28 Cardiopulmonary Bypass Management and Organ Protection
An anesthesiologist-in-training posed the question, “Why is my presence necessary during cardiopulmonary bypass [CPB]? The perfusionist has direct control of the patient’s blood pressure and respiration. The inhalation anesthetic is attached to the bypass circuit. Drugs are administered into the venous reservoir. What is my role?”1 The resident’s own answer was incomplete but more robust than that argued by many clinicians, who suggest that the presence of a member of the anesthesia care team (e.g., anesthesiologist, nurse anesthetist, credentialed anesthesia assistant) during CPB is not essential. The American Society of Anesthesiologists (ASA) states that the absence of anesthesia personnel during the conduct of a general anesthetic violates the first of the ASA Standards for Basic Anesthesia Monitoring.2 The absence of a member of the anesthesia care team during CPB is below the accepted standard of care. Moreover, to bill for anesthesia care when an anesthesia provider is not physically present in the patient’s operating room constitutes fraud.3 At a minimum, the anesthesiologist’s role during CPB is to maintain the anesthetic state—a more challenging task than the usual case when the patient’s blood pressure, heart rate, and movement provide information regarding the depth of anesthesia. The complexities of CPB and the necessary integration of risk factors with the nuances of cardiac surgery warrant the constant thinking and rethinking of how the conduct of CPB and surgery modulates the risk and what protective strategies need implementation. This chapter outlines the tasks, challenges, and responsibilities of the cardiovascular anesthesiologist that extend beyond the maintenance of the anesthetic state, focusing on overall organ protection.
Historic perspective on cardiopulmonary bypass
On May 6, 1953, John Gibbon, Jr., surgically treated a young woman using CPB, and the long-elusive goal of extracorporeal circulation (ECC) was achieved (Figure 28-1). This accomplishment was preceded by 15 years of work by Gibbon and colleagues to develop a device that would provide ECC and support respiration. The 50th anniversary of the first successful use of CPB was celebrated in 2003. A number of insightful perspectives on this important medical landmark accompanied the anniversary of this achievement.4–7
This chapter briefly describes modern bypass circuits and highlights the many current controversies regarding the management of patients during CPB. It also deals with perfusion accidents that can be life-threatening events. It is critical that all members of the cardiac surgery team anticipate and respond appropriately to mishaps during CPB (see Chapter 29). More common than the rare catastrophe that can occur are the injurious end-organ effects that can result from the inherently physiologic nature of CPB. The multiple pathophysiologic perturbations precipitated by the process of ECC and the putative effects of these phenomena on end-organ function are discussed in detail.
Goals and mechanics of cardiopulmonary bypass
Venous return may be decreased deliberately (as is done when restoring the patient’s blood volume before coming off bypass) by application of a venous clamp. From the reservoir, blood is pumped to an oxygenator and heat exchanger unit before passing through an arterial filter and returning to the patient. Additional components of the circuit generally include pumps and tubing for cardiotomy suction, venting, and cardioplegia delivery and recirculation, as well as in-line blood gas monitors, bubble detectors, pressure monitors, and blood sampling ports. A schematic representation of a typical bypass circuit is depicted in Figure 28-2 (see Chapter 29).
The cannulation sites and type of CPB circuit used are dependent on the type of operation planned.8 Most cardiac procedures use full CPB, in which the blood is drained from the right side of the heart and returned to the systemic circulation through the aorta. The CPB circuit performs the function of the heart and lungs. Aorto-atriocaval cannulation is the preferred method of cannulation for CPB, although femoral arteriovenous cannulation may be the technique of choice for emergency access, “redo” sternotomy, and other clinical settings in which aortic or atrial cannulation is not feasible. Procedures involving the thoracic aorta often are performed using partial bypass in which a portion of oxygenated blood is removed from the left side of the heart and returned to the femoral artery. Perfusion of the head and upper extremity vessels is performed by the beating heart, and distal perfusion is provided below the level of the cross-clamp by retrograde flow by the femoral artery. All blood passes through the pulmonary circulation, eliminating the need for an oxygenator.
Central nervous system injury

Incidence and Significance of Injury
CNS dysfunction after CPB represents deficits ranging from neurocognitive deficits, occurring in approximately 25% to 80% of patients, to overt stroke, occurring in 1% to 5% of patients.9–12 The significant disparity between studies in the incidence of these adverse cerebral outcomes relates, in part, to their definition and to numerous methodologic differences in the determination of neurologic and neurocognitive outcome. Retrospective versus prospective assessments of neurologic deficits account for a significant portion of this inconsistency, as do the experience and expertise of the examiner. The timing of postoperative testing also affects determinations of outcome. For example, the rate of cognitive deficits can be as high as 80% for patients at discharge, between 10% and 35% at approximately 6 weeks after CABG, and 10% to 15% more than a year after surgery. Greater rates of cognitive deficits have been reported 5 years after surgery, when up to 43% of patients have documented deficits.10 The issue of whether cardiac surgery causes cognitive loss has been greatly debated. Although some have questioned whether long-term deficits result as a consequence of surgery,13 even if only present in the short term, they are meaningful to patients and families14 (see Chapter 36).
Although the incidence of these deficits varies greatly, the significance of these injuries cannot be overemphasized. Cerebral injury is a most disturbing outcome of cardiac surgery. To have a patient’s heart successfully treated by the planned operation but discover that the patient no longer functions as well cognitively or is immobilized from a stroke can be devastating. There are enormous personal, family, and financial consequences of extending a patient’s life with surgery, only to have the quality of the life significantly diminished.12,15 Mortality after CABG, although having reached relatively low levels in recent years (generally < 1% overall), increasingly is attributable to cerebral injury.12

Risk Factors for Central Nervous System Injury
Successful strategies for perioperative cerebral and other organ protection begin with a thorough understanding of the risk factors, causative factors, and pathophysiology involved. Risk factors for CNS injury can be considered from several different perspectives. Most studies outlining risk factors take into account only stroke. Few describe risk factors for neurocognitive dysfunction. Although it often is assumed that their respective risk factors are similar, few studies consistently have reported the preoperative risks for cognitive loss after cardiac surgery. Factors such as a poor baseline (preoperative) cognitive state, years of education (i.e., more advanced education is protective), age, diabetes, and CPB time frequently are described.16,17
Stroke is better characterized with respect to risk factors. Although studies differ somewhat as to all the risk factors, certain patient characteristics consistently correlate with an increased risk for cardiac surgery–associated neurologic injury. In a study of 2108 patients from 24 centers conducted by the Multicenter Study of Perioperative Ischemia, incidence of adverse cerebral outcome after CABG surgery was determined and the risk factors analyzed. Two types of adverse cerebral outcomes were defined. Type I included nonfatal stroke, transient ischemic attack, stupor or coma at time of discharge, and death caused by stroke or hypoxic encephalopathy. Type II included new deterioration in intellectual function, confusion, agitation, disorientation, and memory deficit without evidence of focal injury. A total of 129 (6.1%) of the 2108 patients had an adverse cerebral outcome in the perioperative period. Type I outcomes occurred in 66 (3.1%) of 2108 patients, with type II outcomes occurring in 63 (3.0%) of 2108 patients. Stepwise logistic regression analysis identified eight independent predictors of type I outcomes and seven independent predictors of type II outcomes (Table 28-1).
TABLE 28-1 Risk Factors for Adverse Cerebral Outcomes after Cardiac Surgery
Risk Factor | Type I Outcomes* | Type II Outcomes* |
---|---|---|
Proximal aortic atherosclerosis | 4.52 (2.52–8.09) | |
History of neurologic disease | 3.19 (1.65–6.15) | |
Use of IABP | 2.60 (1.21–5.58) | |
Diabetes mellitus | 2.59 (1.46–4.60) | |
History of hypertension | 2.31 (1.20–4.47) | |
History of pulmonary disease | 2.09 (1.14–3.85) | 2.37 (1.34–4.18) |
History of unstable angina | 1.83 (1.03–3.27) | |
Age (per additional decade) | 1.75 (1.27–2.43) | 2.20 (1.60–3.02) |
Admission systolic BP > 180 mm Hg | 3.47 (1.41–8.55) | |
History of excessive alcohol intake | 2.64 (1.27–5.47) | |
History of CABG | 2.18 (1.14–4.17) | |
Arrhythmia on day of surgery | 1.97 (1.12–3.46) | |
Antihypertensive therapy | 1.78 (1.02–3.10) |
BP, blood pressure; CABG, coronary artery bypass graft surgery; IABP, intra-aortic balloon pump.
* Adjusted odds ratio (95% confidence intervals) for type I and II cerebral outcomes associated with selected risk factors from the Multicenter Study of Perioperative Ischemia.
From Arrowsmith JE, Grocott HP, Reves JG, et al: Central nervous system complications of cardiac surgery. Br J Anaesth 84:378, 2000.
In a subsequent analysis of the same study database, a stroke risk index using preoperative factors was developed (Figure 28-3). This risk index allowed for the preoperative calculation of the stroke risk based on the weighted combination of the preoperative factors, including age, unstable angina, diabetes mellitus, neurologic disease, prior coronary artery or other cardiac surgery, vascular disease, and pulmonary disease.18 Of all the factors in the Multicenter Study of Perioperative Ischemia analysis and in multiple other analyses,12,19–22 age appeared to be the most overwhelmingly robust predictor of stroke and of neurocognitive dysfunction after cardiac surgery.9,10 Tuman et al22 demonstrated that age has a greater impact on neurologic outcome than it does on perioperative myocardial infarction or low cardiac output states after cardiac surgery (Figure 28-4).
The influence of sex on adverse perioperative cerebral outcomes after cardiac surgery has been evaluated. Women appear to be at greater risk for stroke after cardiac surgery than men.23 Hogue et al24 found that women appear more likely to suffer deficits in the visuospatial cognitive domain after cardiac surgery, although the frequency of cognitive dysfunction after cardiac surgery is similar for women and men.
Other consistent risk factors for stroke after cardiac surgery are the presence of cerebrovascular disease and atheromatous disease of the aorta. With respect to cerebrovascular disease, patients who have had a prior stroke or transient ischemic attack are more likely to suffer a perioperative stroke.23,25–27 Even in the absence of symptomatic cerebrovascular disease, such as the presence of a carotid bruit, the risk for stroke increases with the severity of the carotid artery disease. Breslau et al28 reported that Doppler-detected carotid disease increased the risk for stroke after cardiac surgery by threefold. Similarly, Brener et al29 found that a carotid stenosis greater than 50% increased the risk for stroke from 1.9% to 6.3%.
Although the presence of cerebrovascular disease is a risk factor for perioperative stroke, it does not always correlate well with the presence of significant aortic atherosclerosis.30 Atheromatous disease of the ascending, arch, and descending thoracic aorta has been consistently implicated as a risk factor for stroke in cardiac surgical patients.31–34 The increased use of transesophageal echocardiography (TEE) and epiaortic ultrasonography has added new dimensions to the detection of aortic atheromatous disease and the understanding of its relation to stroke risk. These imaging modalities have allowed the diagnosis of atheromatous disease to be made in a more sensitive and detailed manner, contributing greatly to the information regarding potential stroke risk. The risk for cerebral embolism from aortic atheroma was described early in the history of cardiac surgery,35 and has been described repeatedly in detail since then.12,36–38 For example, Katz et al39 found that the incidence rate of stroke was 25% in patients with a mobile atheromatous plaque in the aortic arch, compared with a stroke rate of 2% in those with limited atheromatous disease. Studies consistently have reported greater stroke rates for patients with increasing atheromatous aortic involvement (particularly the ascending and arch segments).40 This relation is outlined in Figure 28-5.
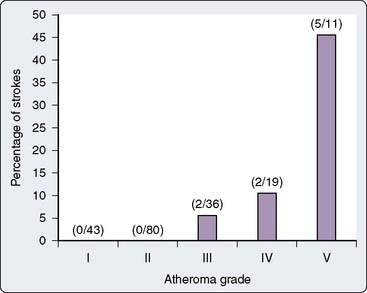
Figure 28-5 Stroke rate 1 week after cardiac surgery as a function of atheroma severity.
(From Hartman GS, Yao FS, Bruefach M 3rd, et al: Severity of aortic atheromatous disease diagnosed by transesophageal echocardiography predicts stroke and other outcomes associated with coronary artery surgery: A prospective study. Anesth Analg 83:701, 1996.)

Cause of Perioperative Central Nervous System Injury
Because CNS dysfunction represents a wide range of injuries, differentiating the individual causes of these various types of injuries becomes somewhat difficult (Box 28-1). They frequently are grouped together and superficially discussed as representing different severities on a continuum of brain injury. This likely misrepresents the different causes of these injuries. The following section addresses stroke and cognitive injury (Table 28-2), and their respective causes are differentiated where appropriate.
TABLE 28-2 Causes of Cognitive Dysfunction after Cardiac Surgery
Cause | Possible Settings |
---|---|
Cerebral microemboli | Generated during cardiopulmonary bypass (CPB); mobilization of atheromatous material or entrainment of air from the operative field; gas injections into the venous reservoir of the CPB apparatus |
Global cerebral hypoperfusion | Hypotension, occlusion by an atheromatous embolus leading to stroke |
Inflammation (systemic and cerebral) | Injurious effects of CPB, such as blood interacting with the foreign surfaces of pump-oxygenator; upregulation of proinflammatory mediators |
Cerebral hyperthermia | Hyperthermia during and after cardiac surgery, such as aggressive rewarming, from hypothermic CPB |
Cerebral edema | Edema from global cerebral hypoperfusion or from hyponatremia; increased cerebral venous pressure from cannula misplacement |
Blood-brain barrier dysfunction | Diffuse cerebral inflammation; ischemia from cerebral microembolization or increased intracranial pressure |
Pharmacologic influences | Anesthetic-related cognitive damage; apoptosis of neonatal brains; proteomic changes |
Genetic influences | Effects of single nucleotide polymorphisms on risk for Alzheimer disease or for acute coronary syndromes and other inflammatory disorders |
Cerebral Embolization
Macroemboli (e.g., atheromatous plaque) and microemboli (e.g., gaseous and particulate) are generated during CPB, and many emboli find their way to the cerebral vasculature.41 Macroemboli are responsible for stroke, with microemboli being implicated in the development of less severe encephalopathies. Sources for the microemboli are numerous and include those generated de novo from the interactions of blood within the CPB apparatus (e.g., platelet-fibrin aggregates) and those generated within the body by the production and mobilization of atheromatous material or entrainment of air from the operative field. Other sources for emboli include lipid-laden debris that can be added by cardiotomy suction.42 Other gaseous emboli may be generated through injections into the venous reservoir of the CPB apparatus itself.43,44
Numerous studies outline the relation between emboli and cognitive decline after cardiac surgery.45–47 However, one of the major limitations in understanding this relation has been the relative inability to discern between gaseous and particulate microemboli.48 Typically, Doppler ultrasonography has been used to measure cerebral embolic signals, but Doppler cannot reliably distinguish between gaseous and particulate emboli.49 In addition to using Doppler evidence, Moody et al41 performed histologic analyses on brains from cardiac surgical patients and described the presence of millions of cerebral emboli represented as small capillary arteriolar dilations.
The impact of aortic atheroma on cognitive decline is incompletely understood. It is widely known from nonsurgical and cardiac surgical studies that there is a clear relation between aortic atheroma and stroke,31,50–52 but the relation between cognitive outcome and cerebral atheroma is uncertain. Several studies describe different results.53,54 Whereas some data suggest that cerebral emboli are more likely with a greater degree of atheroma in the ascending aorta,55 there is a relative failure to demonstrate that these atheroma correspond to cognitive decline.53 Part of the discordance between these two findings may reflect the limitation of Doppler technology to discriminate between gaseous and particulate emboli, thereby possibly misrepresenting the true cerebral embolic load.56
Global Cerebral Hypoperfusion
The concept that global cerebral hypoperfusion during CPB may lead to neurologic and neurocognitive complications originates from the earliest days of cardiac surgery, when significant (in degree and duration) systemic hypotension was a relatively common event. Although making intuitive sense (i.e., that hypotension would lead to global cerebral hypoperfusion), studies that have examined the relation between mean arterial pressure (MAP) and cognitive decline after cardiac surgery generally have failed to show any significant relation.17,57,58
This is not the case for stroke, for which Hartman et al38 and Gold et al59 demonstrated a link between hypotension and the presence of a significantly atheromatous aorta with an increased risk for stroke (see Figure 28-5). This is not a clear relation, however, and likely represents an interaction between macroembolism and global cerebral hypoperfusion. It is likely, for example, that if an area of the brain that is being perfused by a cerebral vessel becomes occluded by an atheromatous embolus, it may be more susceptible to hypoperfusion if collateral perfusion is compromised by concomitant systemic hypotension.60 Other evidence for global cerebral hypoperfusion comes from Mutch et al,61 who examined magnetic resonance imaging assessments of cerebral blood flow (CBF) showing progressive decreases in CBF during the course of experimental CPB in pigs. However, clinical demonstrations of a reduction in CBF during lengthy CPB have not been seen.62
Temperature-Related Factors
The impact of CPB temperature (i.e., hypothermia) on outcome is addressed further in this section. However, with the various trials of hypothermia during CPB and with detailed temperature monitoring, the observation has been made that hyperthermia can occur during certain periods during and after cardiac surgery. During rewarming from hypothermic CPB, there can be an overshoot in cerebral temperature because of aggressive rewarming generally aimed at decreasing time on CPB and overall operating room time. This cerebral hyperthermia may well be responsible for some of the injury that occurs in the brain.63
The postoperative period is also a critical time in which hyperthermia can contribute to brain injury.64,65 Grocott et al64 demonstrated that the peak temperature in the postoperative period (24 hours after surgery) was related to cognitive decline 6 weeks after cardiac surgery. It is not clear whether this hyperthermia causes de novo injury or whether it exacerbates injury that already has occurred (e.g., injury that might be induced by cerebral microembolization or global cerebral hypoperfusion). It is necessary to be cautious in concluding whether these relations are temporal or causal. However, it is assumed that the brain is injured during CPB, and because experimental brain injury is known to cause hyperthermia (resulting from hypothalamic injury66), the hyperthermia that is demonstrated in the postoperative period may be caused by the occurrence or extent of brain injury. However, if hyperthermia results from the inflammatory response to CPB, the hyperthermia itself may induce or exacerbate cerebral injury.
Inflammation
Although it is well-known that blood interacts with the foreign surfaces of the pump-oxygenator to stimulate a profound inflammatory response,67 the systemic end-organ effects of this inflammatory response are less clearly defined. Much of the data relating organ dysfunction in the CNS to the inflammatory response in the cardiac surgical patient have focused on indirect experimental and clinical evidence. It is not clear whether a cerebral inflammatory response occurs as a result of CPB in humans. Hindman et al68 reported that cyclooxygenase mRNA was upregulated after CPB, suggesting that on the molecular biologic level, CPB induces overexpression of this proinflammatory gene in the brain. What is not clear was whether this was a primary event (i.e., as a direct result of the proinflammatory effects of CPB) or a secondary event as a result of other injurious effects of CPB (e.g., microembolization). In settings other than cardiac surgery, inflammation has been demonstrated to directly injure the brain (e.g., sepsis-mediated encephalopathy),69 but it also is known to result as a response to various cerebral injuries (e.g., ischemic stroke).70
There is no direct evidence that inflammation causes cardiac surgery–associated adverse cerebral outcome; however, there is some supportive indirect evidence. For example, Mathew et al71 demonstrated a relation between poor cognitive outcome and an impaired immune response to circulating endotoxin, which inevitably translocates from the gut into the bloodstream because of alterations in splanchnic blood flow during CPB. Having a low antibody response to circulating endotoxin is paradoxically associated with an overstimulated inflammatory response,72 thus demonstrating that the relation between low endotoxin antibodies and poor cognitive outcome may be mediated by an augmented inflammatory response. Increasingly, there is genetic evidence linking inflammation to adverse cerebral outcomes, both stroke and cognitive loss (see Genetic Influences section later in this chapter).
Cerebral Edema
Cerebral edema after CPB has been reported in several studies.73,74 The explanation for why cerebral edema may occur early in the postbypass period is not clear. It may be caused by cytotoxic edema resulting from global cerebral hypoperfusion or possibly by hyponatremia-induced cerebral edema. Generalized cerebral edema caused by increases in cerebral venous pressure caused by cannula misplacement, which frequently occurs during CPB, is another reason.75 Specifically, use of a dual-stage venous cannula often can lead to cerebral venous congestion during the vertical displacement of the heart during access to the lateral and posterior epicardial coronary arteries. It is not clear from these studies whether the edema results because of injury that occurs during CPB, leading to cognitive decline, or whether the edema itself directly causes the injury by consequent increases in intracranial pressure with global or regional decreases in CBF and resulting ischemia.
Blood-Brain Barrier Dysfunction
The function of the blood-brain barrier (BBB) is to aid in maintaining the homeostasis of the extracellular cerebral milieu protecting the brain against fluctuations in various ion concentrations, neurotransmitters, and growth factors that are present in the serum.76 The impact of CPB on the function and integrity of the BBB is not clearly known. Gillinov et al77 were unable to show any changes in BBB dysfunction 2 hours after CPB in piglets as assessed using carbon 14-aminoisobutyric acid tracer techniques in postbypass brain homogenates. However, Cavaglia et al,78 measuring the leakage of fluorescent albumin from blood vessels in brain slices after CPB, were able to demonstrate significant breaches in the BBB. Both studies looked at a single time point (i.e., immediately after CPB), and it is not known whether there are temporal changes in the BBB integrity.
It is difficult to determine whether the changes in BBB integrity, if present at all, are a primary cause of brain dysfunction or simply a result of other initiating events such as ischemia (i.e., from cerebral microembolization) or a diffuse cerebral inflammatory event. Changes in the BBB could cause some of the cerebral edema that has been demonstrated, or it could result from cerebral edema if the edema resulted in ischemic injury (from increases in intracranial pressure).74
Possible Pharmacologic Influences
Anesthetics have been demonstrated to affect cognitive loss after surgery. Experimental studies of cognitive outcome in young rats exposed to anesthetics have demonstrated that relatively brief (several hours) exposure to isoflurane can lead to long-term cognitive changes in the animals.79,80 Coupled with the demonstration in other experimental models of apoptosis in neonatal brains exposed to certain anesthetic agents (e.g., isoflurane, midazolam, nitrous oxide),81 this added to the data suggesting that corresponding proteomic changes can occur in the brain after exposure to anesthetics82 and highlighted this as a potential area for further research.
Genetic Influences
Genetics may play a role in modifying the degree of CNS injury or in the ability of the brain to recover after an injury has occurred. Several investigations have assessed the genetic influences on cerebral outcome after CPB. The most commonly explored gene variant, or single nucleotide polymorphism (SNP), has been the ε4 allele of the apolipoprotein gene. This gene has been reported to be responsible for increasing the risk for sporadic and late-onset Alzheimer disease (as well as complicating outcome after a variety of other head injuries).83 Although early reports suggest that this may be an important influence,84 later reports shed some doubt on how robust this effect is.85 A second SNP examined relates to the platelet surface receptor glycoprotein IIb/IIIa P1A2 (P1A2) receptor polymorphism. This platelet integrin receptor polymorphism is important in the cause of acute coronary syndromes and other thrombotic disorders.86,87 A small study in cardiac surgery patients demonstrated worse impairments in the Mini-Mental Status Examination in the P1A2-positive patients compared with P1A2-negative patients.88
With the multitude of genes that may play a role in injury, it is important to go beyond examining the impact of single SNPs and explore the impact of multiple SNPs, alone or in combination. In a study of 2140 patients examining 26 different SNPs, the presence of the minor alleles of C-reactive protein (CRP), interleukin-6 (IL-6) had a threefold increase in the risk for stroke after cardiac surgery.89 Of note, no single (or combination of) prothrombotic genes were associated with stroke, suggesting that inflammatory, as opposed to thrombotic, mechanisms may be more important to the risk for a stroke.
With respect to cognitive dysfunction after cardiac surgery, a recent study outlined the impact of genetics on outcome after cardiac surgery. In a study of 513 patients who were extensively genotyped (30 SNPs) and had cognitive testing after cardiac surgery, a link between SNPs of CRP and P-selectin (CRP1059G4/C and SELP1087G/A), and a reduction in cognitive deficit were found.90 The incidence rate of cognitive deficit was 16.7% in carriers of the minor alleles of both these genes compared with 42.9% of the patients possessing these major alleles. Unique in this study was the mechanism-based genetic effect in which these polymorphisms also were associated with reductions in both CRP and platelet activation, suggesting that an attenuation of perioperative inflammatory and prothrombotic states may be beneficial with respect to reducing the cognitive deficits after cardiac surgery.91

Neuroprotective Strategies
Emboli Reduction
There are multiple sources of particulate and gaseous emboli during cardiac surgery. Within the CPB circuit itself, particulate emboli in the form of platelet-fibrin aggregates and other debris are generated. Gaseous emboli can be created in the circuit or augmented, if already present, by factors such as turbulence-related cavitation and, potentially, even by vacuum-assisted venous drainage.92 Air in the venous return tubing is variably handled by the bypass circuit (i.e., reservoir, oxygenator, and arterial filters). The ability of the circuit to prevent the transit of gaseous emboli through the oxygenator varies considerably between manufacturers and remains a significant source of emboli. The impact of perfusionist interventions on cerebral embolic load also has been studied. Borger et al44 found that after drug injections into the venous reservoir, gaseous emboli can make a rapid passage through to the arterial outflow. Reducing these perfusionist interventions reduced emboli generation and neurocognitive impairment.
Significant quantities of air can be entrained from the surgical field into the heart itself; flooding the field with carbon dioxide has been proposed as being effective in reducing this embolic source.93 Its ability to specifically reduce cerebral injury has not been rigorously evaluated, although it has been demonstrated to significantly reduce the number of TEE-detectable bubbles in the heart after cardiac surgery.94 Even with the use of carbon dioxide in the surgical field, significant amounts of entrained air can be present. Although the oxygenator-venous reservoir design attempts to purge this air before reaching the inflow cannula, the arterial line filter handles a great deal of what is left. The capacity of the arterial filter to remove all sources of emboli (gaseous or particulate) has significant limitations, and despite its use, emboli can pass easily through and on into the aortic root.
The aortic cannula may be very important to reduce cerebral emboli production. Placement of the cannula into an area of the aorta with a large atheroma burden may cause the direct generation of emboli from the “sandblasting” of atherosclerotic material in the aorta.95 The use of a long aortic cannula, where the tip of the cannula lies beyond the origin of the cerebral vessels, also has been found to reduce emboli load.96 The type of cannula itself may be an important factor. Various designs have allowed the reduction of sandblasting-type jets emanating from the aortic cannula. Baffled cannulae and cannulae that allow the incorporation of regional brain hypothermia and diversion of emboli away from the cerebral vessels have been investigated.97 A cannula that has a basket-like extension that can be inserted just before cross-clamp removal also has been studied.98 In a large (N = 1289) study, this Embol-X cannula was unable to reduce the incidence of CNS dysfunction.99 A smaller (N = 24) study paradoxically showed an increase in embolic signals with its deployment in the aorta.100 This has been because of air bubbles trapped within the basket or abrasion of the atheromatous aortic wall. Few other emboli-reducing strategies, besides arterial line filtration and reducing perfusion interventions itself,44,47 have been studied sufficiently to determine their impact on cognitive loss after cardiac surgery. The safety of introducing new techniques also has not been thoroughly studied; the additional risk assumed when significantly altering a standard of practice to use a new device must be considered (see Chapters 3, 29, and 39).
Blood that is returned from the surgical field through the use of the cardiotomy suction may significantly contribute to the particulate load in the CPB circuit and, subsequently, in the brain. The use of cell-salvage devices to process shed blood before returning it to the venous reservoir may minimize the amount of particulate- or lipid-laden material that contributes to embolization.42,43 Most of this material is small enough or so significantly deformable (due to its high lipid content) that it can pass through standard 40-μm arterial filters. There are several issues with the cell saver, however. One is the cost that is incurred with its use, and the other is its side effects of reducing platelet and coagulation factors through its intrinsic washing processes. Modest use of cell salvage up to a certain, although as yet undefined, volume of blood likely is prudent. Despite this rationale, the results from studies examining neurologic outcome have shown variable effects of cell-saver use on cognitive outcome. A study by Djaiani et al102 demonstrated a benefit, whereas one by Rubens et al101 did not. This may have been caused by differences in cell savers used that likely varied in their ability to remove lipid emboli (see Chapter 29).
Management of Aortic Atherosclerosis
Although the previous section dealt with issues related to reduction of emboli, many of which likely are spawned from atheromatous plaque in the aorta, further specific management of the atheromatous aorta, particularly as it relates to stroke risk, requires special attention. The widespread use of TEE and complementary (and preferably routine) epiaortic scanning has had a tremendous impact on the understanding of the risks involved in the patient with a severely atheromatous aorta. There is indisputable evidence linking stroke to atheroma.31,50–52 However, the strength of association between atheroma and cognitive decline seen after cardiac surgery is less clear.
A small study used a combination of epiaortic scanning and atheroma avoidance techniques (with respect to cannulation, clamping, and vein graft anastomosis placement) to attempt to reduce neurocognitive deficits.54 In that study, the incidence of cognitive decline was lower in patients who had an avoidance technique guided by epiaortic scanning compared with no epiaortic scanning. It was limited by its small size, but it identified an area that requires more investigation. Others have examined this issue and found the relation between cognitive decline and atheroma to be doubtful.103 Regardless of whether atheroma cause cognitive dysfunction, their contribution to cardiac surgery–associated stroke is enough to warrant specific strategies for their management.
One of the difficulties in interpreting studies that have evaluated atheroma avoidance strategies is the absence of any form of blinding of the investigators. For the most part, a strategy is chosen based on the presence of known atheroma, and the results of these patients are compared with historic controls. What constitutes the best strategy is unclear. Multiple techniques can be used to minimize atheromatous material liberated from the aortic wall from getting into the cerebral circulation. These range from optimizing placement of the aortic cannula in the aorta up to an area relatively devoid of plaque to the use of specialized cannulae that reduce the sandblasting of the aortic wall. Alternative aortic cannulae and using different locations possess the ability to decrease embolization of atheromatous plaque. The avoidance of partial occlusion clamping for proximal vein graft placement by performing all of the anastomoses made in a single application of an aortic cross-clamp has been demonstrated to have benefit.104 Specialized cannulae that contain filtering technologies98 and other means to deflect emboli to more distal sites have been developed and are being studied.105 Technology is advancing rapidly, and proximal (and distal) coronary artery anastomotic devices are becoming increasingly available and focus on minimizing manipulation of the ascending aorta. None of these aortic manipulations has yet yielded significant neuroprotective results in large, prospective, randomized trials, but the potential holds promise.
Pulsatile Perfusion
A large volume of literature has accumulated comparing the physiology of pulsatile with nonpulsatile perfusion.106,107 Nevertheless, it remains uncertain whether pulsatile CPB has shown substantive clinical improvement in any outcome measure compared with standard, nonpulsatile CPB. Table 28-3, although by no means complete, represents this highly contradictory body of literature.108–137 Claims of advantages to pulsatile flow are effectively offset by conflicting studies of similar design (see Chapter 29).
TABLE 28-3 Comparison of Studies Investigating Pulsatile Flow during Cardiopulmonary Bypass
References | ||
---|---|---|
Proposed Beneficial Effect of Pulsatile Flow | Yes | No |
Reduced systemic vascular resistance | 108–114 | 115–123 |
Changes in systemic blood flow distribution | 108, 124 | 110, 115, 116, 121 |
Improved microcirculatory flow/aerobic metabolism | 108, 109, 111, 123, 125, 126 | 110, 115, 116, 121–123 |
Attenuation of hormonal responses | ||
Catecholamines | 127 | 109, 119 |
Renin/angiotensin | 114, 119, 122 | 115, 116, 127, 128 |
Antidiuretic hormone | 113, 127 | 117 |
Cortisol | 117, 128 | |
Thromboxane/prostacyclin | 120 | |
Improved renal blood flow or urine output | 111, 113, 115, 124, 126 | 110, 116–118, 123, 129, 136 |
Improved pancreatic blood flow | 124, 129 | |
Improved cerebral blood flow, metabolism, or outcome | 112, 125, 130–132, 140 | 137–139 |
Nonpulsatile CPB is the most commonly practiced form of artificial perfusion. As intuitive as it may seem that this type of nonphysiologic, nonpulsatile pump flow could be injurious, there is an overall lack of data to suggest that using pulsatile flow during clinical CPB has a neurologic benefit. In a large (N = 316), double-blind, randomized investigation by Murkin et al,138 examining the effect of pulsatile versus nonpulsatile CPB on neurologic and neuropsychologic outcome, no significant benefit was demonstrated. One study of balloon pump–induced pulsatile perfusion during CPB failed to show any improvements in jugular venous oxygen saturation of regional brain oxygenation.139 A significant limitation to most pulsatility studies is that, because of technical limitations, true “physiologic” pulsatility is almost never accomplished. Instead, variations of sinusoidal pulse waveforms are produced that do not replicate the kinetics and hydrodynamics of normal physiologic pulsation. A review by Hickey et al,107 published in 1983, offered important criticism and insight into this controversy and remains germane to recent reports. A fundamental difference between pulsatile and nonpulsatile flow is that additional hydraulic energy is required and applied to move blood when pulsatile flow is used. This extra kinetic energy is known to improve red blood cell (RBC) transit, increase capillary perfusion, and aid lymphatic function.133 The hydraulic power of pulsatile flow is the sum over time of the product of instantaneous pressure and instantaneous flow. CPB may influence many of the properties of the blood (viscosity) and the vasculature itself (arterial tone, size, and geometry) as a result of hemodilution, hypothermia, alteration of RBC deformability, and redistribution of flow. As a result of these changes, generation of what appears to be a normal pulsatile pressure waveform may not result in a normal pulsatile flow waveform. Simply reproducing pulsatile pressure is not sufficient to assure reproduction of pulsatile flow, nor does it allow quantification of energetics.
Virtually no study has quantified the energetics of the pulsatile or nonpulsatile perfusion used. Few studies report representative pressure waveforms.108–113 Even fewer give flow waveforms.134,135 When pulsatile flow is not quantified, critical features such as vascular impedance and the hydraulic power delivered cannot be evaluated (i.e., whether the pulsatile perfusion used in a particular study was really delivering more hydraulic power than the nonpulsatile perfusion with which it was compared). Grossi et al135 developed two indices of pulsatility: the pulsatility index, which quantitates the relative sharpness of a given waveform with respect to its mean flow; and the pulse power index, which quantifies the power of a pulsatile waveform compared with nonpulsatile equal flow. They found that despite use of a computer-controlled pulsatile pump, in every case, pulsatility index or pulse power index was considerably less than control (nonbypass pulsatility). Only with specific combinations of pulse rate and pulsatile flow contours, which had high pulsatility index or pulse power index, was lactate production lower than the nonpulsatile perfusion at the same minute flow during pulsatile CPB. This study indicates that not all pulsatile perfusion is the same and that pulsatile modes are not necessarily capable of improved perfusion relative to nonpulsatile systems.
It is, therefore, not surprising that such a wide disparity of results should occur. The authors are unaware of any human study in which pulsatility has been quantitated in terms other than pulse pressures. Consequently, whether the generated pressure waveform is a sine wave or some other pattern cannot be ascertained.113,114,117,119–123,126–128 The comparatively small size of the arterial inflow cannula effectively can filter out a large component of the pulsatile kinetic energy. Consequently, as achieved clinically, pulsatile flow may actually be quite similar energetically to nonpulsatile flow.
Newer pulsatile technologies may better reproduce the normal biologic state of cardiac pulsatility. Computer technologies that allow creating a more physiologic pulsatile perfusion pattern have, at least experimentally, demonstrated preservation of cerebral oxygenation. This approach showed some promise in a pig model of CPB in which pulsatile flow controlled by a computer to replicate the normal biologic variability in pulsatility was associated with significantly lower jugular venous oxygen desaturation during rewarming after hypothermic CPB.140 However, most studies do not present convincing evidence to suggest that routine pulsatile flow during CPB, as can be achieved by widely available technology, is warranted.
Acid-Base Management: Alpha-Stat versus pH-Stat
Optimal acid-base management during CPB has long been debated. Theoretically, alpha-stat management maintains normal CBF autoregulation with the coupling of cerebral metabolism (CMRO2) to CBF, allowing adequate oxygen delivery while minimizing the potential for emboli. Although early studies138 were unable to document a difference in neurologic or neuropsychologic outcome between the two techniques, later studies showed reductions in cognitive performance when pH-stat management was used, particularly in cases with prolonged CPB times.141 pH-stat management (i.e., CO2 is added to the fresh oxygenator gas flow) results in a greater CBF than is necessary for the brain’s metabolic requirements. This luxury perfusion risks excessive delivery of emboli to the brain. Except for congenital heart surgery, for which most outcome data support the use of pH-stat management142,143 because of its improvement in homogenous brain cooling before circulatory arrest, adult outcome data support the use of alpha-stat management.
Temperature and Rewarming Strategies
The use of hypothermia remains a mainstay of perioperative management in the cardiac surgical patient. Its widespread use relates to its putative, although not definitively proved, global organ-protective effects. Although hypothermia has a measurable effect on suppressing cerebral metabolism (approximately 6% to 7% decline per 1° C),144 it is likely that its other neuroprotective effects may be mediated by nonmetabolic actions. In the ischemic brain, for example, moderate hypothermia has multimodal effects, including blocking the release of glutamate,145 reducing calcium influx,146 hastening recovery of protein synthesis,147 diminishing membrane-bound protein kinase C activity,148 slowing of the time to onset of depolarization,149 reducing formation of reactive oxygen species,150 and suppressing nitric oxide (NO) synthase activity.151 Some or all of these effects in combination may convey some of the neuroprotective effects of hypothermia. Although experimental demonstrations of this are abundant, clinical examples of hypothermia neuroprotection have been elusive until recently.152–155
Some of the most meaningful data on CPB temperature and cerebral outcome came from work that had its origins in the late 1980s and early 1990s. It was at that time that warm CPB was used because of its putative myocardial salvaging effects when used with continuous warm cardioplegia.156–159 However, because CPB was being conducted at higher temperatures than what were considered conventional, the implications for the brain were also studied. Several large studies have been undertaken to elucidate the effects of temperature management on cerebral outcome after cardiac surgery. The Warm Heart Investigators trial,156 a trial performed at Emory University,160 and a later trial at Duke University,161 although having several methodologic differences, had similar results with respect to neurocognitive outcome,162,163 but some divergent results with respect to stroke. None of the studies, or ones performed since, demonstrated any neuroprotective effect of hypothermia on neurocognitive outcome after cardiac surgery. However, the Emory trial did demonstrate an apparent injurious effect (as manifest by a worse stroke outcome) of what were most likely mild degrees of hyperthermia during CPB. Neither the Warm Heart Investigators trial nor the Duke trial showed any effect of temperature on stroke per se. These data suggest that active warming to maintain temperatures at (or greater) than 37° C may pose an unnecessary risk for stroke.
Just as hypothermia has some likely protective effects on the brain, hyperthermia, in an opposite and disproportionate fashion, has some injurious effects. Although the studies referred to previously156,160,161 demonstrated no neuroprotective effect, there is emerging evidence that if some degree of neuroprotection is afforded by hypothermia, it may be negated by the obligatory rewarming period that must ensue.63 Grigore et al63 demonstrated in a prospective trial that compared with conventional “fast” rewarming, slower rewarming resulted in a lower incidence of neurocognitive dysfunction 6 weeks after cardiac surgery. These lower rewarming rates led to lower peak cerebral temperatures during rewarming, consistent with past observations that rapid rewarming can lead to an overshoot in cerebral temperature resulting in inadvertent cerebral hyperthermia.164 By reducing this rewarming rate, it reduces the overshoot in temperature and prevents the negative effects of cerebral hyperthermia. Consistent with the concept that preventing some of the rewarming may be protective was a study by Nathan et al165 that demonstrated an intermediate-term (3 months) neurocognitive benefit for patients who were maintained between 34° C and 36° C for a prolonged (12 hours) period after surgery. That trial may have had its beneficial effect by the avoidance of cerebral hyperthermia during rewarming rather than the prolonged hypothermia.165 However, the 5-year follow-up rate did not show a sustained benefit.166
Although there are numerous sites for monitoring temperature during cardiac surgery, several warrant special consideration. One of the lessons learned from the three warm versus cold trials, as well as from other information regarding temperature gradients between the CPB circuit, nasopharynx, and brain,164 is that it is important to monitor (and use as a target) a temperature site relevant to the organ of interest. If it is the body, a core temperature measured in the bladder, rectum, pulmonary artery, or esophagus is appropriate. However, if the temperature of the brain is desired, barring implantation of a thermistor directly into the brain (which actually has been done),167 it is important to look at surrogates of brain temperature. These include nasopharyngeal temperature and tympanic membrane temperature. More invasive surrogates of brain temperature have been obtained using a jugular bulb thermistor.164,168 Testing these different temperature sites has demonstrated that vast temperature gradients appear across the body and across the brain. It is likely that during periods of rapid flux (e.g., during rewarming), these temperature gradients are maximal.
Mean Arterial Pressure Management during Cardiopulmonary Bypass
The relation between blood pressure during CPB and CBF is pertinent to understanding whether MAP can be optimized to reduce neurologic injury. Tables 28-4 and 28-5 outline some of the pertinent studies regarding the relation (or lack thereof) between blood pressure and neurologic outcome. Plochl et al169 examined the lower threshold of the autoregulatory curve in dogs whereby further decreasing blood pressure would result in inadequate CBF and oxygen delivery during CPB. In that study, the brain became perfusion pressure dependent below 50 mm Hg. The investigators also demonstrated that hypothermia did not shift this threshold leftward. Clinically, the available data suggest that in an otherwise normal patient, CBF during nonpulsatile hypothermic CPB using alpha-stat blood gas management is largely independent of MAP as long as that MAP is within or near the autoregulatory range for the patient (i.e., 50 to 100 mm Hg).170 Although the autoregulatory curve traditionally is considered a horizontal plateau, this plateau has a slightly positive slope, but this slight upward slope is unlikely to have a significant clinically meaningful effect. For example, Newman et al17 demonstrated that under hypothermic conditions, CBF changed only 0.86 mL/100 g/min for every 10-mm Hg change in MAP. Although this change (1.78 mL/100 g/min) was greater with normothermia,171 these changes represented a relatively small fraction of the normal CBF of approximately 50 mL/100 g/min. Underlying essential hypertension as a comorbidity, however, likely is the effect of a rightward shift in the autoregulatory curve. The degree to which this rightward shift occurs is not clear, but it would be reasonable to expect that it is at least 10 mm Hg, suggesting that the lower range of autoregulatory blood flow is more likely to be 60 than 50 mm Hg.172 In addition, diabetes may lead to autoregulatory disturbances that make CBF more pressure passive than in individuals without diabetes.173,174
TABLE 28-4 Studies Supporting Relation between Intraoperative Hypotension and Postoperative Neurologic Dysfunction
First Author | Year | Patients |
---|---|---|
Gilman746 | 1965 | 35 |
Javid747 | 1969 | 100 |
Tufo748 | 1970 | 100 |
Lee749 | 1971 | 71 |
Stockard750 | 1973 | 25 |
Stockard751 | 1974 | 75 |
Branthwaite752 | 1975 | 538 |
Savageau753 | 1982 | 227 |
Gardner754 | 1985 | 168 |
Gold59 | 1995 | 248 |
TABLE 28-5 Studies Not Supporting Relation between Intraoperative Hypotension and Postoperative Neurologic Dysfunction
First Author | Year | Patients |
---|---|---|
Kolkka755 | 1980 | 204 |
Ellis756 | 1980 | 30 |
Sotaniemi757 | 1981 | 49 |
Slogoff758 | 1982 | 204 |
Govier170 | 1984 | 67 |
Nussmeier57 | 1986 | 182 |
Fish759 | 1987 | 100 |
Townes760 | 1989 | 90 |
Slogoff644 | 1990 | 504 |
Bashein761 | 1990 | 86 |
Stanley762 | 1990 | 19 |
Kramer763 | 1994 | 230 |
McKhann764 | 1997 | 456 |
Although the data relating MAP to neurologic and neurocognitive outcome after CABG surgery are inconclusive, most data suggest that MAP during CPB is not the primary predictor of cognitive decline or stroke after cardiac surgery. However, with increasing age, MAP during CPB may play a role in improving cerebral collateral perfusion to regions embolized, improving neurologic and cognitive outcome.17
Gold et al59 added significantly to the understanding of the influence of perfusion pressure on outcome after cardiac surgery in a study of 248 patients randomized to low (50 to 60 mm Hg) or high (80 to 100 mm Hg) MAP during CPB. Although a difference was demonstrated in their composite end point of combined adverse cardiac and neurologic outcome (4.8% high vs. 12.9% low; P = 0.026), when the individual outcomes were compared, there were similar trends but no statistical differences. A secondary analysis of the same data performed by Hartman et al38 found interesting interactions among pressure, aortic atheroma, and stroke; patients who were at risk for cerebral embolic stroke (from having severely atheromatous aortas) were more likely to manifest a stroke if MAP was maintained in the lower range than in the higher range. Intuitively, this is logical. Some experimental data in the noncardiac surgical setting suggest that collateral perfusion to penumbral areas of brain suffering from ischemic injury are relatively protected by higher perfusion pressure.60 Overall, it appears that MAP (in the normal range) has little effect on cognitive outcome; but in those with significant aortic atheroma, it may be prudent to modestly increase blood pressure.
Glucose Management
Hyperglycemia is a common occurrence during the course of cardiac surgery. Administration of cardioplegia containing glucose and stress response–induced alterations in insulin secretion and resistance increase the potential for significant hyperglycemia.175 Hyperglycemia has been repeatedly demonstrated to impair neurologic outcome after experimental focal and global cerebral ischemia.176–178 The explanation for this adverse effect likely relates to the effects that hyperglycemia have on anaerobic conversion of glucose to lactate, which ultimately cause intracellular acidosis and impair intracellular homeostasis and metabolism.179 A second injurious mechanism relates to an increase in the release of excitotoxic amino acids in response to hyperglycemia in the setting of cerebral ischemia.177 If hyperglycemia is injurious to the brain, the threshold for making injuries worse appears to be 180 to 200 mg/dL.180,181
Despite much experimental data, the role of glucose management on cerebral outcome after clinical CPB is not clear. Although Hindman et al182 cautioned about the use of glucose-containing prime for CPB, Metz and Keats183 did not find a difference in neurologic outcome in patients undergoing CPB with a glucose prime (blood glucose during CPB of 600 to 800 mg/dL) versus no glucose prime (blood glucose level of 200 to 300 mg/dL). This finding was supported by Nussmeier et al,184 who reported that use of a glucose-containing prime was not a risk factor for cerebral injury in nondiabetic or diabetic patients having CABG procedures. In the largest retrospective review, outcome data from 2862 CABG patients showed no association between the intraoperative maximum glucose concentration and major adverse neurologic outcome or in-hospital mortality.185,186
The appropriate type of perioperative serum glucose management and whether it adversely affects neurologic outcome in patients undergoing CPB remain unclear. The major difficulty in hyperglycemia treatment is the relative ineffectiveness of insulin therapy. Using excessive amounts of insulin during hypothermic periods may lead to rebound hypoglycemia after CPB. Chaney et al187 attempted to maintain normoglycemia during cardiac surgery with the use of an insulin protocol and came to the conclusion that even with aggressive insulin treatment, hyperglycemia often is resistant and may actually predispose to postoperative hypoglycemia. This concern over potentially increasing adverse effects by exerting tight glycemic controls reportedly has been demonstrated.188,189 Attempting to mediate injury may actually predispose to additional injury.
Off-Pump Cardiac Surgery
Off-pump coronary artery bypass (OPCAB) surgery frequently is used for the operative treatment of coronary artery disease (CAD). Although its ability to optimally treat CAD (through the demonstration of long-term graft patency) has not yet been shown in a long-term, prospective, randomized, controlled fashion, it is clear that OPCAB and similar operations will continue to be mainstays of cardiac surgery, although in an evolving fashion. Their impact on adverse cerebral outcomes after cardiac surgery has been variably reported.190
Although early data suggested less cognitive decline after OPCAB procedures, most studies have not seen it eliminated altogether. The reasons for this are unclear but likely reflect the complex pathophysiology involved. For example, if inflammatory processes play a role in initiating or propagating cerebral injury, OPCAB, with its continued use of sternotomy, heparin administration, and wide hemodynamic swings, all of which may contribute to a stress and inflammatory response, may be a significant reason why cognitive dysfunction is still seen. Ascending aortic manipulation, with its ensuing particulate embolization, is also still commonly used (see Chapter 18).
The results of the largest OPCAB study by Van Dijk et al191 to examine cognitive dysfunction showed that, although there was a reduction in cognitive decline in the early months after surgery, at 1 year,192 and even at 5 years,166 there were no differences between groups. In a subset of patients from this study, jugular bulb saturation was examined. More desaturation (indicative of ischemia risk to the brain) was seen in the OPCAB group. This may have been caused by the low cardiac output that can be seen during manipulation of the heart, as well as jugular venous hypertension.193 The effects of OPCAB on stroke risk have not been sufficiently analyzed, but one meta-analysis showed no beneficial effect on the incidence of stroke.194
Pharmacologic Neuroprotection
The CNS ischemic cascade (Figure 28-6) is triggered by reductions in CBF, globally or regionally, to the point at which the demands of cerebral metabolism can no longer be met.195 This depletion in cerebral energy stores leads to membrane ionic pump failure, resulting in a number of injurious events mediated by the influx of sodium, the opening of voltage-dependent calcium gates, a release of stored intracellular calcium, and overall membrane depolarization. Membrane depolarization results in the release of excitatory amino acids (e.g., glutamate, aspartate), with subsequent dramatic increases in intra-cellular calcium. This increase in cytoplasmic calcium propagates the cascade through the activation of a number of calcium-dependent enzymes, including endonucleases, NO synthase, various proteases, protein kinases, and phospholipases. If left unabated, these enzymes will result in neuronal death.
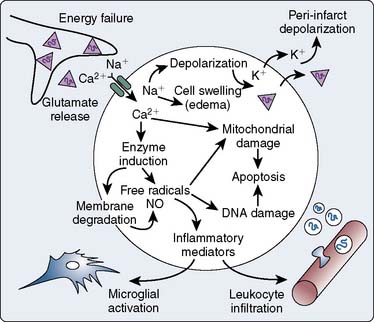
Figure 28-6 Cerebral ischemic cascade.
(From Dirnagl U, Iadecola C, Moskowitz M: Pathobiology of ischemic stroke: An integrated review. Trends Neurosci 22:391, 1999.)
No pharmacologic therapies have been approved by the U.S. Food and Drug Administration or foreign regulatory agencies for the prevention or treatment of cardiac surgery–associated cerebral injury, despite numerous previous investigations of specific pharmacologic agents in this setting (Table 28-6). This has not stopped progress, however, with multiple clinical trials ongoing. The most relevant cardiac surgery pharmacologic neuroprotection strategies, past and present, are reviewed here.
TABLE 28-6 Agents Studied as Pharmacologic Neuroprotectants during Cardiac Surgery
Agent | Study Reference |
---|---|
Thiopental | 57 |
Propofol | 200 |
Acadesine | 205 |
Aprotinin | 209, 210 |
Nimodipine | 220–222 |
GM1 ganglioside | 223 |
Dextromethorphan | 225 |
Remacemide | 225 |
Lidocaine | 230 |
β-Blockers | 232 |
Pegorgotein | 235 |
C5 complement inhibitor (pexelizumab) | 237 |
Lexiphant (platelet-activating factor antagonist) | 239 |
Clomethiazole | 244 |
Ketamine | 251 |
Thiopental
Thiopental was one of the first agents investigated as a potential neuroprotective agent for cardiac surgery. In a study by Nussmeier et al,57 thiopental was administered until electroencephalographic (EEG) isoelectricity was obtained before cannulation and continued until separation from CPB. In this study, neurologic complications on postoperative day 10 were significantly reduced in the thiopental group versus control subjects. Based on the encouraging results of this trial, high-dose thiopental frequently was used for valvular and other open ventricular procedures. The proposed mechanism of this effect related to the suppressive effects of barbiturates on cerebral metabolism. This mechanism, together with experimental data reporting the beneficial effects of the barbiturates,196 made it a logical choice for cardiac surgery. However, results of additional investigations of the use of thiopental were not as positive. Studies by Pascoe et al197 and Zaidan et al198 failed to support a beneficial effect of thiopental on neurologic outcome after cardiac surgery. These negative trials and the side effects of prolonged sedation with barbiturates served to quell the optimism for barbiturates. Retrospectively examining the initial Nussmeier et al57 study, the beneficial effects of the thiopental might not have been related to a direct neuroprotective effect, but to an indirect effect on reducing emboli-containing CBF. The well-known cerebral vasoconstricting effects of thiopental (matching CBF with a barbiturate-induced reduction in CMRO2) may have resulted in a reduction in embolic load to the brain during CPB and, as a result, a beneficial effect on neurologic outcome. It subsequently has been shown that isoelectricity itself is not necessary to incur a neuroprotective benefit from barbiturates.199 Evaluations of subisoelectric doses of thiopental have not been performed.
Propofol
Propofol has effects similar to those of thiopental on CMRO2 and CBF and has some antioxidant and calcium channel antagonist properties.200 Together with supportive data from experimental cerebral ischemia studies,201–203 propofol has been evaluated as a neuroprotectant in the setting of cardiac surgery. A prospective, randomized, clinical trial was conducted to determine whether propofol-induced EEG burst suppression would reduce the incidence or severity of cerebral injury during valvular surgery.204 In a randomized trial (n = 215) of burst-suppression doses of propofol, there was no beneficial effect on cognitive outcome at 2 months. The investigators concluded that EEG burst-suppression doses of propofol provided no neuroprotection during valvular cardiac surgery. No other studies in nonvalve cardiac surgery have assessed the effects of propofol on the brain.
Acadesine
The adenosine-regulating agent, acadesine, was studied in the early 1990s with the aim of improving myocardial outcome; stroke was examined as a secondary outcome.205 Compared with placebo, high- and low-dose infusions of acadesine resulted in a lower stroke rate (P = 0.016). Other adenosine-like agents have provided neuroprotection in preclinical experimental settings.206,207 Despite this positive, albeit indirect, clinical data and supportive experimental data, no further clinical neuroprotection indication for acadesine has been pursued.208 However, studies of this drug recently have been renewed. Whether this will have any effect on neurologic outcome is not known.
Aprotinin
Aprotinin is a nonspecific serine protease inhibitor that was first used in the 1950s for the treatment of pancreatitis. Its indication in cardiac surgery was for the prevention of blood loss and transfusion. In a large, multicenter trial of aprotinin for primary or redo CABG and valvular surgery evaluating its blood loss–reducing effects, the high-dose aprotinin group also had a lower stroke rate compared with placebo (P = 0.032).209,210 Similarly, Frumento et al211 retrospectively examined patients at high risk for stroke (because of the presence of significant aortic atheroma), and those who received aprotinin had a significantly lower stroke rate. In a small (N = 36) study examining the effect of aprotinin on cognitive deficit after CABG surgery, the incidence of cognitive deficit was reduced in the aprotinin group (58% aprotinin vs. 94% placebo; P = 0.01).212 However, the high rate in the placebo group, the small size of the study, and methodologic concerns limit the applicability of these results to broader populations.213 Animal investigations in the setting of cerebral ischemia failed to show any direct benefit on functional or neurohistologic outcome after cerebral ischemia.214
There has been considerable investigation of the potential mechanism for aprotinin-derived neuroprotection. Initial enthusiasm focused on its anti-inflammatory effects potentially preventing some of the adverse inflammatory sequelae of cerebral ischemia. However, aprotinin may have beneficial effects independent of any direct neuroprotective effect through an indirect effect of modulating cerebral emboli. Brooker et al42 identified the cardiotomy suction as a major source of cerebral emboli during CPB. By extrapolation, if a drug reduces the amount of particulate-containing blood returning from the operative field to the cardiotomy reservoir (by decreasing overall blood loss), cerebral emboli and the resulting neurologic consequences also may be decreased.
The potential adverse effects of aprotinin were reported by Mangano et al215 in their observational study of 4,374 patients. In that study, patients having received aprotinin had a significantly greater rate of cerebrovascular complications (P < 0.001). The Blood Conservation using Antifibrinolytics: A Randomized Trial (BART) reported a significant reduction in bleeding but an overall mortality risk with aprotinin compared with other antifibrinolytics.216 However, there were no differences in the stroke rate with aprotinin compared with tranexamic acid (2.5% aprotinin vs. 3.7% tranexamic acid [0.78 odds ratio, 95% confidence interval, 0.45 to 1.35]). Although the Mangano et al215 study and the BART trial contributed to the market withdrawal of aprotinin, the relevance of the potential neurologic effects of kallikrein inhibition remains. At least two other highly potent kallikrein inhibitors (CU-2010 and ecallantide) are being considered for clinical development, the neurologic effects of which are unknown.217–219
Nimodipine
Calcium plays a central role in propagating cerebral ischemic injury. For this reason, as well as a demonstrated beneficial effect of the calcium channel blocker nimodipine in subarachnoid hemorrhage and experimental cerebral ischemia, a randomized, double-blind, placebo-controlled, single-center trial was undertaken to assess the effect of nimodipine on neurologic, neuro-ophthalmologic, and neuropsychologic outcomes after valvular surgery.220–222 The trial was not completed after safety concerns regarding increased bleeding and death rates in the nimodipine group prompted an external review board to suspend the study after enrolling 150 of 400 patients planned to be studied. There also was no neuropsychologic deficit difference between the placebo or nimodipine groups at this interim review. As a result, the true effect of this drug or similar calcium blockers may never be fully known in this setting.
GM1 Ganglioside
The monosialoganglioside GM1 ganglioside has been investigated as a potential neuroprotectant during cardiac surgery.223 In addition to the potential beneficial effects of this type of compound on preserving neuronal membranes, some data suggest that it has a potential beneficial effect on reducing excitatory amino acid transmission.224 In a preliminary (but underpowered) cardiac surgery study, no beneficial effect was demonstrated. However, the study authors used this pilot trial to describe useful statistical methodology needed to measure differences in neurocognitive outcome, thereby constituting a template for later trials. This trial highlights one of the biggest difficulties in this investigative field: the interpretation of negative but underpowered studies.
Dextromethorphan
The N-methyl-d-aspartate (NMDA) receptor plays a major role in cerebral ischemic injury.195 Although human stroke trials have been limited by distressing psychomimetic side effects, a wealth of experimental data point to NMDA-receptor antagonists as being robust neuroprotective agents with a potential role in CPB-associated cerebral injury.225 Dextromethorphan, known for its antitussive activity, has some nonspecific NMDA antagonistic properties. A small (N = 12) pilot study examined dextromethorphan in the setting of pediatric cardiac surgery using EEG and magnetic resonance imaging end points to determine a difference between treatment groups but saw no difference, probably because of the small size of the study.226 No other studies have examined NMDA-receptor antagonism in the setting of pediatric cardiac surgery.
Remacemide
A second NMDA-receptor antagonist that has been evaluated for neuroprotection during CABG surgery is remacemide. In a well-designed and well-executed study by Arrowsmith et al,225 remacemide was given orally for 4 days before CABG. A neurocognitive battery was performed 1 week before and 8 weeks after CABG. A deficit was defined as a decrease in one standard deviation in 2 or more of the 12 tests within the neurocognitive battery. The patients were evaluated for their learning ability by subtracting the postoperative neurocognitive score from the preoperative score (formulating a Z-score). Although there was no difference between groups with respect to the dichotomous outcome of cognitive deficit (P = 0.6), examination of a continuous measure of learning ability showed there was a beneficial cognitive effect in the patients who received remacemide (P = 0.028). Despite these apparently beneficial results, because of the length of time that it took to perform this single-center trial, initial nonbeneficial preliminary results, and a prolonged period of data analysis and review for publication, this drug was not further pursued for this indication. It has, however, highlighted the potential utility of this class of drugs for this indication, and ongoing investigations examining other NMDA-receptor antagonists continue.227–229
Lidocaine
Intravenous lidocaine, because of its properties as a sodium channel blocking agent and potential anti-inflammatory effects, has been investigated as a neuroprotectant in cardiac surgery. In a study of 55 patients undergoing valvular surgery, a lidocaine infusion (in an antiarrhythmic dose of 1 mg/min) was begun preinduction and maintained for 48 hours after surgery.230 Neurocognitive testing was performed before surgery and 8 days, 2 months, and 6 months after surgery. Compared with placebo, neurocognitive outcome 8 days after surgery was significantly better in the lidocaine group (P = 0.025). However, a much larger double-blind, randomized trial in cardiac surgery failed to replicate the finding.231 Mathew et al,231 in a study of 241 patients, found no difference in the incidence of cognitive loss with the perioperative administration of lidocaine. Interestingly, they found that in patients with diabetes, and in those receiving high doses of lidocaine, that outcome was worse and may have confounded any potential benefit in the overall cohort receiving it. Lidocaine cannot be recommended at this time as a clinical neuroprotective agent in cardiac surgery, but it continues to be investigated.
β-Blockers
Although the use of β-blockers in patients with cardiac disease has been predominantly directed toward the prevention of adverse myocardial events, in a study of neurologic outcomes after cardiac surgery, β-blockers have been demonstrated to have mixed effects on neurologic outcomes. In a retrospective study of almost 3000 patients, stroke and encephalopathy were studied.232 Patients receiving β-blocker therapy had a significantly lower incidence of neurologic deficit versus those not receiving β-blockers. Although the reasons for this potential benefit are not clear, there are several potential reasons why β-blockers may be efficacious, including the modulation of cerebrovascular tone and CPB-related inflammatory events. Support for a potential neuroprotective effect from β-blockers has come from a study of carvedilol, which is known to have mixed adrenergic antagonist effects, as well as acting as an antioxidant and inhibitor of apoptosis233 (see Chapter 10). Any potential benefit to β-blocker therapy needs to be tempered by recent data in the noncardiac surgery population that demonstrated neurologic harm. The perioperative ischemic evaluation (POISE) trial, although demonstrating a reduction in myocardial infarction, demonstrated an increase in stroke rate in patients randomized to receive metoprolol perioperatively.234 It is unclear how this information pertains to the cardiac surgical population.
Pegorgotein
The generation of reactive oxygen species is a well-described pathophysiologic mechanism of ischemic/reperfusion injury. Combined with the whole-body inflammatory response associated with CPB and its own generation of reactive oxygen species, this mechanism has opened the field of neuroprotection and cardiac surgery to antioxidant therapies. Superoxide dismutase is involved in the catabolism of free radicals, and its mimetics have had beneficial results in the setting of experimental ischemia. Pegorgotein, a monomethyoxy-polyethyleneglycol covalently linked to superoxide dismutase, is protective against reperfusion-mediated cardiac and neuronal injury in animal studies.235 One study was initiated to examine whether pegorgotein would be associated with a reduced number of neurocognitive deficits after cardiac surgery.236 In this study of 67 patients undergoing primary elective CABG surgery (n = 22 to 23 in each of three groups: placebo, 200 IU/kg pegorgotein, or 5000 IU/kg pegorgotein), no difference in neurocognitive outcome was found.
C5 Complement Inhibitor: Pexelizumab
The activation of complement is central to the inflammatory response seen as a response to CPB.237 In a small (n = 18) study using a simple assessment of cognitive function, patients receiving an inhibitor to C5 (h5G1.1-scFv, pexelizumab), demonstrated fewer visuospatial deficits at hospital discharge.238 Additional large-scale (phase III) investigations of this compound to more adequately delineate any potential longer term neuroprotective effects from this drug in this setting have been performed. Mathew et al238 assessed pexelizumab in a 914-patient study aimed at evaluating its effect on myocardial outcome and mortality. A secondary end point of neurocognitive outcome demonstrated that pexelizumab, although having no effect on global measures of cognition, appeared to have a benefit with respect to the visuospatial domain.
Platelet-Activating Factor Antagonist: Lexiphant
Platelet-activating factor antagonists have demonstrated neuroprotective effects in experimental models of cerebral ischemia.239 Platelet-activating factor is thought to modulate postischemic injury by the release of cerebral cellular lipids and free fatty acids that may result in cellular injury and cerebral edema.240 In an investigation of 150 cardiac surgery patients receiving placebo or one of two different doses of lexiphant, no protective effects were found in neurocognitive outcome 3 months after cardiac surgery. This study was significantly underpowered, which is a recurring and troublesome feature of many studies in this field.241
Clomethiazole
Clomethiazole, which enhances γ-aminobutyric acid (GABA)-receptor activity, has been evaluated in CABG surgery. GABA repeatedly has been shown to be an important neuroprotective target in focal and global experimental ischemia.242,243 However, in a relatively large, well-designed, and well-conducted study, it failed to decrease neurocognitive dysfunction after cardiac surgery.244
Steroids
Corticosteroids have long been considered as potential cerebroprotective agents, in part, because of their ability to reduce the inflammatory response. Inflammation is considered an important factor in propagating ischemia-mediated brain injury.245,246 However, with the exception of spinal cord injury,247 steroids have never been demonstrated to possess any significant clinical neuroprotective properties. Furthermore, the administration of steroids actually has worsened cerebral outcome in a large trial (N = 10,000). The Corticosteroid Randomization after Significant Head Injury (CRASH) trial demonstrated an increased relative risk for death (1.18 [95% confidence interval, 1.09 to 1.27]; P = 0.0001) in those receiving high-dose steroids within 8 hours of injury.248,249 Part of their lack of effect may result from the hyperglycemia that generally follows their administration. Hyperglycemia in animal models and several human studies of cerebral injury has been associated with worsened neurologic outcome.180,250 The administration of steroids with the intent of conferring some degree of neuroprotection during cardiac surgery cannot be recommended. This therapeutic modality remains one that is being actively studied.
Ketamine
The neuroprotective effects of S(+)-ketamine, a frequently used anesthetic that is also an NMDA-receptor antagonist, were evaluated in a small (N = 106) study enrolling cardiac surgery patients.251 The incidence of neurocognitive dysfunction 10 weeks after surgery trended toward being lower in the ketamine group (20% for ketamine vs. 25% for controls; P = 0.54), but because the study was underpowered, it was not a significant change. Although some experimental evidence supports its role as a neuroprotectant, there is insufficient clinical evidence to support its use for this indication.252
Future Neuroprotective Drugs
Despite the failure thus far to discover a robust pharmacologic neuroprotectant, efforts continue in this investigative field. Most of these drug trials are using cognitive dysfunction, or mild cognitive impairment, as primary end points, although stroke is assumed (albeit with little evidence) to be on the same brain injury continuum. Dexanabinol is one such potential neuroprotective compound that is a synthetic noncompetitive NMDA-receptor antagonist. It also possesses some tumor necrosis factor-α antagonist properties. Its neuroprotective potential has been evaluated extensively experimentally in the setting of various models of cerebral ischemia.253,254 It currently is being evaluated in early-phase clinical trials in CABG for the prevention of neurocognitive dysfunction.
Acute renal injury
Despite concern for almost half a century over the seriousness of renal dysfunction as a complication,255–258 acute kidney injury (AKI) persists as a prevalent and important postcardiac surgery predictor of early mortality.259 Even during procedures in which there is no evidence of AKI by serum creatinine, more subtle markers often demonstrate renal tubular injury.260 Increasing degrees of AKI after cardiac surgery are associated with poorer outcome, greater costs, and more short- and long-term resource utilization.256,261–263 The degree of AKI also predicts poorer long-term survival in patients returning home.264–266 Notably, long-term outcome is just as strongly linked to the success of renal recovery as the magnitude of the injury; incomplete return of renal function occurs in up to one third of the patients.264–266 Although some of the harm associated with AKI simply reflects its accompaniment of other serious complications as an “epiphenomenon” (e.g., sepsis), there is also compelling evidence that AKI itself contributes to adverse outcome.267–272 Accumulation of “uremic toxins” beyond creatinine has widespread adverse effects on most organ systems,267,268,273 and where it is best studied in chronic renal disease, inadequate clearance of uremic toxins adversely affects survival.274 (See Chapter 37.)
Even when postoperative dialysis is avoided, the strong relation of AKI with adverse outcome continues to fuel the search for therapies to protect the kidney. Although practicing avoidance of the numerous recognized renal insults is a well-established approach to reducing AKI rates, the search for renoprotective strategies has otherwise been extremely disappointing. More recently, lack of progress has fueled a strategic reexamination of AKI with agreement that, compared with other conditions such as acute myocardial infarction (AMI) in which advances have been made, progress in AKI therapy has been hampered by its obligate delay in diagnosis (serum creatinine accumulation takes 48 to 72 hours) and poor agreement on its definition.275,276 Thus, strategic policies have reframed AKI along the lines of AMI, viewing it as a condition with threshold criteria, whose treatment demands prompt diagnosis and acute intervention.275 On a positive note, consensus serum creatinine thresholds are already available to diagnose AKI and are becoming widely embraced, whereas tools for the kidney allowing earlier diagnosis equivalent to the ST segment, creatine kinase-MB (CK-MB), and troponin for the heart may soon be available clinically.275–279

Clinical Course, Incidence, and Significance
Surgical procedure is important when considering postoperative AKI. The incidence varies widely by operation (Figure 28-7), each cardiac surgery having its own characteristic renal insult and pattern of serum creatinine change. For example, creatinine often declines immediately after CABG surgery (presumably because of hemodilution) but then increases, typically peaking on postoperative day 2, then returning toward or even below baseline values in subsequent days. Up to 30% of CABG patients sustain sufficient insult to meet threshold AKI criteria (e.g., RIFLE–injury275/AKIN criteria280: a creatinine increase > 0.3 mg/dL or 50% within the first 48 hours).256,261,281,282 The reported incidence varies according to the definition of kidney injury, as well as by the institution reporting their results (Figure 28-8). Even among patients with similar risk factors undergoing the same procedure, however, current models poorly predict the likelihood of AKI. AKI also has been linked to accelerated long-term renal decline that may lead to end-stage disease requiring chronic dialysis.283–288
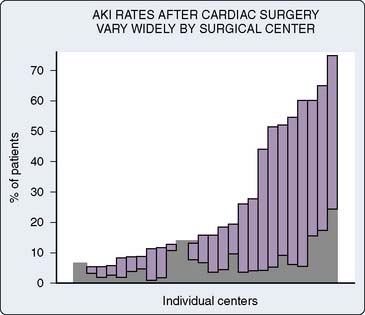
Figure 28-8 Even after adjusting for case complexity, among institutions there is wide variation in acute kidney injury (shaded bar represents risk, injury, or failure by ADQI-RIFLE-system)275 and dialysis (dark bar) rates, as exemplified by Heringlake and colleagues353 in a study of 2003 data from 26 German heart centers.
(Modified from Heringlake M, Knappe M, Vargas Hein O, et al: Renal dysfunction according to the ADQI-RIFLE system and clinical practice patterns after cardiac surgery in Germany. Minerva Anesthesiol 72:645–654, 2006, by permission.)
Of the 1% to 3% of patients sustaining AKI severe enough to require dialysis after CABG, up to 60% will die before hospital discharge, and many of the survivors will require continuing dialysis or be left with chronic kidney disease.289 Up to 20% of individuals presenting for nonemergent CABG surgery have chronic kidney disease.290,291 Although dialysis after cardiac surgery is always important, when this is associated with poor preoperative renal function, postoperative mortality rates more closely resemble similar patients who avoid dialysis.292 The rate of “renal recovery” after AKI is also difficult to predict, but emerging evidence suggests it is highly associated with outcome and apparently independent of AKI itself.265,293

Risk Factors and Surgery-Related Acute Kidney Injury Pathophysiology
Numerous studies have characterized risk factors for nephropathy after cardiac surgery (Figure 28-9).294 Despite an increasing understanding of perioperative renal dysfunction, known risk factors account for only one third of the observed variability in creatinine increase after cardiac surgery. Procedure-related risk factors include emergent and redo operations,295,296 valvular procedures,295,297,298 and operations requiring a period of circulatory arrest296 or extended durations of CPB.256,261,299–301 Infection and sepsis,296,299,302 atrial fibrillation,303 and indicators of low cardiac output states, including need for inotropic agents and insertion of an intra-aortic balloon pump during surgery, also have been associated with renal impairment.261,295,296,299,304
Preoperative demographic risk factors identified include advanced age,256,261,296,298,304,305 increased body weight,256 African American ethnicity,256,306 hypertension and wide pulse pressure,295,307,308 baseline anemia,309 peripheral or carotid atherosclerotic disease,256,295 diabetes, preoperative hyperglycemia and/or increased hemoglobin A1c in individuals without diabetes,256,261,307,310,311 reduced left ventricular (LV) function, and obstructive pulmonary disease.256,295,296,299,300 Interestingly, baseline chronic kidney disease is not a risk factor for AKI, but because even small amounts of additional renal impairment may lead to dialysis when severe renal disease is present at baseline, these individuals are at greatest risk for dialysis.
A genetic predisposition to AKI exists and explains more variation in postcardiac surgery AKI than conventional clinical risk factors alone.312,313 A handful of candidate polymorphisms known to affect inflammation and vasoconstriction have been studied, and several, alone or in combination, demonstrate strong associations with postcardiac surgery AKI.312,313 For example, CO possession of the IL-6 -572C and angiotensinogen (AGT) 842C polymorphisms in whites (6% of patients) predicts an approximately fourfold greater than average creatinine increase (121%) after CABG surgery.313 These data add credence to the idea that much of the variability in postoperative AKI may result from genetic influences313 and suggest that wider genetic profiling and future whole-genome studies may lead to useful preoperative risk prediction tools and may even provide a roadmap in the search for useful renoprotective strategies.
Postcardiac surgery AKI for an individual patient reflects the actual net injury from numerous potential perioperative sources known capable of inflicting insult (see Figure 28-9). Many markers of renal risk hint at causes of perioperative AKI in individuals. Thus, perioperative AKI contrasts with single-insult renal injuries such as contrast nephropathy. Nonetheless, a common pathway mediates the consequences of AKI including tubular and vascular cell dysfunction, necrosis, and apoptosis.314 Although details of the trigger mechanisms for this reflexive component of AKI remain elusive, there is better understanding of the direct renal consequences of some insults specific to cardiac surgery. Although the physiology of perioperative renal recovery is as yet less studied, emerging data suggest this is also likely to be important. A brief overview of AKI pathophysiology focused toward cardiac surgery is pertinent.
Using intraoperative epiaortic scanning, Davila-Roman et al315 found that ascending aortic atheroma burden correlates with AKI. Sreeram et al316 noted that postoperative AKI correlates with arterial emboli counts. Conlon et al,317 however, did not see a relation between renal artery stenosis and AKI. Renal atheroembolism is sometimes a dominant source of cardiac surgical AKI318 and often observed at autopsy.319 Filter devices deployed before cross-clamp removal often capture macroscopic atheroemboli.98 Plaque disruption caused by balloon pump counterpulsation also is a contributor to AKI.296,320 Antiembolism strategies have been adopted into the conduct of cardiac surgery,321–324 but evidence that this is decreasing AKI is lacking. One randomized filter device trial in a post hoc analysis found less AKI in higher-risk patients.99
Other emboli sources may be relevant to AKI in some circumstances. Fat droplets, particulates, and bubbles are common during cardiac surgery. Renal embolic infarcts from any source are wedge shaped and involve adjacent cortex and medulla, highlighting the vascular arrangement and lack of redundancy of kidney perfusion. Incriminated particulates other than atheroma include thrombus, platelet fibrin debris, septic vegetations, and even normal vessel wall.98,325 One third of surgery patients with bacterial endocarditis suffer significant AKI.326 Although sternal marrow lipid droplet reinfusion with red cell salvage is common327 and may have effects on renal cortical blood flow,328 its importance is unknown. Air bubbles rarely have been associated with AKI.329 Surgical field insufflation of CO2 reduces intravascular emboli,330 but whether this reduces AKI rates has not been assessed.
Many cardiac surgery patients meet the criteria for systemic inflammatory response syndrome in the early postoperative period. AKI is a major consequence of systemic inflammatory response syndrome.331 Sepsis is a strong predictor of postoperative AKI, likely mediated through the effects of renal inflammation.275,296,299,332 A surge of circulating proinflammatory cytokines is typical after trauma, major surgery, and CPB.333 Local cytokine release also occurs in response to ischemia/reperfusion-mediated renal nuclear factor-kappa B (NF-κB) activation.334 Finally, impaired renal filtration affects the course of any inflammatory response by influencing the primary clearance mechanism for many cytokines (e.g., IL-6, IL-1β, tumor necrosis factor-α).335
Many elements of cardiac surgery contribute to the risk for hypoperfusion and ischemia/reperfusion-mediated AKI. Embolism, low-output syndrome, and exogenous catecholamines can all contribute,296,316,336 leading to cellular high-energy phosphate depletion, calcium accumulation, oxygen free radical generation, local leukocyte activation, and NF-κB activation.337 These changes can cause apoptotic cell death through caspase activation (executioner enzymes) or tissue necrosis, or both.338 Apoptosis instigates more local inflammation and injury,339,340 and, experimentally, inhibition of caspase or NF-κB attenuates ischemia/reperfusion AKI.
Femoral artery cannulation can be complicated by leg ischemia and has been blamed for myoglobinuric AKI.341 Although statins can cause myopathy, these agents have not been associated with increased renal risk in vascular and major noncardiac surgery patients.342 Myoglobin and hemoglobin avidly bind NO and are believed to cause AKI through vasoconstrictor effects, but also through direct cytotoxicity and frank tubular obstruction.343
Withdrawal of the antifibrinolytic agent aprotinin from the market eliminates one concern of perioperative renal toxicity for cardiac surgery patients.344 In contrast, the lysine analog antifibrinolytics ε aminocaproic acid and tranexamic acid can raise concern because of their renal effects of small protein spillage into the urine (tubular proteinuria).345,346 A single retrospective analysis of 1502 patients involving the introduction of ε aminocaproic acid therapy did not find an increase in AKI.290 Although tubular proteinuria often heralds tubular injury, with lysine analog antifibrinolytics, this is completely resolved within 15 minutes after the agent is discontinued.345,346
Other perioperative nephrotoxins include some antibiotics,347 α-adrenergic agonist agents,348 cyclosporine,349 and nonsteroidal anti-inflammatory agents.350 However, the net effect on postcardiac surgery AKI of α1-mediated vasoconstriction and dopaminergic and α2-mediated renal vasodilation with hemodynamic compromise is unknown. Although experimentally, even short periods of high-dose norepinephrine cause long-lasting AKI,351 and catecholamines during cardiac surgery also predict AKI,296,352 disentangling cause and association for these agents is problematic because they are rarely used in the absence of other major risk factors (e.g., low output state). Interestingly, in a survey of German intensive care units (ICUs) involving more than 29,000 cardiac surgery patients, centers with worse AKI rates were more likely to prefer dopamine over other inotropes and avoid norepinephrine as a vasopressor.353
Intravenous contrast is known to have nephrotoxic effects. Contrast-associated nephropathy usually is heralded by a significant increase in serum creatinine within 5 days after intravascular contrast injection and occurs in approximately 2% to 7% of patients.354,355 The pathophysiology primarily results from vasoconstriction and direct renal tubular cell injury. Those at greatest risk typically have preprocedure renal impairment.356 In a study of 27 CABG patients, Garwood et al357 found indicators of tubular injury to be increased in those presenting for surgery within 5 days of cardiac catheterization relative to those further separated from an intravenous contrast injection. Use of low-osmolar contrast media and aggressive prestudy hydration have significantly reduced the risk for contrast-associated nephropathy for patients with diabetic nephropathy and other causes of chronic renal disease (Box 28-2).

Strategies for Renal Protection
Nonetheless, despite its limitations as an early biomarker, serum creatinine remains an important clinical tool because of its many other uses. Indisputably, creatinine accumulation serves as a prognostic “gold standard,” heralding AKI that is highly predictive of other major adverse outcomes including mortality.256 Validation for even the most promising of newer early AKI biomarkers is limited or lacking in comparison. In addition to injury, serum creatinine characterizes renal recovery unlike most AKI biomarkers. Renal recovery as reflected by declining creatinine levels is highly predictive of short- and long-term outcomes beyond the magnitude of kidney insult.265 Finally, the generalizability across studies and settings of creatinine-based consensus definitions for AKI, such as RIFLE and AKIN,280 are gaining popularity.
Early Acute Kidney Injury Biomarkers
The search for better early AKI biomarkers currently involves several contenders involving numerous physiologic mechanisms (Table 28-7). Markers such as creatinine and cystatin C that accumulate to diagnostic levels because of decreased clearance are further challenged during the perioperative period because of “signal-to-noise” confounders, such as hemodilution, which complicate early AKI recognition by disrupting steady-state assumptions. Although only a few new early biomarker candidates involve a substitute “ideal” creatinine, most involve one of three other early consequences of AKI: tubular cell damage, tubular cell dysfunction, and the adaptive stress response of the kidney. For example, damaged renal cells leak contents directly into urine; this strategy underpins “tubular enzymuria” AKI biomarkers including β-N-acetyl-β-d-glucosaminidase and at least eight other candidates. Monitoring markers of the kidney’s “stress” response provides another strategy for AKI recognition, including some frontrunners; these include neutrophil gelatinase-associated lipocalin, urinary IL-18, and at least three other candidates. Simple urinary Po2 monitoring correlates with changes in renal medullary oxygen levels and predicts subsequent AKI in cardiac surgery patients.
Early acute kidney injury (AKI) biomarkers reflect renal insult through diverse mechanisms. Understanding of the physiology underpinning each marker can aid in understanding their potential value to improve the diagnosis of AKI.
Cardiopulmonary Bypass Management and the Kidney
Profound hypothermia is a highly effective component of the protective strategy used during renal transplantation. Mild hypothermia during CPB would, therefore, seem to be a logical component of a perioperative renal protective strategy.358 However, three separate studies have not found any protective benefit of mild hypothermia during CPB.359–361 In the largest study, including 298 elective CABG patients who were randomly assigned to normothermic (35.5° C to 36.5° C) or hypothermic (28° C to 30° C) CPB, there was no association between normothermic (as compared with hypothermic) bypass and increased renal dysfunction after cardiac surgery.361
Low CPB blood pressure typically is not associated with the hypoperfusion characteristic of hypovolemic shock and low output states, conditions that are highly associated with AKI. Studies addressing the role of CPB perfusion pressure have not shown an association with AKI.305,310,362,363 A retrospective, multivariable analysis of minute-to-minute CPB blood pressure data from 1404 CABG surgery procedures, including an assessment of a degree-duration integral index of MAP less than 50 mm Hg, found no links between acute or extended episodes of hypotension during CPB and AKI.310
Moderate hemodilution is thought to reduce the risk for kidney injury during cardiac surgery through blood viscosity-related improvement in regional blood flow.364,365 However, the practice of extreme hemodilution (hematocrit < 20%) during CPB has been linked to adverse renal outcome after cardiac surgery.366,367 Accounting for perfusion pressure, in the study of 1404 CABG patients cited earlier,310 independent associations were noted for both lowest hematocrit during CPB and transfusion with postoperative AKI. Other studies have reported similar patterns and suggest that profound hematocrit change (e.g., > 50% decline) may be even less well tolerated, highlighting the importance of a clinical strategy including transfusion only after all measures of hemodilution avoidance have been taken.368–371
Glycemic control during CPB has been identified as a potential opportunity to attenuate AKI. However, recent evidence has called into question the influential findings of Van den Berghe et al,372 who reported reduced AKI and dialysis rates with postoperative therapy targeting tight serum glucose control (63% of study patients were postcardiac surgery). Despite widespread adoption of these intensive insulin protocols, numerous subsequent studies have failed to reproduce Van den Berghe et al’s findings of benefit. Wiener et al373 performed a meta-analysis of the available randomized ICU studies involving more than 3500 patients and found no reductions in AKI, dialysis rates, or mortality. In a study combining “Van den Berghe-like” postoperative management of 400 cardiac surgery patients randomized to intensive intraoperative insulin therapy (target, 80 to 100 mg/dL) versus usual management, Gandhi et al188 found no benefit and similar dialysis rates (6/199 vs. 4/201; P = 0.54), even noting an unexpected increase in 30-day mortality and stroke with tight control.
Pharmacologic Intervention
As evidenced by a recent Cochrane database review, few interventions are available to the clinician to pharmacologically prevent or treat established perioperative AKI.374 Proposed changes to improve the likelihood of success in finding renoprotective strategies have included increasing the size of studies designed to see benefit should it be present and, as outlined earlier, improving timely AKI detection to allow earlier intervention. Researchers have performed meta-analyses for many prevalent therapies by combining data from their randomized clinical trials. In some cases, these reports indicate that study size concerns may have been warranted. Potential optimism comes from a finding of reduced AKI in a meta-analysis of trials involving 934 cardiac surgery patients comparing various natriuretic peptides with placebo (urodilatin: 2 studies; brain natriuretic peptide [BNP]: 3 studies; atrial natriuretic peptide [ANP]: 9 studies)375; however, combined evaluation of the most studied single agent, ANP, still remained inconclusive.376 Another meta-analysis involving 20 studies assessing various methods to guide fluid and inotrope therapy to achieve hemodynamic optimization targets, involving 4220 patients, found reduced AKI and a trend toward reduced mortality.377 Notably, although hopeful, neither of these meta-analyses has sufficient evidence to make practical recommendations for changes in clinical practice.
Other systematic reviews and meta-analyses of collected AKI cardiac surgery studies recently have been reported. For example, several meta-analyses found N-acetylcysteine not to have renal benefit even in large cardiac surgery populations.378–381 A meta-analysis limited to randomized cardiac surgery trials of fenoldopam (20 studies, more than 1400 patients) concluded that a larger trial (1700 to 2300 patients) was needed to reconcile promising but conflicting evidence.382 Another less rigorous fenoldopam analysis of trials and observational studies (1059 patients, 13 studies) by Landoni et al383 suggested reduced dialysis and death after cardiac surgery. A meta-analysis of statin therapy before cardiac surgery including 3 randomized and 16 observational studies (more than 30,000 patients) found reduced incidence of renal failure with this therapy.384 A meta-analysis of 61 studies comparing “renal” dose dopamine with placebo (3359 patients) found extremely modest benefit on day 1—increased urine output, slight serum creatinine decline (4%), and GFR increase (6%).385 However, by day 2, these gains were lost, and there was no effect on mortality, need for dialysis, or adverse events. Meta-analyses of loop diuretics trials and controlled studies in critically ill patients described improvements in markers of renal function (urine output, oliguric period) and reduced need for dialysis, but also concerning trends toward increased mortality, and poorer renal recovery.386–388 Unfortunately, because of the limitations of current research tools, most potential renoprotective therapies have not been subjected to the rigor of a large randomized trial, or even meta-analysis, and none has been given the opportunity to be used soon after the onset of AKI. Additional data, including rationale and existing studies for a number of these therapies, are outlined later.
Dopamine
Mesenteric dopamine1 (D1)-receptor agonists increase renal blood flow, decrease renal vascular resistance, and enhance natriuresis and diuresis. Despite the absence of clinical evidence of renoprotection, this rationale has been used to justify the use of low-dose (“renal-dose”) dopamine (< 5 μg/kg/min) for decades. However, numerous double-blind, randomized studies in several surgical and nonsurgical settings have failed to demonstrate any renal benefits.389–391 Concerns have been raised that dopamine in cardiac surgery is not benign, including evidence suggesting impairment of hepatosplanchnic metabolism, despite an increase in regional perfusion,392 and increased postoperative arrhythmias.393 Despite the lack of benefit and accumulating concerns regarding the use of low-dose dopamine, many centers continue to use this agent for renoprotection.
Fenoldopam
Fenoldopam mesylate, a derivative of benzazepine, is a selective D1-receptor agonist. Although first approved as an antihypertensive agent, fenoldopam has shown promise in the prevention of contrast-induced nephropathy.394–396 There are, however, few randomized, controlled studies to evaluate the agent as a therapy for postoperative renal dysfunction. In one prospective, randomized study involving 160 patients with preoperative renal dysfunction, improved renal function with fenoldopam versus placebo was reported after cardiac surgery; however, no long-term benefit was evaluated.397 Other prospective, randomized, double-blind studies enrolling patients with established postoperative renal injury have proved inconclusive or even indicated possible adverse outcomes in patients with diabetes.398 More systematic study is necessary before this agent can be recommended for renoprotection in cardiac surgery.
Diuretic Agents
Loop diuretics, such as furosemide, produce renal cortical vasodilation and inhibit reabsorptive transport in the medullary thick ascending limb, causing more solute to remain in the renal tubule and increasing urine generation. In animal models, administration of furosemide and other loop diuretics has been shown to increase oxygen levels in the renal medulla,399 presumably by reducing oxygen consumption by tubular active transport; but it also results in distal tubular hypertrophy.400 In experimental models, loop diuretics have provided protection from renal tubular damage after ischemia/reperfusion and nephrotoxic injuries.401–403 In contrast with evidence from animal experiments, several clinical studies have shown no benefit and, possibly, even harm from perioperative diuretic therapy in cardiac surgery patients.404–406 In a double-blind, randomized, controlled trial comparing infusions of furosemide, low-dose dopamine, or placebo administered during and for 48 hours after surgery in 126 cardiac surgery patients, Lassnigg et al407 found no benefit of dopamine and a greater postoperative increase in serum creatinine in the group receiving furosemide. Although they may facilitate avoidance of dialysis in responsive patients by maintaining fluid balance in selected patients, there is insufficient evidence to support the routine use of loop diuretics as specific renoprotective agents. However, in situations of severe hemoglobinuria, they may facilitate urine production and tubular clearance of this nephrotoxin.
Mannitol, an osmotic diuretic, has been evaluated in several studies of cardiac surgical patients.359,408,409 Although an increased diuresis has been documented, few studies have carefully assessed postoperative renal dysfunction in these patients. In an animal model of thoracic aortic clamping, mannitol did not provide evidence of improved renal function after unclamping.410 In addition to the lack of beneficial effect on the kidney, several studies have identified a nephrotoxic potential of high-dose mannitol, especially in patients with preexisting renal insufficiency.411
Several studies have addressed the potential for renoprotection with natriuretic peptides. Three natriuretic peptides have received most of the attention in human trials: ANP (anaritide), urodilatin (ularitide), and BNP (nesiritide).412 Natriuretic peptides have primary effects, including receptor-mediated natriuresis and vasodilation, and normally are secreted in response to volume expansion. ANP increases glomerular filtration and urinary output by constricting efferent and dilating afferent renal arterioles.413 In a secondary analysis of randomized data from an ICU study of 504 patients with established AKI, Allgren et al414 noted a 24-hour intravenous infusion of ANP (0.2 μg/kg/min) was associated with improved dialysis-free survival in oliguric patients (8% vs. 27%; P = 0.008) but not in nonoliguric patients (59% vs. 48%; P = 0.03). Unfortunately, a repeat study designed to reproduce these favorable findings did not see any benefit.415 Few studies have evaluated urodilatin, and these have all provided inconclusive results.416 BNP has potent vasodilating properties and is generated in response to ventricular dilatation. Recent evidence from cardiology studies suggests that BNP treatment may worsen renal function in patients with heart failure.417,418 However, two randomized studies in cardiac surgery patients suggest renoprotective benefit from this agent.419,420
N-acetylcysteine
N-acetylcysteine is an antioxidant that enhances the endogenous glutathione scavenging system and has shown promise as a renoprotective agent by attenuating intravenous contrast-induced nephropathy. The weight of evidence, including four meta-analyses, suggests that potential benefits that may exist with contrast nephropathy are not pertinent to the perioperative patient.378–381,421,422
Adrenergic Agonists
The α1– and α2-adrenergic receptors in the kidney modulate vasoconstrictor and vasodilatory effects, respectively. Agents that attenuate renal vasoconstriction may have potential as renoprotective drugs because vasoconstriction most likely contributes to the pathophysiology of AKI. Clonidine, an α2-agonist, has been shown experimentally to inhibit renin release and cause a diuresis, and it has been evaluated in an experimental AKI model, confirming its potential as a renoprotective agent.423–427 Similarly, two clinical trials have demonstrated some promise. A prospective, double-blind, randomized, placebo-controlled clinical trial evaluating preoperative clonidine in 48 CABG patients found that creatinine clearance decreased over the first postoperative night from 98 ± 18 (before surgery) to 68 ± 19 mL/min (P < 0.05) in the placebo-treated group, but it remained unchanged in clonidine-treated patients (90 ± 1 to 92 ± 17 mL/min; P < 0.05).428 The effect was transient, however, with creatinine clearance in both groups being no different at postoperative day 3. Despite being positively supported in a second trial,429 clonidine has not gained popular acceptance as a renoprotective agent. Notably, decreased afferent α1-adrenergic receptor–mediated vasoconstriction has been suggested as an explanation for the renoprotective benefit of thoracic epidural blockade in cardiac surgery patients.430
Calcium Channel Blockers
Diltiazem is the calcium channel blocker that has been the most evaluated as a renoprotective agent in cardiac surgery, with its ability to antagonize vasoconstricting signals and reports of beneficial effects in experimental models of toxic and ischemic acute renal failure.431,432 However, in humans, numerous small, randomized trials and a retrospective study combined to provide a confusing picture, including evidence suggesting diltiazem therapy in cardiac surgery patients may have minor renal benefits, no benefit, or even potential harm.433–438
Sodium Bicarbonate
The perioperative infusion of sodium bicarbonate recently has attracted attention because of reduced AKI compared with a placebo saline infusion in 100 postcardiac surgery patients.439 Despite evidence that sodium bicarbonate-based hydration appears to be of benefit in other settings such as contrast-induced nephropathy, the considerable additional fluid and sodium loads required with this therapy have raised concern for some clinicians.440
Angiotensin-Converting Enzyme Inhibitor and Angiotensin-1–Receptor Blockers
The renin-angiotensin system mediates vasoconstriction and is important in the paracrine regulation of the renal microcirculation. Angiotensin-converting enzyme inhibitor and angiotensin- 1–receptor blocker agents act by inhibiting steps in activation of the renin-angiotensin system. Although angiotensin-converting enzyme inhibitor and angiotensin-1 receptor–blocker agents have demonstrated effects at slowing the progression of most chronic renal diseases,441 their role in AKI has not been well studied.442,443 In a study of 249 aortic surgery patients, Cittanova et al444 reported an increased risk for postoperative renal dysfunction in patients receiving preoperative angiotensin-converting enzyme inhibitor therapy. Animal studies suggest that both groups of drugs have protective properties in experimental AKI.445 In a small (N = 18), double-blind, placebo-controlled clinical trial enrolling CABG patients, preservation of renal plasma flow intraoperatively in patients receiving the angiotensin-converting enzyme inhibitor captopril relative to placebo was demonstrated.446 A similar study assessing perioperative enalaprilat (an angiotensin-1–receptor blocker) therapy in 14 CABG patients demonstrated greater renal plasma flow in the enalaprilat group before CPB and on postoperative day 7, as well as greater creatinine clearance after CPB.447,448
Insulin-Like Growth Factor
The concept of combining AKI prevention with enhanced renal recovery is appealing given the emerging evidence; insulin-like growth factor offers the potential for the importance of the latter, evidenced by promising findings from animal AKI studies and reports in humans with chronic kidney disease of improved renal function and delayed need for dialysis.449–451 However, unfortunately, the only randomized controlled trial in 72 patients with acute renal failure found no renal benefit and a significant side effect profile.452
Alternate Perioperative Renoprotective Strategies
In a survey involving more than 29,000 cardiac surgery patients from 26 German heart programs, Heringlake et al353 reported extremely wide variation in AKI rates by center, ranging from 3.1% to 75% (mean, 15.4%; see Figure 28-9). Although programs were unaware of their AKI ranking when responding to a questionnaire, centers were asked to provide case mix data and answer questions on their standard practice in patients with increased renal risk. Interestingly, groups of centers with higher and lower AKI rates had similar case mix and urgency/emergency rates, but several differences existed in their responses to standard management questions. High and low AKI centers used loop diuretics at about the same rates but selected norepinephrine in preference to epinephrine or dopamine as a vasopressor, and they were less likely to prefer dopamine for either inotropy or renal prophylaxis. Using monitoring of AKI rates among centers as a tool to identify outliers and alter practice patterns was a useful strategy to improve outcomes in one study.262
Myocardial injury
From the earliest days of modern cardiac surgery, perioperative myocardial dysfunction, with its associated morbidity and mortality, has been reported.453 Evidence, including substantial subendocardial cellular necrosis, led to the conclusion that this injury resulted from an inadequate substrate supply to the metabolically active myocardium.454 Optimizing myocardial protection during cardiac surgery involves several compromises inherent in allowing surgery to be performed in a relatively immobile, bloodless field while preserving postoperative myocardial function. The fundamental tenets of this protection center on the judicious use of hypothermia together with the induction and maintenance of chemically induced electromechanical diastolic cardiac arrest. Bigelow et al455 were the first to describe the use of hypothermia for this purpose, and this was complemented by subsequent work by Melrose et al,456 who first reported the electromechanical arrest of the heart by the administration of potassium-containing cardioplegia. Despite continued efforts directed at myocardial protection, it is clear that myocardial injury, although reduced, still remains a problem, and with it, the representative phenotype of postoperative myocardial dysfunction (see Chapters 29, 32, and 34).

Incidence and Significance of Myocardial Dysfunction after Cardiopulmonary Bypass
Unlike other organs at risk for damage during cardiac surgery, it is assumed, because of the very nature of the target of the operation being performed, that all patients having cardiac surgery will suffer some degree of myocardial injury. Although the injury can be subclinical, represented only by otherwise asymptomatic increases in cardiac enzymes (e.g., myocardial creatine kinase isoenzyme [CK-MB]), frequently it manifests more overtly. The degree to which these enzymes are released by injured myocardium, frequently to levels sufficiently high to satisfy criteria for myocardial infarction, have been related to perioperative outcome after cardiac surgery.457–459 Chaitman et al458 reported CK-MB results from the Guard During Ischemia Against Necrosis (GUARDIAN) trial in 11,950 patients with acute coronary syndromes or undergoing high-risk percutaneous intervention or CABG surgery. CK-MB values more than 10 times the upper limit of normal during the initial 48 hours after CABG were significantly associated with 6-month mortality (P < 0.001).458

Risk Factors for Myocardial Injury
With an increasingly sicker cohort of patients presenting for cardiac surgery,460 many with acute ischemic syndromes (e.g., often with evolving AMI) or significant LV dysfunction, the need has never been greater for optimizing myocardial protection to minimize the myocardial dysfunction consequent to aortic cross-clamping and cardioplegia.461 The continued increase in cardiac transplantation and other complex surgeries in the heart failure patient has served to fuel the search for better myocardial protection strategies.

Pathophysiology of Myocardial Injury
Myocardial stunning represents the myocardial dysfunction that follows a brief ischemic event. It is differentiated from the reversible dysfunction associated with chronic ischemia, which is termed hibernation.462 Myocardial stunning typically resolves over the 48 to 72 hours after the ischemic event and frequently is observed after aortic cross-clamping with cardioplegic arrest.463,464 Important factors that contribute to stunning include not only the metabolic consequences of oxygen deprivation but also the premorbid condition of the myocardium, reperfusion injury, acute alterations in signal transduction systems, and the effects of circulating inflammatory mediators.
Predictably, with the release of the aortic cross-clamp and the restoration of blood flow, myocardial reperfusion occurs. With reperfusion, the paradox represented by the balance of substrate delivery restoration needed for normal metabolism that also can serve as the substrate for injurious free radical production, becomes a significant issue for consideration. Reperfusion causes a rapid increase in free radical production within minutes, and it plays a major role initiating myocardial stunning. Bolli et al465 identified the importance of this effect by demonstrating that antioxidants administered just before reperfusion significantly diminished myocardial stunning, an effect not observed if the same substances were introduced after reperfusion. Sun et al466,467 subsequently showed the significance of the free radical effect by demonstrating that up to 80% of myocardial stunning could be avoided with an appropriately timed regimen of free radical scavengers.
Free radical insults during myocardial reperfusion result in the near-immediate dysfunction of proteins involved in ion transport and excitation-contraction coupling, as well as injury to membranes through damage mediated through lipid peroxidation.468 Free radical–mediated myocardial dysfunction is related to a myofilament defect manifested by an impairment in Ca-activated excitation-contraction coupling.468 Although free radicals can directly injure these myofilament components, injury to other proteins related to ion transport and membrane integrity serve to increase intracellular calcium. Several mechanisms have been proposed to explain this impairment of myofilament function, although the most likely explanation includes physiologic downregulation of excitation-contraction coupling in response to cellular Ca2+ overload. The mechanisms underlying Ca2+ overload are not well understood, but they are thought to involve free radical–related and free radical–independent abnormalities in Ca2+ homeostasis. Whatever the cause, the rapid calcium influx during myocardial reperfusion quickly can overload the myocyte with Ca2+.469 The importance of Ca2+ overload is demonstrated by the substantial protection from reperfusion injury obtained by pretreatment with traditional Ca2+ blocking agents.470
A free radical–independent mechanism for Ca2+ overload involves activation of the Na+/H exchanger during reperfusion in an attempt by the cell to correct intracellular pH.471 The resultant increase in intracellular Na+ from activation of the Na+/ H+ exchanger further activates the Na+/Ca2+ exchanger, increasing Ca2+ influx.472 Evidence for the activation of Na+/ H+ exchangers being responsible for some of the harmful effects of reperfusion is shown by the benefits that Na+/H+ exchanger inhibitors have had on myocardial stunning with reductions in arrhythmias, improvements in systolic and diastolic function, and reductions in myocyte injury.473 In addition to its role in Ca2+ overload, activation of the Na+/H+ exchanger has been linked to increased phospholipase activity, generation of prostaglandins and other eicosanoids, and activation of platelets and neutrophils—all potentially injurious processes.471,473
In addition to free radical upregulation, myocardial reperfusion associated with acute myocardial ischemic injury induces inflammation mediated by neutrophils and an array of humoral inflammatory components.471,474,475 Several studies using novel neutrophil inhibitors have demonstrated a cardioprotective effect in models of myocardial stunning.475,476 Prostaglandins also are generated during reperfusion. The adverse effects of prostaglandins appear to be synergistic with increases in intracellular calcium. The relation of leukotriene and cytokine release to reperfusion injury is less clear.471,474 Consistent with a role for prostaglandins in this injury is the demonstration that inhibition of prostaglandins by nonsteroidal anti-inflammatory agents can significantly diminish myocardial stunning.477
A potential additional mechanism for myocardial dysfunction specific to the setting of CPB relates to proposed acute alterations in β-adrenergic signal transduction.478 Acute desensitization and downregulation of myocardial β-adrenergic receptors during CPB have been demonstrated after cardiac surgery.478,479 Although the role of the large increases in circulating catecholamines seen with CPB on β-adrenergic malfunction is unclear, it has been proposed that an increased incidence of post-CPB low cardiac output states and reduced responsiveness to inotropic agents may, in part, be attributed to this effect.480,481

Myocardial Protection during Cardiac Surgery: Cardioplegia
Optimizing the metabolic state of the myocardium is fundamental to preserving its integrity. The major effects of temperature and functional activity (i.e., contractile and electrical work) on the metabolic rate of myocardium have been extensively described.454,472,482 With the institution of CPB, the emptying of the heart significantly reduces contractile work and myocardial oxygen consumption (Mvo2). Nullifying this cardiac work reduces the Mvo2 by 30% to 60%. With subsequent reductions in temperature, the Mvo2 further decreases, and with induction of cardiac arrest and hypothermia, 90% of the metabolic requirements of the heart can be reduced (Figure 28-10). Temperature reductions diminish metabolic rate for all electromechanical states (i.e., beating or fibrillating) of the myocardium (Table 28-8).
TABLE 28-8 Influence of Temperature on Myocardial Oxygen Consumption for Different Work and Electrical Conditions
Although cardiac surgery on the empty beating heart or under conditions of hypothermic fibrillation (both with the support of CPB) is sometimes performed, aortic cross-clamping with cardioplegic arrest remains the most prevalent method of myocardial preservation. Based on the principle of reducing metabolic requirements, the introduction of selective myocardial hypothermia and cardioplegia (i.e., diastolic arrest) marked a major clinical advance in myocardial protection.483,484 With the various additives in cardioplegia solutions (designed to optimize the myocardium during arrest and attenuate reperfusion injury) and the use of warm cardioplegia, the idea of delivering metabolic substrates (as opposed to solely reducing metabolic requirements) also is commonplace. Several effective approaches to chemical cardioplegia are used. The clinical success of a cardioplegia strategy may be judged by its ability to achieve and maintain prompt continuous arrest in all regions of the myocardium, early return of function after cross-clamp removal, and minimal inotropic requirements for successful separation from CPB. Composition, temperature, and route of delivery constitute the fundamentals of cardioplegia-derived myocardial protection (see Chapter 29).
Composition of Cardioplegia Solutions
The composition of the various cardioplegia solutions used during cardiac surgery varies as much between institutions as it does between individual surgeons. In general terms, cardioplegia can be classified into blood-containing and nonblood-containing (i.e., crystalloid) solutions. Crystalloid cardioplegia has fallen out of favor, with blood cardioplegia in various combinations of temperatures and routes of delivery being the most used solution. However, even within the category of blood cardioplegia, the individual chemical constituents of the solution vary considerably with respect to the addition of numerous additives. Table 28-9 outlines the various additives to cardioplegia solutions together with their corresponding rationale for use. Although all cardioplegia solutions contain greater than physiologic levels of potassium, solutions used for the induction of diastolic arrest contain the greatest concentrations of potassium as opposed to solutions used for the maintenance of cardioplegia. In addition to adjustment of electrolytes, manipulation of buffers (e.g., bicarbonate, tromethamine), osmotic agents (e.g., glucose, mannitol, potassium), and metabolic substrates (e.g., glucose, glutamate, and aspartate) constitute the most common variations in cardioplegia content. Oxygenation of crystalloid cardioplegia before infusion is aimed at increasing aerobic metabolism, but the limited oxygen-carrying capacity of crystalloid makes a rapid decline in metabolic rate through immediate and sustained diastolic arrest critical to effective cardioprotection with this technique.
TABLE 28-9 Strategies for the Reduction of Ischemic Injury with Cardioplegia
Principle | Mechanism | Component |
---|---|---|
Reduce O2 demand | Hypothermia | Blood, crystalloid, ice slush, lavage |
Perfusion | ||
Topical/lavage | ||
Asystole | KCl, adenosine (?), hyperpolarizing agents | |
Substrate supply and use | Oxygen | Blood, perfluorocarbons, crystalloid (?) |
Glucose | Blood, glucose, citrate-phosphate-dextrose | |
Amino acids | Glutamate, aspartate | |
Buffer acidosis | Hypothermia (Rosenthal factor), intermittent infusions | |
Buffers | Blood, tromethamine, histidine, bicarbonate, phosphate | |
Optimize metabolism | Warm induction (37° C), warm reperfusion | |
Reduce Ca2+ overload | Hypocalcemia | Citrate, Ca2+ channel blockers, K channel openers (?) |
Reduce edema | Hyperosmolarity | Glucose, KCl, mannitol |
Moderate infusion pressure | 50 mm Hg |
From Vinten-Johansen J, Thourani VH: Myocardial protection: An overview. J Extra Corpor Technol 32:38, 2000.
Blood cardioplegia has the potential advantage of delivering sufficient oxygen to ischemic myocardium to sustain basal metabolism or even augment high-energy phosphate stores, as well as possessing free radical scavenging properties.485 The introduction of blood cardioplegia in the late 1970s followed recognition of the clinical utility of this technique.486 Although low-risk cardiac surgical patients appear to do equally well with crystalloid or blood cardioplegic protection, evidence is compelling that more critically ill patients, including those with “energy-depleted” hearts (e.g., cardiogenic shock, AMI before CPB), have improved outcomes using blood cardioplegia.487,488 Patients at high risk also appear to have better recovery using a combination of antegrade and retrograde blood cardioplegia delivery, when compared with antegrade administration alone.488
Because an infusion of oxygenated blood cardioplegia is in many ways similar to myocardial reperfusion, it is not surprising that the composition of blood cardioplegia is based on reperfusate parameters known to minimize myocardial stunning.489,490 These parameters include maintenance of Ca2+ at 1.0 mEq/L (by chelating Ca2+ from perfusate blood) to diminish myocyte Ca2+ uptake; pH between 7.6 and 7.8 (the pH of water in the hypothermic temperature range used); osmolality between 340 and 360 mOsm to minimize edema-related myocardial dysfunction after reperfusion; and hyperkalemia between 10 and 25 mEq/L to safely sustain electromechanical arrest. Blood is mixed in a ratio of 4:1 with a prepared crystalloid solution to create blood cardioplegia with these characteristics.
Infusion of a single, warm (37° C) reperfusion dose of cardioplegia (so-called hot shot) containing metabolic substrates (i.e., glucose, glutamate, and aspartate) just before aortic cross-clamp removal is preferred by some clinicians. The rationale for this is evidence that normothermia maximally enhances myocardial aerobic metabolism and recovery after an ischemic period. Although some have advocated continuous infusion of hyperkalemic warm cardioplegia throughout the period of aortic cross-clamping,491 this technique has not gained wide popularity for CABG because of the technical challenges of grafting vessels perfused in this way and the threat of ischemia to nonperfused (warm) myocardium during grafting.
Numerous other cardioplegia additives continue to be assessed involving various buffers, osmotic agents, metabolites, ATP and precursors, enzymes controlling ATP synthesis and catabolism, oxygen radical scavengers and antioxidants. Protection of myocardial β-adrenergic receptor function using intracoronary administration of esmolol appears to hold promise as an alternate cardioprotective method.492 Alternative cardioplegia strategies potentially extending safe ischemic periods for heart transplantation to up to 24 hours also are being evaluated.493–495
Cardioplegia Temperature
The composition of cardioplegia solutions varies considerably; in contrast, myocardial temperature during cardioplegia is almost uniformly reduced to between 8° C and 10° C or less by the infusion of refrigerated cardioplegia and external topical cooling with ice slush. However, the introduction of warm cardioplegia has challenged the once universally accepted necessity of hypothermia for successful myocardial protection.156 Although hypothermic cardioplegia is the most commonly used, numerous investigations have examined tepid (27° C to 30° C) and warm (37° C to 38° C) temperature ranges for the administration of cardioplegia. Much of the work aimed at determining the optimum temperature of the cardioplegia solution centered on the fact that, although hypothermia clearly offered some advantages to the myocardium in suppressing metabolism (particularly when intermittent cardioplegia was delivered), it may have some detrimental effects.
The deleterious effects of hypothermia include the increased risk for myocardial edema (through ion pump activity inhibition) and the impaired function of various membrane receptors on which some pharmacologic therapy depends (such as the various additives to the cardioplegia solutions). The other disadvantages of hypothermic cardioplegia, in addition to the production of the metabolic inhibition in the myocardium, are an increase in plasma viscosity and a decrease in RBC deformability.157,482,496 As a result, investigations aimed at using warmer cardioplegia temperatures have been conducted.156,497 During the initial phase of cardioplegia delivery, hypothermic temperatures, in addition to inhibiting some of the needed drug-receptor interactions, fail to optimize the metabolic rate in the myocardium. Hypothermia results in a leftward shift in the oxygen hemoglobin dissociation curve, inhibiting the release of oxygen into tissues. The myocardium is relatively ischemic during this initial induction phase of cardioplegia, with the uptake of the oxygen to this tissue being low and, as a result, significant oxygen debt occurs.
A compromise temperature (tepid, 27° C to 30° C) also has been proposed.497 Ikonomidis et al497 compared outcomes for patients receiving warm, tepid, or cold cardioplegia. Although numerous differences were found among the various groups, the recovery of LV stroke work at 1 and 4 hours after surgery was optimal in the tepid group. The researchers concluded that tepid cardioplegia provided better overall protection with superior functional recovery.498 Hayashida et al498 conducted a randomized trial comparing the effects of cold (9° C), tepid (29° C), and warm (37° C) cardioplegia in 42 patients undergoing CABG surgery. Overall, the investigators found that Mvo2 and lactate production were greatest in the warm group, intermediate in the tepid group, and least in the cold cardioplegia group. However, early postoperative LV function was optimized in the tepid cardioplegia group.498
Cardioplegia Delivery Routes
If using tepid or warm cardioplegia administration, the continuous administration of this cardioplegia needs to be ensured. Retrograde cardioplegia, in which a cardioplegia catheter is introduced into the coronary sinus, allows for almost continuous cardioplegia administration. Retrograde delivery is also useful in settings in which antegrade cardioplegia is problematic, such as with severe aortic insufficiency or during aortic root or aortic valve (and, frequently, mitral) surgery. It also allows the distribution of cardioplegia to areas of myocardium supplied by significantly stenosed coronary vessels. Retrograde cardioplegia has proved safe and effective for cardioplegia in patients with CAD and in those undergoing valve surgery.499,500 With the administration of retrograde cardioplegia, certain provisos should be considered. The acceptable perfusion pressure to limit perivascular edema and hemorrhage needs to be limited to less than 40 mm Hg.501
Two trials added information about cardioplegia routes of delivery. The CABG patch trial502 enrolled high-risk CAD patients with impaired LV function and demonstrated the superiority of the combined antegrade and retrograde delivery of blood cardioplegia compared with antegrade cardioplegia alone. The limitation of this trial was that the antegrade group received crystalloid cardioplegia (as opposed to blood cardioplegia in the antegrade-retrograde group), raising questions about whether the differences in the groups were seen because of the route of administration or the constituents of the cardioplegia itself. A second trial failed to demonstrate any differences when the administration of intermittent antegrade cold blood cardioplegia was compared with a group receiving antegrade cold blood cardioplegia induction followed by retrograde cold blood maintenance for valve surgery.503 They did find that the antegrade-retrograde approach was technically more convenient, allowing for shorter aortic cross-clamp times.
Retrograde cardioplegia does have some limitations. Although the retrograde approach has been shown to effectively deliver cardioplegia adequately to the left ventricle, because of shunting and blood flowing into the atrium and ventricles by the thebesian veins and various arteriosinusoidal connections, the right ventricle and septum frequently receive inadequate delivery of cardioplegia. Difficulties with retrograde delivery also can occur if the coronary sinus catheter is placed beyond the great cardiac vein, or if anatomic variants occur that communicate with systemic veins, such as a persistent left superior vena cava (SVC).501,504,505 Because retrograde cardioplegia is inefficient in producing arrest of the beating heart, induction of arrest with this technique must be achieved by a single antegrade infusion of cardioplegia before its institution (Box 28-3; see Chapter 29).
Ischemic Preconditioning
Myocardial stunning during cardiac surgery is affected by several parameters. The preischemic state of the myocardium can influence the degree of stunning that follows an ischemic event. Ischemic preconditioning (IPC) is endogenous myocardial protection triggered by exposure to brief (5 to 15 minutes) periods of ischemia. IPC is a natural defense mechanism that permits the heart to better tolerate myocardial ischemia. Although brief ischemic episodes in themselves result in stunning, they also build up a temporary resistance to the adverse effects of subsequent more prolonged ischemia.506,507 IPC has been well described experimentally.508–510 Several proposed mechanisms are responsible for IPC, including the activation of several myocardial G protein–coupled receptors, most notably A1 adenosine and α1-adrenergic receptors.511 Protein kinase C appears to be a key cellular mediator of IPC, in part through activation of ATP-sensitive potassium channels.512
Myocardial protection strategies continue to be an active area of investigation, including assessment of IPC. Attempts to induce IPC by brief ischemia or pharmacologic means before CPB have been assessed in human cardiac surgical patients. Sevoflurane, a frequently used volatile anesthetic, has been demonstrated to pharmacologically replicate IPC.513 Administration of adenosine, before bypass or in cardioplegic solutions, has been studied as a pharmacologic means to induce IPC. It has been associated with reduced postoperative myocardial injury, reduced inotropic requirements, and improved myocardial recovery.514,515 The potential for myocardial stunning after beating heart OPCAB has not been fully assessed. Intermittent coronary occlusion before OPCAB to induce IPC, although unclear as to its effect, has also undergone clinical assessment.516 (See Chapter 9.)
Gastrointestinal complications

Incidence and Significance
Gastrointestinal (GI) complications after cardiac surgery, although occurring relatively infrequently (0.5% to 5.5%), portend a significantly increased risk for overall adverse patient outcome. The variability in the reported incidence of GI complications is partly a reflection of how they are defined, as well as the variable patient and operative risk factors in the studied cohorts.517–528 As devastating as they are, because of the relative low incidence, studies of GI complications are few. Although the most commonly considered GI complications include pancreatitis, GI bleeding, cholecystitis, and bowel perforation or infarction, hyperbilirubinemia (total bilirubinemia > 3.0 mg/dL) also has been described as an important complication after cardiac surgery. In one of the largest prospective studies examining these complications after CPB, McSweeney et al525 studied 2417 patients undergoing CABG (with or without concurrent intracardiac procedures) in a multicenter study in the United States. The overall incidence rate of GI complications in this study was 5.5%, ranging from 3.7% for hyperbilirubinemia to 0.1% for major bowel perforation or infarction (Table 28-10).
In addition to their association with other morbid events, adverse GI complications are significantly associated with increased mortality after cardiac surgery.525,529–531 The average mortality among subtypes of GI complications in the study by McSweeney et al525 was 19.6%, and in other reports, the mortality rate ranges from 13% to 87%, with an overall average mortality rate of 33%. Even the seemingly insignificant complication of having an increased laboratory measurement of total bilirubin was associated with a 6.6 odds ratio of death in McSweeney et al’s study, compared with a death odds ratio of 8.4 for all adverse GI outcomes combined. Apart from the significant effect on mortality, the occurrence of an adverse GI outcome also significantly increases the incidence of perioperative myocardial infarction, renal failure, and stroke, as well as significantly prolonging ICU and hospital length of stay.525

Risk Factors
Many preoperative, intraoperative, and postoperative risk factors for GI complications have been identified in a number of studies.523,525,532–537 Because many factors are associated with one another, it is only when these risk factors are examined in multivariable analyses that a more accurate understanding of what the most significant risk factors for visceral complications after cardiac surgery are. Table 28-11 represents the most consistently reported risk factors for GI complications after cardiac surgery: Before surgery, age (> 75 years), history of congestive heart failure, presence of hyperbilirubinemia (> 1.2 mg/dL), combined cardiac procedures (e.g., CABG plus valve), repeat cardiac operation, preoperative ejection fraction less than 40%, preoperative increases in partial thromboplastin time, and emergency operations; intraoperatively, prolonged CPB, use of TEE, and blood transfusion; and postoperatively, requirements for prolonged inotropic vasopressor support, IABP use for the treatment of low cardiac output; and prolonged ventilatory support are all risk factors. These factors identify patients at high risk, and they lend some credence to the overall pathophysiology and suspected causes of these adverse events. If there is a common link among all these risks, it is that many of these factors would be associated with impairment in oxygen delivery to the splanchnic bed.

Pathophysiology and Causative Factors
Impairments in splanchnic perfusion commonly occur during even the normal conduct of cardiac surgery. When these are superimposed on an already depressed preoperative cardiac output or associated with prolonged postoperative low cardiac output, the impairment in splanchnic blood flow is further perpetuated. The systemic inflammatory response to CPB itself can be initiated by splanchnic hypoperfusion by means of translocation of endotoxin from the gut into the circulation. De novo splanchnic hypoperfusion can be a result of the humoral vasoactive substances that are released by inflammation remote from the gut.538–540 Another causative factor for GI complications directly related to splanchnic hypoperfusion is atheroembolism. Several studies have directly attributed atheroembolism to splanchnic hypoperfusion and gut infarction.532,536,541 Prolonged ventilator support is another causative factor for GI complications. Several lines of investigation have described a relation between prolonged ventilation and GI adverse events; this likely results from a direct effect of positive-pressure ventilation impairing cardiac output and, subsequently, splanchnic perfusion.521,523,535,542 Lung volume reduction surgery and lung transplantation also produce (approximately 9%) GI complications.543,544

Protecting the Gastrointestinal Tract during Cardiac Surgery
As with other aspects of organ protection, critical causative factors need to be addressed with specific targeted therapies (Box 28-4). Unfortunately, as with most other organ-protective strategies, the major limitation in making definitive recommendations is an overall lack of large, well-controlled, prospectively randomized studies to provide supportive data for any one particular technique. However, some recommendations can be made, and attention can be focused on a number of other less well-studied but potentially valid strategies.
Cardiopulmonary Bypass Management
Because CPB itself has been shown to impair splanchnic blood flow, modifications in how it is conducted may have some salutary effects on GI tract integrity. Several studies have focused on the issue of the relative importance of pressure versus flow during CPB, demonstrating that it is likely more beneficial to maintain an adequate bypass flow rate than only maintaining pressure during bypass.545–547 The addition of significant vasoconstrictors to artificially maintain an adequate MAP in the presence of inadequate flow on CPB may lead to further compromise of splanchnic blood flow. Few definitive data offer guidance about pulsatility. Some studies have shown improvements with pulsatility by indirect measurements (i.e., gastric mucosal intracellular pH), but no studies have found definitive differences in clinical outcomes. Similarly, the optimal bypass temperature to protect the gut also is unknown. Just as aggressive rewarming can be injurious to the brain,63 there is some evidence that rewarming can cause increases in visceral metabolism, making any overshoot in temperature suspect by adversely altering the balance of gut oxygen consumption and delivery.548
Emboli Reduction
Whereas microembolization and macroembolization to the splanchnic bed clearly occur during CPB and possibly even during the postbypass period, there are few data to determine whether emboli reduction strategies can alter GI outcome. Mythen et al549 found a relation between transcranial Doppler–detected emboli (used as a surrogate for overall microembolic load within the body) and adverse changes in gastric mucosal intracellular pH. However, with respect to microembolization, a trial that used an intra-aortic filter to reduce atheroemboli failed to have any influence on the rate of GI complications.99 It remains prudent to avoid maneuvers (i.e., aortic cannulation and cross-clamping) in areas of high atheroma burden, which is an overall tenet of cardiac surgery for the prevention of all other complications.
Drugs
A range of vasoactive drugs has been used to enhance splanchnic blood flow during CPB. It is likely that most of these drugs, such as the phosphodiesterase III inhibitors, dobutamine, and other inotropic agents, maintain or enhance splanchnic blood flow not because of a direct effect on the vasculature but by the inherent enhancement in cardiac output. Dobutamine and dopamine have had a paradoxic detrimental effect on the splanchnic vasculature, with some evidence that they cause further mucosal ischemia.550,551 Dopexamine also has been studied in a small group that did show an improvement in mucosal blood flow (using laser–Doppler flowmetry), but there was no beneficial effect on gastric mucosal intracellular pH.552 An increasingly common drug in the setting of cardiac surgery is vasopressin. Although vasopressin clearly can augment systemic MAP, it does so at the cost of severe impairments to splanchnic blood flow.553 Although there are always trade-offs when choosing which vasoactive agent to use, if having a very low MAP is going to be detrimental to other organ systems, the choice to use vasopressin should at least be made with the knowledge that it can have an adverse effect on splanchnic blood flow.
Selective Gastrointestinal Decontamination
Addressing the concept that gut translocation of endotoxin plays a role in inflammation and other organ injuries, one interesting therapy that has been tried in the setting of cardiac surgery involves the administration of oral antibiotics to selectively decontaminate the GI tract before surgery.554 Although examined in a small (N = 100) study, a combination of oral polymyxin, tobramycin, and amphotericin before surgery for 3 days reduced the degree of endotoxemia, it was not associated with a beneficial effect on patient outcomes. One possible explanation is that there is such an overwhelmingly large depot of gram-negative organisms in the GI tract that the elimination of even a large population of these by oral antibiotics still leaves significant repositories of endotoxin in the gut. Killing these bacteria may in itself cause the release of the endotoxin, which can then be absorbed into the systemic circulation by impairment of mucosal blood flow inherent in the unphysiologic flow during CPB.
Off-Pump Cardiac Surgery
There is little evidence that the use of off-pump cardiac surgery is in any way beneficial to the GI tract. Three retrospective studies525,533,555 have shown no differences in GI complications. One reason for this lack of apparent difference between on-pump and off-pump cardiac surgery may again be related to the common denominator of splanchnic perfusion. OPCAB surgery is fraught with hemodynamic compromise that may lead to prolonged periods of splanchnic hypoperfusion by itself or as a result of the concurrent administration of vasopressors to maintain normal hemodynamics during the frequent manipulations of the heart.
Lung injury during cardiac surgery

Incidence and Significance
Pulmonary dysfunction was one of the earliest recognized complications of cardiac surgery using CPB.556 However, as improvements in operative technique and CPB perfusion technologies occurred, the overall frequency and severity of this complication decreased. Juxtaposed to the improvements in cardiac surgery, which led to an overall reduction in complications, is an evolving patient population that now comprises a higher risk group with a greater degree of pulmonary comorbidities, increasing their risks for postoperative pulmonary dysfunction. With the advent of fast-track techniques,557 even minor degrees of pulmonary dysfunction have reemerged as significant contributors to patient morbidity and the potential need for extended postoperative ventilation. As with most postoperative organ dysfunction, there is a range of dysfunction severity. Arguably, some degree of pulmonary dysfunction occurs in most patients after cardiac surgery; however, it manifests clinically only when the degree of dysfunction is particularly severe or the pulmonary reserve is significantly impaired.558,559 As a result, even minor CPB-related pulmonary dysfunction can cause significant problems in some patients.
Definitions have varied. One commonly accepted definition that was used in a large study (N = 1461 patients) performed in the setting of cardiac surgery defined acute pulmonary dysfunction as a patient requiring mechanical ventilation with a Pao2/Fio2 ratio of less than 150, irrespective of positive end-expiratory pressure, coupled with a chest radiograph that indicated the development of bilateral pulmonary infiltrates, assuming that other causes of hypoxia such as pneumothorax could be excluded. Using this definition, approximately 12% of patients on admission to the cardiovascular ICU after cardiac surgery met the criteria of early acute pulmonary dysfunction.560,561 Early acute pulmonary dysfunction should be differentiated from the more severe but less common ARDS that occurs in 1% to 2% of patients.560,562 Although these two forms likely represent similar processes with different degrees of severity, ARDS differs particularly in its timing. The definition of ARDS in the setting of cardiac surgery generally requires the presence of refractory hypoxemia, diffuse bilateral pulmonary infiltrates on chest radiograph, the requirement for mechanical ventilation with an Fio2 of more than 0.40, and most importantly, duration of at least 3 days (as opposed to on admission to the ICU). This must be in the presence of a low pulmonary capillary wedge pressure (< 18 mm Hg). In Welsby et al’s563 study of 2609 consecutive adult cardiac surgery patients, 7.5% of whom had pulmonary complications, the overall mortality rate was 21%, with 64% of these patients remaining in the hospital more than 10 days. In another study, the most significantly affected patients (i.e., those with ARDS) had a mortality rate upward of 80%.564 These significant morbidity and mortality rates lend credence to pulmonary complications remaining as significant and relevant today as in the early days of cardiac surgery.
Atelectasis and pleural effusions are the most common pulmonary abnormalities seen after cardiac surgery, presenting in more than 60% of patients.565,566 Atelectasis commonly is attributed to a number of intraoperative and postoperative events. With the induction of general anesthesia, physical compression of the left lower lobe to aid exposure of the heart and facilitate in the dissection of the internal mammary artery and the apnea occurring during the conduct of CPB itself have been implicated.567,568 Postoperative causes include the poor respiratory efforts by patients with impaired coughing, lack of deep inspirations, and pleural effusions.569 Despite a high incidence of these radiographically recognized complications, the clinical significance is relatively low.570,571
Pneumonia after cardiac surgery also has a variable incidence but a much greater significance to overall patient outcome. Reported rates of pneumonia range widely from 2% to 22%.572–575 Pneumonia occurring early after cardiac surgery portends a poor outcome, illustrated in one study by a mortality rate of 27%.576 A number of factors increase the risk for postoperative pneumonia, including smoking, the presence of chronic obstructive pulmonary disease, other pulmonary complications requiring prolonged intubation, significant cardiac failure itself, and the transfusion of large volumes of blood products.

Risk Factors for Pulmonary Dysfunction
Rady et al560 defined preoperative, operative, and postoperative variables that represent risk factors for early pulmonary dysfunction after cardiac surgery. Although it is not entirely clear whether any of these risk factors can be modified, identifying their presence increases the level of vigilance for the subsequent development of pulmonary dysfunction. The risk factors for the most severe ARDS syndrome as defined by Christenson et al562 include hypertension, current smoking, emergency surgery, congestive heart failure (New York Heart Association Class III and IV), low postoperative cardiac output, and a LV ejection fraction of less than 40%. More recently, Filsoufi et al reported the risk factors for respiratory failure, focusing on valve surgery patients.577 They studied 2808 patients having risk factors of preoperative renal failure, female sex, ejection fraction less than 30%, double-valve procedures, active endocarditis, advanced age (> 70 years), congestive heart failure, reoperation, emergent procedures, previous myocardial infarction, and prolonged (> 180 minutes) CPB time.

Pathophysiology and Causative Factors
Studies have demonstrated CPB-induced changes in the mechanical properties (i.e., elastance or compliance and resistance) of the pulmonary apparatus (particularly the lung as opposed to the chest wall) and changes in pulmonary capillary permeability. Impairment in gas exchange has been demonstrated to be a result of atelectasis with concomitant overall loss of lung volume.556,578–582 Most research has focused on the development of increases in pulmonary vascular permeability (leading to various degrees of pulmonary edema) as the principal cause of the impaired gas exchange that occurs during cardiac surgery and results in a high alveolar-arteriolar gradient (A-a Do2).
The cause of pulmonary dysfunction and ARDS after cardiac surgery is complex but largely revolves around the CPB-induced systemic inflammatory response with its associated increase in pulmonary endothelial permeability.583,584 A central causative theme is a significant upregulation in the inflammation induced because of the interaction between the blood and foreign surfaces of the heart-lung machine or the inflammation related to the consequences of splanchnic hypoperfusion with the subsequent translocation of significant amounts of endotoxin into the circulation. Endotoxin is proinflammatory, and it has direct effects on the pulmonary vasculature.585 Clinical studies have demonstrated an increase in circulating intracellular adhesion molecules after CPB in patients with development of acute lung injury.586 Pathologic examination of the lungs of patients manifesting ARDS has shown extensive injury to the tissue, including swelling and necrosis of endothelial cells and type I and II pneumocytes.587 In addition to CPB-mediated inflammation, inflammation mediated by endotoxemia has been reported. Several studies have identified transfusion of packed RBCs (> 4 units) as a risk factor for ARDS in the cardiac surgical patient.588,589 Transfusion-related ARDS has been repeatedly recorded in noncardiac surgical settings.

Pulmonary Thromboembolism
Although not an injury to the lungs occurring as a direct result of CPB itself, deep vein thrombosis (DVT) and pulmonary embolism occur with regular frequency in the cardiac surgical population. The incidence rate of pulmonary embolism after cardiac surgery ranges from 0.3% to 9.5%, with a mortality rate approaching 20%.326,590,591 The incidence of pulmonary embolism appears to be lower after valve surgery compared with CABG, which may be because of the anticoagulation that is started soon after valve surgery.591,592
The incidence rate of DVT is 17% to 46%, with most cases being asymptomatic.326,591 The greater incidences were reported from series that used lower extremity ultrasound to more comprehensively examine populations.326 DVT has been reported for the leg from which the saphenous vein grafts were harvested and for the contralateral leg.591,593 In a study of post-CABG patients (n = 270) admitted to a rehabilitation unit who underwent lower extremity ultrasound examinations, DVT was detected in 17%, with two patients subsequently experiencing development of pulmonary emboli.594 In a postmortem study in 147 patients after cardiac surgery, pulmonary embolism was the cause of death for 4% (see Chapter 24).
The recommendations for DVT prophylaxis in cardiac surgery are aspirin and elastic gradient compression stockings in patients who ambulate within 2 to 3 days after surgery, and low-molecular-weight heparin and sequential compression stockings in nonambulatory patients.326 These recommendations are based on a randomized trial in which prophylaxis with sequential pneumatic compression stockings provided no added protection against DVT in ambulating CABG patients treated with aspirin and elastic gradient-compression stockings.595

Pulmonary Protection
Ventilatory Strategies
Several studies have examined the use of continuous positive airway pressure during CPB as a means to minimize the decrement in the A-a Do2 gradient that can occur after surgery. In a small study by Gilbert et al,596 the effect of continuous positive airway pressure was examined in a randomized trial of 18 patients undergoing CABG surgery with CPB. Continuous positive airway pressure did not appear to make any difference with respect to changes in measured lung resistance and elastance. In a larger (N = 61) study by Berry et al,597 continuous positive airway pressure did appear to have some transient beneficial effects on A-a Do2; however, these minor differences dissipated 4 hours after bypass. Overall, it is unlikely that continuous positive airway pressure plays any major role in preventing or treating the pulmonary dysfunction that occurs in the setting of cardiac surgery.
The inspired oxygen content of the gases that the lungs see during the period of apnea during CPB may have an effect on the A-a Do2 gradient, probably because of the enhanced effect of greater Fio2 on the atelectasis (so-called absorption atelectasis). With these findings in mind, it would be prudent to reduce the Fio2 to room air levels during CPB. Several simple therapies can be introduced before separation from CPB, including adequate tracheobronchial toilet and the delivery of several vital capacity breaths that may reduce the amount of atelectasis that has occurred during CPB568 (Box 28-5; see Chapter 35).
Ventilatory support of patients with acute lung injury (including ARDS) has undergone changes.598,599 Although not studied specifically in the setting of cardiac surgery, a study authored by the Acute Respiratory Distress Syndrome network highlighted the avoidance of ventilator-associated pulmonary mechanotrauma.599 Delivery of repeated large tidal volumes may damage the alveoli and other small lung structures, and these mechanical stresses may activate a pulmonary inflammatory response with a local release of cytokines, further enhancing injury to the lungs. As a result, these groups of investigators randomized patients to small (approximately 6 mL/kg) or traditional tidal volumes (12 mL/kg). They demonstrated that the low tidal volume ventilation strategy could reduce mortality in patients with ARDS by up to 25%. It would be prudent to use this beneficial ventilator strategy in the cardiac surgical patient presenting with significant acute lung injury.
Pharmacologic Pulmonary Protection
Steroids
Anti-inflammatory therapies may play a role in moderating the effects of the more significant forms of pulmonary dysfunction that occur after cardiac surgery and that have inflammation as a central causative factor. However, with the exception of corticosteroids, few anti-inflammatory therapies are available for routine use. Corticosteroid use can reduce the amount of systemic inflammation as measured by circulating cytokines.600–602 However, this has not been coupled with a reduction in pulmonary dysfunction. Chaney et al, in two separate studies,187,603 demonstrated that relatively high doses of methylprednisolone actually had a detrimental effect on post-CPB pulmonary function. Both studies demonstrated no improvement or worsening in lung compliance, shunt, A-a Do2, and delays in extubation. It was speculated that the A-a Do2 deterioration and delayed pulmonary extubation associated with steroid administration were attributable to steroid-induced sodium retention and vasodilation resulting in increased shunt and increased lung water content with pulmonary edema. Steroid administration led to significant hyperglycemia, which is difficult to treat during CPB.603 In a similar study by Oliver et al602 comparing placebo with steroids or hemofiltration, the steroid-treated patients had larger increases in postoperative A-a Do2 gradients. Using a preset mechanical ventilation protocol to guide ventilation weaning, steroids again failed to reduce the time to tracheal extubation (519 ± 293 vs. 618 ± 405 minutes; P = 0.21), confirming Chaney et al’s603 findings.
Aprotinin
The nonspecific serum protease inhibitor aprotinin was used to reduce blood loss and transfusion after cardiac surgery, and there is some evidence that it reduced CPB-related systemic inflammation.604–606 Aprotinin was first investigated for use in the setting of cardiac surgery as a means to protect the lungs from the whole-body inflammatory response initiated as a result of the contact activation of blood with the foreign surfaces of the CPB apparatus.607,608 These studies serendipitously discovered aprotinin’s salutary effect on preventing blood loss and transfusion in cardiac surgery.609 After these studies, aprotinin was extensively evaluated for its blood loss and transfusion-sparing effects, with little further work focused on the pulmonary effects. Despite being a robust inhibitor of CPB-related inflammatory response, any ability to prevent the pulmonary complications of CPB has not yet been demonstrated.
Nitric Oxide
Consistent sequelae of pulmonary dysfunction include the development of variable degrees of increased pulmonary vascular resistance and pulmonary hypertension. As a result, several pulmonary vasodilators have been used, most notably inhaled NO, in an attempt to reduce the pulmonary artery pressures and with it the workload of the right ventricle.610–612
NO has been used in cardiac surgery and in heart and lung transplantation as a selective pulmonary vasodilator.613 However, no trials have demonstrated any beneficial use for its prophylactic administration in the setting of cardiac surgery. Although it has statistically significantly reduced pulmonary artery pressures in cardiac surgery, it is unclear whether these reductions are reflected in improvements in overall outcome.
Management of bypass: an overview

The Prebypass Period
An important objective is to prepare the patient properly for CPB (Box 28-6). This phase invariably involves two key steps: anticoagulation and vascular cannulation. With rare exception,614 heparin is still the anticoagulant clinically used for CPB. Dose, method of administration, and opinions as to what constitutes adequate anticoagulation vary. Heparin must be administered before cannulation for CPB, even if cannulation must be done emergently. Failure to do so is to risk thrombosis in both the patient and extracorporeal circuit. Chapters 30 and 31 offer a complete discussion of hemostasis management for the cardiac surgery patient. After heparin has been administered, a period of at least 3 minutes is customarily allowed for systemic circulation and onset of effect. An activated coagulation time or heparin concentration measurement demonstrating actual achievement of adequate anticoagulation is then performed.

Vascular Cannulation
The next major step in the prebypass phase is vascular cannulation. The goal of vascular cannulation is to provide access whereby the CPB pump may divert all systemic venous blood to the pump oxygenator at the lowest possible venous pressures and deliver oxygenated blood to the arterial circulation at pressure and flow sufficient to maintain systemic homeostasis (see Chapter 29).
Arterial Cannulation
Arterial cannulation generally is established before venous cannulation to allow volume resuscitation of the patient, should it be necessary. The ascending aorta is the preferred site for aortic cannulation because it is easily accessible, does not require an additional incision, accommodates a larger cannula to provide greater flow at a reduced pressure, and carries a lower risk for aortic dissection compared with other arterial cannulation sites (femoral or iliac arteries). Because hypertension increases the risk for aortic dissection during cannulation, the aortic pressure may be temporarily reduced (MAP < 70 mm Hg) during aortotomy and cannula insertion. Several potential complications are associated with aortic cannulation including embolization of air or atheromatous debris, inadvertent cannulation of aortic arch vessels, aortic dissection, and other vessel wall injury.615–620
Reviews and clinical reports emphasize the importance of embolization as the major mechanism of focal cerebral injury in cardiac surgery patients. Barzilai et al621 and Wareing et al616 reported intraoperative use of two-dimensional epiaortic ultrasound imaging as a guide to selection of cross-clamping and cannulation sites. In 24% and 33% of the patients in these studies, respectively, ultrasonic findings led to selection of alternate cannulation sites. A femoral or axillary artery, rather than the ascending aorta, can be cannulated for systemic perfusion. These alternate sites can be used when ascending aortic cannulation is considered relatively contraindicated, as in severe aortic atherosclerosis, aortic aneurysm or dissection, or known cystic medial necrosis.8,118,622,623 The anesthesiologist can look for evidence of cannula malposition by checking for unilateral blanching of the face, gently palpating carotid artery pulses for new unilateral diminution, and by measuring blood pressure in both arms to check for new asymmetries. However, robust assessments of CBFs can be made more reliably with the use of near-infrared spectroscopy cerebral oximetry (see Chapter 16).
Venous Cannulation
Venous cannulation can be achieved using a single atrial cannula that is inserted into the right atrium and directed inferiorly (Figure 28-11). Drainage holes in this multistage cannula are located in the inferior vena cava (IVC) and right atrium to drain blood returning from the lower extremities and the SVC and coronary sinus, respectively. This technique has the advantage of being simpler, faster, and requiring only one incision; however, the quality of drainage can be compromised easily when the heart is lifted for surgical exposure.624 The bicaval cannulation technique, required in cases in which right atrial access is necessary, involves cannulating the SVC and IVC (Figure 28-12). Loops placed around the vessels can be tightened to divert all caval blood flow away from the heart. Blood returning to the right atrium from the coronary sinus will not be drained using this technique, so an additional vent or atriotomy is necessary.
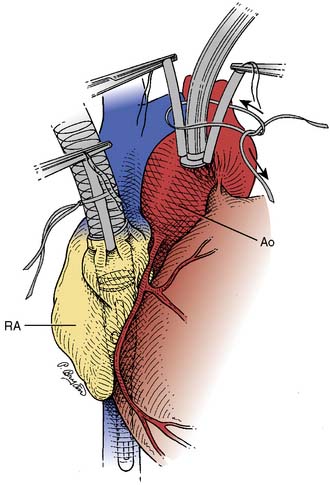
Figure 28-11 Aortic (Ao) and single, double-staged, right atrial (RA) cannulation.
Notice the drainage holes of venous cannula in right atrium and inferior vena cava.
(From Connolly MW: Cardiopulmonary Bypass. New York, Springer-Verlag, 1995, p 59.)
During CPB, blood will continue to return to the left ventricle from a variety of sources, including the bronchial and thebesian veins, as well as blood that traverses the pulmonary circulation. Abnormal sources of venous blood include a persistent left SVC, systemic-to-pulmonary shunts, and aortic regurgitation. It is important to avoid LV filling and distention during CPB to prevent myocardial rewarming, minimize LV wall tension, and limit myocardial oxygen demand.625 This can be accomplished with the use of a vent placed in the left ventricle via the left superior pulmonary vein. Alternate sites include the pulmonary artery, the aortic root, or directly into the left ventricle via the ventricular apex.

Other Preparations
Once anticoagulation and cannulation are complete, CPB can be instituted. Because there is redundant pulmonary artery catheter (PAC) length in the right ventricle, and the heart is manipulated during CPB, there is a tendency for distal migration of the catheter into pulmonary artery branches.626 This distal migration of the catheter increases the risks for “overwedging” and pulmonary artery damage. During the prebypass phase, it is advisable to withdraw the PAC 3 to 5 cm to decrease the likelihood of these untoward events. It also is advisable to check the integrity of all vascular access and monitoring devices. A PAC placed through an external jugular627,628 or subclavian vein629 can become kinked or occluded on full opening of the sternal retractor. If TEE is being used, the probe should be placed in the “freeze” mode and the tip of the scope placed in the neutral and unlocked position. Leaving the electronic scanning emitter turned on during hypothermic CPB adds heat (in older TEE equipment models) to the esophagus and posterior wall of the ventricle.
Before initiating CPB, the anesthesiologist should assess the depth of anesthesia and adequacy of muscle relaxation. It is important to maintain paralysis to prevent patient movement that could result in dislodgment of bypass circuit cannulae and prevent shivering as hypothermia is induced (with the attendant increases in oxygen consumption).630 It often is difficult to determine the depth of anesthesia during the various stages of CPB. Because blood pressure, heart rate, pupil diameter, and the autonomic nervous system are profoundly affected by ECC (e.g., the heart is asystolic; blood pressure is greatly influenced by circuit blood flow; sweating occurs with rewarming), these variables do not reliably reflect the anesthetic state. Although hypothermia decreases anesthetic requirements, it is necessary to provide analgesia, hypnosis, and muscle relaxation during CPB. Useful adjuncts to assessing depth of anesthesia are available in the form of processed EEG devices. For example, the bispectral index has proved useful in preventing awareness during cardiac surgery.631 With the initiation of CPB and hemodilution, blood levels of anesthetics and muscle relaxants will acutely decrease. However, plasma protein concentrations also decrease, which increases the free-fraction and active drug concentrations. Every drug has a specific kinetic profile during CPB, and kinetics and pharmacodynamics during CPB will vary greatly among patients. Many clinicians administer additional muscle relaxants and opioids at the initiation of CPB. A vaporizer for potent inhalation drugs may be included in the bypass circuit. Hall et al632 presented an extended discussion of pharmacokinetics and bypass. A final inspection of the head and neck for color, symmetry, adequacy of venous drainage (neck vein and conjunctiva engorgement), and pupil equality is reasonable to serve as a baseline for the anesthetic state. A summary of preparatory steps to be accomplished during the prebypass phase is given in Table 28-12.
Initiation and discontinuation of bypass support

Initiation of Cardiopulmonary Bypass
Uncomplicated Initiation
Once all preparatory steps have been taken, the perfusionist progressively increases delivery of oxygenated blood to the patient’s arterial system, as systemic venous blood is diverted from the patient’s right side of the heart, maintaining the pump’s venous reservoir volume. After full flow is achieved, all systemic venous blood is (ideally) drained from the patient to the pump reservoir. An on-CPB checklist of issues to address shortly after initiation of bypass can serve as a valuable safety tool (Table 28-13). The central venous pressure (CVP) and pulmonary arterial pressure should decrease to near zero (2 to 5 mm Hg), whereas systemic flow, arterial pressure, and oxygenation are maintained at desired values.
CVP, central venous pressure; PA, pulmonary artery; SVC, superior vena cava.
Hypotension with Onset of Bypass
Systemic arterial hypotension (MAP = 30 to 40 mm Hg) is relatively common on initiation of CPB. Gordon et al633 proposed that much of this could be explained by the acute reduction of blood viscosity that results from hemodilution with nonblood priming solutions. These investigators proposed systemic vascular resistance (SVR = MAP − CVP/CO) to be the product of blood viscosity (η) and inherent systemic vascular hindrance: SVR = η•SVH. MAP increases with initiation of hypothermia-induced vasoconstriction, together with levels of endogenous catecholamines and angiotensin. The hemodilution also results in the loss of NO binding by hemoglobin634; the excess free NO can lead to further vasodilation. Treatment with α-agonists usually is not necessary if the hypotension is brief (< 60 seconds). Of concern is the potential for myocardial and cerebral ischemia because hypothermia has not yet been achieved.
Until the aortic cross-clamp is applied, the coronary arteries are perfused with hemodiluted, nonpulsatile blood. Schaff et al635 showed that subendocardial ischemia occurred in the distribution of critical coronary stenosis when MAP was less than 80 mm Hg in the normothermic empty beating heart. If placement of the aortic cross-clamp is delayed, MAP should be maintained in the range of 60 to 80 mm Hg to support myocardial perfusion, especially in the presence of known coronary stenosis or ventricular hypertrophy. This arterial pressure is likely adequate to maintain CBF until hypothermia is induced.

Pump Flow and Pressure during Bypass
Pump flow during CPB represents a careful balance between the conflicting demands of surgical visualization and adequate oxygen delivery. Two different approaches exist. The first is to maintain oxygen delivery during ECC at normal levels for a given core temperature. Although this may limit hypoperfusion, it does increase the delivered embolic load. The second approach is to use the lowest flows that do not result in end-organ injury. This approach offers the potential advantage of less embolic delivery, as well as potentially improved myocardial protection and surgical visualization.636,637 However, some of these advantages are not seen when the left ventricle is vented during CPB.638
During CPB, pump flow and pressure are related through overall arterial impedance, a product of hemodilution, temperature, and arterial cross-sectional area. This is important because the first two factors, hemodilution and temperature, are critical determinants of pump flow requirements. Pump flows of 1.2 L/min/m2 perfuse most of the microcirculation when the hematocrit is near 22% and hypothermic CPB is being used.639 However, at lower hematocrits or periods of higher oxygen consumption, these flows become inadequate.640–642 Because of changes in oxygen demand with temperature and the plateauing of oxygen consumption with increasing flow, a series of nomograms have been developed for pump flow selection (Figure 28-13).