Chapter 6 Cardiology
Long Case
Cardiac disease
This chapter deals with problems that are likely to be discussion areas in the long-case section. Cardiac long-case patients may have complex cyanotic heart disease, or heart disease and other medical problems either causally related, as in Noonan syndrome or congenital rubella, or as a complication of their heart disease or its treatment (e.g. hemiparesis with cyanotic heart disease).
There have been many advances on several fronts in cardiology recently:
• Subacute bacterial endocarditis prophylaxis guidelines have been modified to reduce the number of cardiac conditions for which antibiotics are recommended with dental procedures (see below).
• Advances have been made in understanding the genetics and clinical aspects of the long QT syndrome, with risk stratification for experiencing a sudden life-threatening cardiac event (see below).
• Medical therapy for Marfan syndrome with angiotensin II receptor blockers (ARBs) has been shown to decrease the risk of aortic root dilatation, after a specific metabolic defect of the aortic wall was discovered that improved with ARBs (see below).
• Echocardiography has benefited from recent advances in computer technology, signal processing and high-frequency transducer design, to encompass new modalities: three-dimensional echocardiography (3DE), tissue Doppler imaging (TDI) and speckle tracking echocardiography (STE) are used to evaluate parameters including ventricular volume, myocardial velocity, left-ventricular twist/torsion (during systole) (STE), regional strain and strain rate (TDI and STE) and mechanical dyssynchrony (TDI, SDE, 3DE).
• Modification of cardiac risk factors is now actively occurring on children. In 2008, both the American Academy of Pediatrics and the American Heart Association reviewed obesity and the metabolic syndrome with insulin resistance, hypertension and early coronary artery disease, and now diet and drug therapy (including statins) are being recommended for children as young as 10 years.
History
Diagnosis
When made (birth, days, weeks or months); where; symptoms at diagnosis (e.g. cyanosis, tachypnoea, poor feeding, failure to thrive, recurrent infection); how (initial investigations: chest X-ray, electrocardiography, echocardiography, angiography).
Past history
1. Aetiology (e.g. rheumatic fever, pregnancy complicated by maternal rubella, smoking, alcoholism, or drugs such as lithium, warfarin or phenytoin).
2. Indications for, and number of, previous hospital admissions.
3. Pattern and timing of change in condition (e.g. when cyanosis or heart failure developed, when and how it was controlled).
4. Episodes of infective endocarditis.
5. Other complications of disease (e.g. cerebral thrombosis or cerebral abscess with cyanotic heart disease).
Treatment
1. Past surgery, complications thereof, plans for further operative procedures.
2. Past interventional catheter procedures.
3. Past ablation therapy, pacemaker or defibrillator placement.
4. Medications, past and present, the side effects of these, monitoring levels (e.g. digoxin), treatment plans for future.
5. Exercise restrictions (may be inappropriately applied by parents).
6. Recommendations for antibiotic prophylaxis for dental procedures; maintenance of dental hygiene.
8. Any need for identification bracelet.
9. Instructions for air travel and high altitude.
10. Any recent investigations monitoring treatment (e.g. Holter monitoring; oxygen saturation).
11. Any recent changes in treatment regimen, and indications for these.
Current state of health
• Symptoms of cardiac failure, such as fatigue and shortness of breath (in comparison with peers), cough, sweating, poor feeding, recurrent chest infections.
• Cyanosis, squatting with ‘Fallot turns’.
• Episodes suggestive of arrhythmias, such as syncope, alteration of consciousness, dizziness, shortness of breath, palpitations in older children (what was the rate; i.e. faster than when usually playing or excited), or a ‘funny feeling’ in the chest, sweating, nausea, vomiting, or the parents being unable to count the rapid pulse rate, suggesting a tachyarrhythmia.
• Chest pain (e.g. myocardial ischaemia versus musculoskeletal, pleural or pericardial causes) or headache (e.g. polycythaemia in cyanotic heart disease, severe hypertension, cerebral abscess).
• Recent change in condition. Note the temporal relationship between associated symptoms with any tachycardia or palpitation: they should coincide—functional chest pain can be followed by a reflex sinus tachycardia.
Other associated problems
These may either be related to the main diagnosis (e.g. Down syndrome, Marfan syndrome) or be more general problems (e.g. poor growth, developmental delay, especially involving gross motor abilities, exercise limitations and psychological effects).
Social history
1. Disease impact on child; for example, growth, development, schooling (academic performance, sports restrictions, teachers’ attitudes, peers’ attitudes, teasing, amount of school missed and whether schooling is appropriate).
2. Disease impact on parents; for example, financial situation, financial burden of disease so far, government allowances being received, marriage stability, restrictions on social life, plans for further children, genetic counselling, or at least an awareness of the risks of recurrence, and availability of fetal echocardiography.
3. Disease impact on siblings; for example, sibling rivalry, effect of family financial burden.
4. Social supports; for example, social worker, contact with other families of children with similar problems, break from managing child.
5. Coping: contingency plans (e.g. plan if child develops severe febrile illness); parents’ degree of understanding regarding cause, prognosis, exercise restriction, and antibiotic prophylaxis for operations and dental procedures.
6. Access to local doctor, paediatrician, cardiac outpatients clinic (where, how often), other clinics attended.
Management issues
1. General development, growth and nutrition
Chronic left-to-right shunts sufficient to cause cardiomegaly often cause growth retardation. This may be an indication for surgical correction even in the absence of other indications such as pulmonary hypertension. Marked hypoxia may be associated with growth retardation, but the hypoxia has to be severe to do so. Most patients who have Eisenmenger syndrome with cyanotic heart disease and pulmonary hypertension do not have increased energy requirements or inadequate caloric intake, and do not have growth retardation. It takes profound hypoxia to cause growth retardation.
2. Prophylaxis against subacute bacterial endocarditis (SBE) risk
Genitourinary and gastroenterological surgery
As enterococci are the usual problem, the recommended treatment should adequately cover these organisms; thus parenteral gentamicin and ampicillin is an appropriate choice.
4. Social issues
Impact of disease
Siblings receiving less parental attention than expected in a normal family is a commonly encountered problem.
Travel
All these children should carry a letter explaining the nature of their heart lesion, their usual medications, and the name of their doctor or usual treatment centre, and have access to a hospital if they have a disease prone to sudden deterioration (e.g. Fallot spells). An identification bracelet should also be worn. Those with pacemakers in particular should carry written details regarding their unit and its program. Patients with severe congestive cardiac failure, severe cyanosis or pulmonary hypertension must avoid high altitudes (e.g. above 1500 m) and require supplemental oxygen to be available during commercial air travel. Hot climates can be a problem in children with polycythaemia, and adequate hydration must be maintained. The child’s exercise tolerance must be considered when planning the trip itinerary.
5. Specific problems
Specific syndromes: cardiac involvement
Marfan syndrome
This is an autosomal dominant condition caused by defective fibrillin, a protein important to the integrity of connective tissue (see Figure 6.1). The relevant gene (FBN1) has been mapped to chromosome 15q21.1. The cardiac features are the most important and life-threatening aspects of Marfan syndrome, manifesting in childhood in 25% of those affected. The cardiac involvement is progressive in around one third of these children. Features include the major diagnostic criterion of dilatation of the ascending aorta with or without aortic regurgitation, and involving at least the sinuses of Valsalva or dissection of the ascending aorta. Minor diagnostic criteria for Marfan syndrome include mitral valve prolapse with or without mitral valve regurgitation, dilatation of the main pulmonary artery in the absence of another anatomic cause (before age 40), calcification of the mitral annulus (before age 40), and dilatation or dissection of the descending thoracic or abdominal aorta (before age 50).
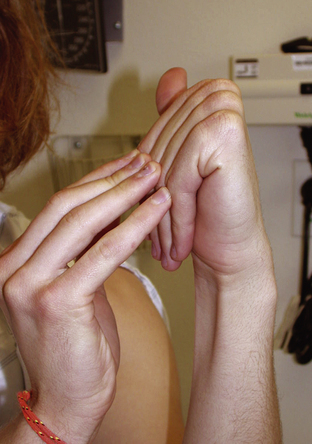
Figure 6.1 Marfan syndrome—joint hypermobility.
Jones, Kenneth. 2005. Smith’s Recognizable Patterns of Human Malformation, 6th edition, p. 550.
Parental education regarding the importance of avoiding strenuous exercise and competitive or contact sports is important, and should begin before preschool, placing less emphasis on the importance of sporting activities. Non-strenuous activities should be encouraged (e.g. walking, fishing, golf). The symptoms of aortic dissection must be discussed, including chest pain and syncope.
Dilatation or dissection of aorta at level of sinuses of Valsalva
Displacement of lens (ectopia lentis)—directed upward; also early cataract formation
Dolichostenomelia: disproportionately long extremities; decreased upper to lower segment ratio (US:LS < 0.85 for older children/adults) or arm span to height ratio > 1.05; other diagnostic skeletal features—
Distinctive facial features (dolichocephaly, down-slanting palpebral fissures, deeply set eyes (enophthalmos), decreased malar prominence (malar hypoplasia), diminished jaw (retrognathia)
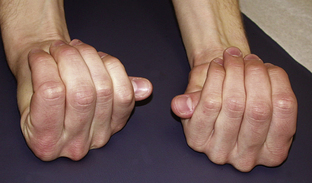
Figure 6.2 Steinberg thumb sign.
Jones, Kenneth. 2005. Smith’s Recognizable Patterns of Human Malformation, 6th edition, Figure 2B.
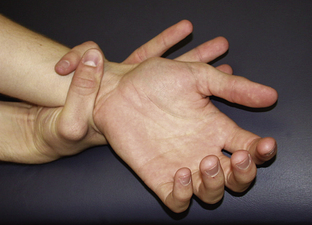
Figure 6.3 Walker–Murdoch wrist sign.
Jones, Kenneth. 2005. Smith’s Recognizable Patterns of Human Malformation, 6th edition, Figure 2C.
The acronym MARFANS also can be used as an (alternative) aide-mémoire:
M. Mitral prolapse ± Mitral regurgitation
A. Ascending aorta dilatation (involving sinuses of Valsalva)/dissection/Arched palate (high arched, with tooth crowding)/Acetabuli (protrusio acetabuli)
R. Regurgitation of aorta/mitral/Retinal detachment/Reduced US:LS ratio
F. Fibrillin defective/Facial gestalt (dolichocephaly, down-slanting palpebral fissures, deeply set eyes (enophthalmos), decreased malar prominence (malar hypoplasia), diminished jaw (retrognathia)/Flat cheek bones/Flat cornea/Flexible joints (joint hypermobility)
A. Apical blebs (on CXR)/Air leaks (spontaneous pneumothorax)/Arachnodactyly
Near-sightedness (myopia)/Neurological involvement related to dural ectasia—stretching of dural sac in the lumbosacral region/Nerve entrapment—CSF leak from dural sac (causing postural hypotension and headache)
S. Scoliosis/Sternal deformity/Skeletal dolichostenomelia/Sacral dural ectasia
Noonan syndrome (NS)
NS is an autosomal dominant condition, associated with four genes. In 50% of cases, the associated gene locus is at 12q24.1, with mutations in PTPN11, the gene encoding the non-receptor type protein, tyrosine phosphatase SHP-2. The other genes are KRAS, SOS and RAF1. Almost all patients with NS have some cardiac defect, particularly a dysplastic (and often stenotic) pulmonary valve, which is more common with a PTPN11 mutation, or hypertrophic cardiomyopathy (HCM), which affects around 20–30% of NS children, but is less common with a PTPN11 mutation. The ECG frequently shows left-axis deviation and a dominant S wave over the praecordial leads, even in NS patients with no known cardiac disease; the cause for this is not known. Phenotypic features of NS include dysmorphic facial features, short stature, webbed neck and skeletal anomalies (see the short case on dysmorphism).
NS patients with dysplastic pulmonary valves can have rapid progression of pulmonary valvular obstruction and may require review more frequently than for non-NS pulmonary valve lesions. Also, NS-associated valve obstruction is more likely to require surgical intervention. Balloon valvoplasty is usually unsuccessful in abolishing the obstruction, and simple valvotomy may be inadequate. Often complete excision of the valve, resection of the right-ventricular outflow muscle, and occasionally an outflow tract patch may be needed. Atrial septal defects (ASDs) (Figure 6.10) and pulmonary artery branch stenoses may coexist with valvular pulmonary stenosis (Figure 6.4). Other infrequent findings with NS include ventricular septal defects (VSDs) (Figure 6.12) and tetralogy of Fallot (Figure 6.17 and Figure 6.16).
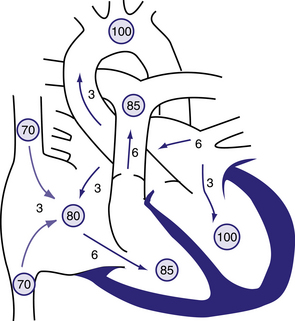
Figure 6.10 Physiology of atrial septal defect (ASD).
Circled numbers represent oxygen saturation values. The numbers next to the arrows represent volumes of blood flow (in L/min/m2). This illustration shows a hypothetical patient with a pulmonary-to-systemic blood flow ratio (Qp:Qs) of 2:1. Desaturated blood enters the right atrium from the vena cavae at a volume of 3 L/min/m2 and mixes with an additional 3 L of fully saturated blood shunting left to right across the ASD; the result is an increase in oxygen saturation in the right atrium. Six litres of blood flows through the tricuspid valve and causes a mid-diastolic flow rumble. Oxygen saturation may be slightly higher in the right ventricle because of incomplete mixing at the atrial level. The full 6 L flows across the right-ventricular outflow tract and causes a systolic ejection flow murmur. Six litres returns to the left atrium, with 3 L shunting left to right across the defect and 3 L crossing the mitral valve to be ejected by the left ventricle into the ascending aorta (normal cardiac output). Behrman et al, 2007. Nelson Textbook of Paediatrics, 17th edition, Figure 419.1.
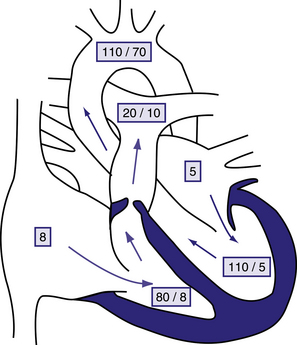
Figure 6.4 Physiology of valvular pulmonary stenosis.
Boxed numbers represent pressure in mmHg. Because of the absence of right-to-left or left-to-right shunting, blood flow through all cardiac chambers is normal at 3 L/min/m2. The pulmonary-to-systemic blood flow ratio (Qp:Qs) is 1:1. Right atrial pressure is increased slightly as a result of decreased right ventricular compliance. The right-ventricle is hypertrophied, and systolic and diastolic pressure is increased. The pressure gradient across the thickened pulmonary valve is 60 mmHg. The main pulmonary artery pressure is slightly low, and poststenotic dilatation is present. Left heart pressure is normal. Unless right-to-left shunting is occurring through a foramen ovale, the patient’s systemic oxygen saturation will be normal. Behrman et al, 2007. Nelson Textbook of Paediatrics, 17th edition, Figure 420.1.
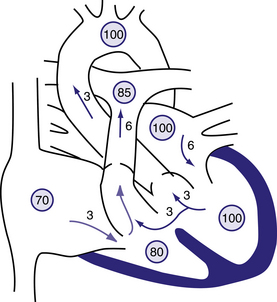
Figure 6.12 Physiology of a large ventricular septal defect (VSD).
Circled numbers represent oxygen saturation values. The numbers next to the arrows represent volumes of blood flow (in L/min/m2). This illustration shows a hypothetical patient with a pulmonary-to-systemic blood flow ratio (Qp:Qs) of 2:1. Desaturated blood enters the right atrium from the vena cava at a volume of 3 L/min/m2 and flows across the tricuspid valve. An additional 3 L of blood shunts left to right across the VSD, the result being an increase in oxygen saturation in the right ventricle. Six litres of blood is ejected into the lungs. Pulmonary arterial saturation may be further increased because of incomplete mixing at right-ventricular level. Six litres returns to the left atrium, crosses the mitral valve, and causes a middiastolic flow rumble. Three litres of this volume shunts left to right across the VSD, and 3 L is ejected into the ascending aorta (normal cardiac output). Behrman et al, 2007. Nelson Textbook of Paediatrics, 17th edition, Figure 419.5.
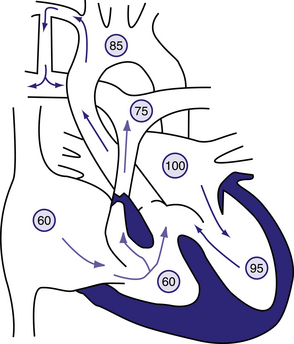
Figure 6.17 Blalock–Taussig shunt in the patient with tetralogy of Fallot.
Circled numbers represent oxygen saturation values. The intracardiac shunting pattern is as described for Figure 423.1. Blood shunting left to right across the shunt from the right subclavian artery to the right pulmonary artery increases total pulmonary blood flow and results in a higher oxygen saturation than would exist without the shunt (see Fig. 423.1). Behrman et al, 2007. Nelson Textbook of Paediatrics, 17th edition, Figure 423.5.
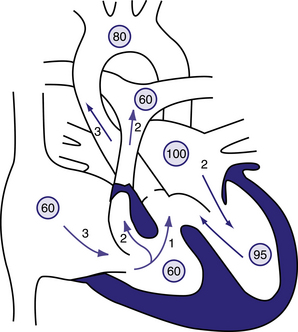
Figure 6.16 Physiology of the tetralogy of Fallot.
Circled numbers represent oxygen saturation values. The numbers next to the arrows represent volumes of blood flow (in L/min/m2). Atrial (mixed venous) oxygen saturation is decreased because of the systemic hypoxemia. A volume of 3 L/min/m2 of desaturated blood enters the right atrium and traverses the tricuspid valve. Two litres flows through the right-ventricular outflow tract into the lungs, whereas 1 L shunts right to left through the ventricular septal defect (VSD) into the ascending aorta. Thus, pulmonary blood flow is two thirds normal (Qp:Qs of 0.7:1). Blood returning to the left atrium is fully saturated. Only 2 L of blood flows across the mitral valve. Oxygen saturation in the left ventricle may be slightly decreased because of right-to-left shunting across the VSD. Two litres of saturated left-ventricular blood mixing with 1 L of desaturated right-ventricular blood is ejected into the ascending aorta. Aortic saturation is decreased, and cardiac output is normal. Behrman et al, 2007. Nelson Textbook of Paediatrics, 17th edition, Figure 423.1.
The acronym NOONANS can be used as an aide-mémoire:
Neurodevelopmental problems: 25% learning disability, 10–15% special education, <30% mild intellectual impairment, 72% articulation problems, non-verbal abilities better than verbal, specific learning problems/Neuromuscular examination—hypotonia (floppy strong): joint hyperextensibility/Neurosurgical—Arnold–Chiari type I
O. Obstructive heart lesions: RVOT (pulmonary valve); LVOT (HCM)/Other: ASD, VSD, tetralogy of Fallot, coarctation of aorta, branch pulmonary artery stenosis
O. Ocular anomalies in up to 95%: strabismus, refractive errors, amblyopia, nystagmus; hypertelorism, epicanthic folds, droopy eyelids, vivid blue or blue–green irises
Neoplasia risk: juvenile myelomonocytic leukaemia (JMML); myeloproliferative disorders; Noonan-like/multiple giant-cell lesion syndrome (granulomas and joint and bone anomalies); hepatosplenomegaly (related to subclinical myelodysplasia)
A. Abnormal coagulation (33% have one or more defects)/Abnormal lymphatics: lymphoedema (hands/feet/scrotum/vulva), lymphangiectasia (lung, gut or testis)
Neck webbing, with low-set posteriorly rotated ears/Nipples appear low-set/Nephrological anomalies: duplex collecting systems, renal hypoplasia