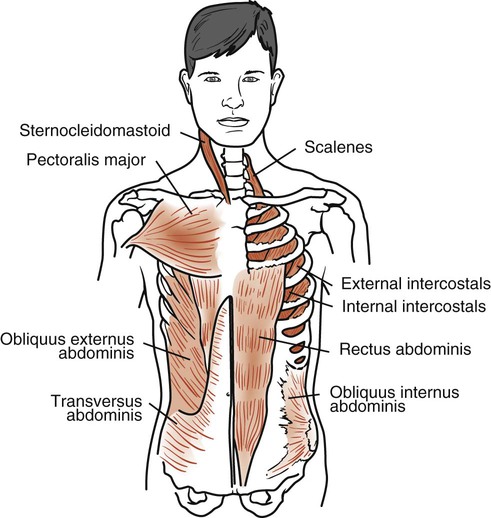
Sternocleidomastoid
The sternocleidomastoid (SCM) muscles are strong neck muscles arising from two heads, one from the manubrium and one from the medial part of the clavicle (see Fig. 3-6). These two heads fuse into one muscle mass that inserts behind the ear into the mastoid process. It is innervated by the accessory nerve and the second cervical nerve. There are two of these muscles, one on each side of the neck. When one SCM contracts, it tilts the head toward the shoulder of the same side and rotates the face toward the opposite shoulder. If the two SCM muscles contract together, they pull the head forward into flexion. When the head is fixed, the muscles assist in elevating the sternum, increasing the anteroposterior diameter of the thorax.
The SCMs are the most important accessory muscles of inspiration. Their contractions can be observed in all patients during forced inspiration and in all patients who are dyspneic. These muscles become visually predominant in patients who are chronically dyspneic (see Chapter 5).
Scalenes
The anterior, medial, and posterior scalenes are three separate muscles that are considered a functional unit. They are attached superiorly to the transverse processes of the lower five cervical vertebrae and inferiorly to the upper surface of the first two ribs (see Fig. 3-6). They are innervated by their corresponding cervical spinal nerves. These muscles are primarily supportive neck muscles, but they can assist in respiration through reverse action. When their superior attachment is fixed, the scalenes act as accessory respiratory muscles and elevate the first two ribs during inspiration.
Pectoralis Major
The pectoralis major is a large muscle arising from the clavicle, the sternum, and the cartilages of all the true ribs (see Fig. 3-6). This muscle spreads across the anterior chest and inserts into the intertubercular sulcus of the humerus. It is innervated by the lateral and medial pectoral nerves and cervical nerves C5, C6, C7, C8, and T1. There are two of these muscles, one on each side of the body. This muscle rotates the humerus medially and draws the arm across the chest. During climbing and pull-ups, it draws the arms toward the trunk. During forced inspiration when the arms are fixed, it draws the ribs toward the arms, thereby increasing thoracic diameter.
Trapezius
The trapezius consists of two muscles that form an extensive diamond-shaped sheet extending from the head down the back and out to both shoulders (Fig. 3-7). Its upper belly originates from the external occipital protuberance, curves around the side of the neck, and inserts into the posterior border of the clavicle. The middle part of the muscle arises from a thin diamond-shaped tendinous sheet, the supraspinous ligaments, and the spines of the upper thoracic region; it then runs horizontally and inserts into the spine of the scapula. Its lower belly arises from the supraspinous ligaments and the spines of the lower thoracic region, runs upward, and inserts into the lower border of the spine of the scapula. This large muscle is innervated by the external or spinal part of the accessory nerve and cervical nerves C3 and C4. Its main function is to rotate the scapulae during arm elevation and control gravitational descent of the arms. It also braces the scapulae and raises them, as when shrugging the shoulders. Its ability to stabilize the scapulae makes it an important accessory muscle in respiration. This stabilization enables the serratus anterior and pectoralis minor to elevate the ribs.
Erector Spinae
The erector spinae is a large muscle extending from the sacrum to the skull (see Figure 3-7). It originates from the sacrum, the iliac crest, and the spines of the lower thoracic and lumbar vertebrae. It separates into a lateral iliocostalis, an intermediate longissimus, and a medial spinalis column. This muscle mass inserts into various ribs and vertebral processes all the way up to the skull. It is innervated by the corresponding spinal nerves. These muscles extend, laterally flex, and rotate the vertebral column. They are considered accessory respiratory muscles through their extension of the vertebral column. In deep inspiration, these muscles extend the vertebral column, allowing further elevation of the ribs.
Expiration
Rectus Abdominis
The rectus abdominis rises from the pubic crest, extends upward, and inserts into the xiphoid process and the costal margin of the fifth, sixth, and seventh costal cartilages (see Fig. 3-6). It is innervated by corresponding spinal nerves and its action is considered within the context of the other abdominal muscles.
Obliquus Externus Abdominis
The obliquus externus abdominis arises in an oblique line from the fifth costal cartilage to the 12th rib (see Fig. 3-6). Its posterior fibers attach in an almost vertical line with the iliac crest. The other fibers extend down and forward and attach to the front of the xiphoid process, the linea alba, and the pubic symphysis. It is innervated by the lower six thoracic spinal nerves.
Obliquus Internus Abdominis
The obliquus internus abdominis originates from the lumbar fascia, the anterior two-thirds of the iliac crest, and the lateral two-thirds of the inguinal ligament (see Fig. 3-6). Its posterior fibers run almost vertically upward and insert into the lower borders of the last three ribs. The other fibers join an aponeurosis attached to the costal margin above, the linea alba in the midline, and the pubic crest below. It is innervated by the lower six thoracic nerves and the first lumbar spinal nerves.
Transversus Abdominis
The transversus abdominis arises from the inner surface of the lower six costal cartilages, the lumbar fascia, the anterior two-thirds of the iliac crest, and the lateral one-third of the inguinal ligament (see Fig. 3-6). It runs across the abdomen horizontally and inserts into the aponeurosis, extending to the linea alba. It is innervated by the lower six thoracic nerves and the first lumbar spinal nerves.
Action of the Abdominal Muscles
The four muscles of the abdomen work together to provide a firm but flexible wall to keep the abdominal viscera in position. The abdominal muscles exert a compressing force on the abdomen when the thorax and pelvis are fixed. This force can be used in defecation, urination, parturition, and vomiting. In forced expiration, the abdominal muscles help force the diaphragm back to its resting position and thus force air from the lungs. If the pelvis and vertebral column are fixed, the obliquus externus abdominis aids expiration further by depressing and compressing the lower part of the thorax. Patients with chronic obstructive pulmonary disease (COPD) have difficulty in exhalation, which causes them to trap air in their lungs. The continued contraction of the abdominal muscles throughout exhalation helps them force this air from the lungs. The abdominal muscles also play an important role in coughing. First, a large volume of air is inhaled, and the glottis is closed. The abdominal muscles then contract, raising intrathoracic pressure. When the glottis opens, the large difference in intrathoracic and atmospheric pressure causes the air to be expelled forcefully at tremendous flow rates (tussive blast). Individuals with weak abdominal muscles (from neuromuscular diseases, paraplegia, quadriplegia, or extensive abdominal surgery) often have ineffective coughs (see Chapters 6 and 32).