4 Cardiac Electrophysiology
Diagnosis and Treatment
Cardiac rhythm disturbances are common and an important source of morbidity and mortality.1,2 Supraventricular tachycardias (SVTs) have an estimated incidence from 35 per 100,000 person-years for paroxysmal SVT to 5 to 587 per 100,000 person-years for atrial flutter for individuals age 50 years versus those older than 80 years, respectively.3,4 But it is atrial fibrillation that has proved to be the most common sustained cardiac arrhythmia in the general population, affecting more than 2.3 million Americans.5 The prevalence of atrial fibrillation is strongly related to age, occurring in fewer than 1% of individuals younger than 55 years but in nearly 10% of those older than 80 years.5 The occurrence of atrial fibrillation increases health resource utilization, heightens the risk for stroke, and is associated with long-term mortality.6
There has been a major shift in the treatment of cardiac arrhythmias since the 1980s; this is due, in part, to advances made in catheter- and surgical-based ablations, as well as widely held views that pharmacologic treatments have limited efficacy and, in some instances, may actually increase risk for mortality. These latter observations are mostly due to the negative inotropic and proarrhythmic effects of these drugs.7,8 Data from prospective randomized trials showing improved survival for patients with implantable cardioverter-defibrillators (ICDs) compared with those given antiarrhythmic drugs have further contributed to a shift to nonpharmacologic treatments.9
Basic electrophysiologic principles

Anatomy and Physiology of the Cardiac Pacemaker and Conduction Systems
Sinus Node
The sinoatrial node (SAN; Figure 4-1) is a spindle-shaped structure composed of highly specialized cells located in the right atrial sulcus terminalis, lateral to the junction of the superior vena cava (SVC) and the right atrium10,11 (see Box 4-1 for a summary of the anatomy of the cardiac pacemaker and conduction system). Three cell types have been identified in the SAN (nodal, transitional, and atrial muscle cells), but no single cell appears to be solely responsible for initiating the pacemaker impulse. Rather, multiple cells in the SAN discharge synchronously through complex interactions.12–14 Rather than a discrete and isolated structure, studies suggest that the SAN consists of three distinct regions, each responsive to a separate group of neural and circulatory stimuli.15 The interrelationship of these three regions appears to determine the ultimate rate of output of the SAN. Although the SAN is the site of primary impulse formation, subsidiary atrial pacemakers located throughout the right and left atria also can initiate cardiac impulses.16–18 In a series of studies both in dogs and humans, it was confirmed that there is an extensive system of atrial pacemakers widely distributed in the right and left atria, as well as in the atrial septum.15,19–21 Because the atrial pacemaker system occupies a much larger area than the SAN, it can be severed during arrhythmia surgery, resulting in impaired rate responsiveness.10 However, it is extremely difficult to completely abolish SAN activity through catheter-based ablation techniques.
The arterial supply to the SAN (SAN artery) is provided from either the right coronary artery (RCA; in 60% of the population) or the left circumflex coronary artery (see Figure 4-1). The SAN is richly innervated with postganglionic adrenergic and cholinergic nerve terminals. Vagal stimulation, by releasing acetylcholine, slows SA nodal automaticity and prolongs intranodal conduction time, whereas adrenergic stimulation increases the discharge rate of the SAN.10
Internodal Conduction
For many years, there has been much controversy concerning the existence of specialized conduction pathways connecting the SAN to the atrioventricular (AV) node. Most electrophysiologists now agree that preferential conduction is unequivocally present, and that spread of activation from the SAN to the AV node follows distinct routes by necessity because of the peculiar geometry of the right atrium.10 The orifices of the superior and inferior cavae, the fossa ovalis, and the ostium of the coronary sinus divide the right atrium into muscle bands, thus limiting the number of routes available for internodal conduction (see Figure 4-1). These routes, however, do not represent discrete bundles of histologically specialized internodal tracts comparable with the ventricular bundle branches.22 It has been suggested that a parallel arrangement of myocardial cells in bundles, such as the crista terminalis and the limbus of the fossa ovalis, may account for preferential internodal conduction. Although electrical impulses travel more rapidly through these thick atrial muscle bundles, surgical transection will not block internodal conduction because alternate pathways of conduction through atrial muscle are available.23
Atrioventricular Junction and Intraventricular Conduction System
The AV junction (Figure 4-2) corresponds anatomically to a group of discrete specialized cells, morphologically distinct from working myocardium and divided into a transitional cell zone, compact portion, and penetrating AV bundle (bundle of His).24 Based on animal experiments, the transitional zone appears to connect atrial myocardium with the compact AV node.25 The compact portion of the AV node is located superficially, anterior to the ostium of the coronary sinus above the insertion of the septal leaflet of the tricuspid valve. The longitudinal segment of the compact AV node penetrates the central fibrous body and becomes the bundle of His. As the nodal-bundle axis descends into the ventricular musculature, it gradually becomes completely isolated by collagen and is no longer in contact with atrial fibers. The AV junction is contained within the triangle of Koch, an anatomically discrete region bounded by the tendon of Todaro, the tricuspid valve annulus, and the ostium of the coronary sinus (Figure 4-3). This triangle is avoided in all cardiac operative procedures to prevent surgical damage to AV conduction. Individual variability in the specific anatomy of the AV nodal area is dependent on the degree of central fibrous body development.10
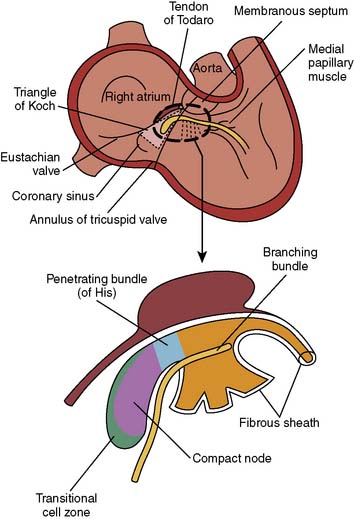
Figure 4-2 Anatomic relation of the atrioventricular junction in relation to other cardiac structures.
(From Harrison DC [ed]: Cardiac Arrhythmias: A Decade of Progress. Boston: GK Hall Medical Publishers, 1981.)
The branching of the nodal-bundle axis begins at the superior margin of the muscular interventricular septum. At this level, the bundle of His emits a broad band of fasciculi, forming the left bundle branch that extends downward as a continuous sheet into the left side of the septum beneath the noncoronary aortic cusp (see Figure 4-1). The left bundle divides into smaller anterior and broader posterior fascicles, although this is not a consistent anatomic delineation. The right bundle branch usually originates as the final continuation of the bundle of His, traveling subendocardially on the right side of the interventricular septum toward the apex of the right ventricle. The distal branches of the conduction system connect with an interweaving network of Purkinje fibers, expanding broadly on the endocardial surface of both ventricles. Blood supply to the AV node is mostly from the RCA (in 85% of the population) or from the left circumflex artery. The bundle of His is supplied by branches from the anterior and posterior descending coronary arteries. Innervation to the SA and AV nodes is complex because of substantial overlapping of vagal and sympathetic nerve branches. Stimulation of the right cervical vagus nerve causes sinus bradycardia, whereas stimulation of the left vagus produces prolongation of AV nodal conduction. Stimulation of the right stellate ganglion speeds SA nodal discharge rate, whereas stimulation of the left ganglion produces a shift in the pacemaker from the SAN to an ectopic site.26

Basic Arrhythmia Mechanisms
The mechanisms of cardiac arrhythmias are broadly classified as: (1) focal mechanisms that include automatic or triggered arrhythmias, or (2) reentrant arrhythmias (Box 4-2). Cells that display automaticity lack a true resting membrane potential and, instead, undergo slow depolarization during diastole (Figures 4-4 and 4-5). Diastolic depolarization results in the transmembrane potential becoming more positive between successive action potentials until the threshold potential is reached, leading to cellular excitation. Cells possessing normal automaticity can be found in the SAN, subsidiary atrial foci, AV node, and His-Purkinje system.10,13,27–29 The property of slow diastolic depolarization is termed spontaneous diastolic, or phase 4 depolarization. Factors that may modify spontaneous diastolic depolarization are shown in Figure 4-5 and include alterations in the maximum diastolic potential, threshold potential, and rate or slope of diastolic depolarization. The net effect of these factors is to influence the rate (increased or decreased) at which the threshold potential is achieved, resulting in either an increase or a decrease in automaticity. The ionic mechanism of diastolic depolarization involves the “funny” current, which, in turn, may involve a decrease in net outward K+ movement and/or an increase in net inward Na+ movement.26,30–33 Pacemaker cells with the fastest rate of phase 4 depolarization become dominant in initiating the cardiac impulse, with other automatic foci subject to overdrive suppression.
When cells that normally display automaticity (e.g., SAN, AV node, Purkinje fibers) change the rate of pacemaker firing, altered normal automaticity is said to occur. Although the ionic mechanisms resulting in altered normal automaticity are unchanged, other factors such as those seen in Figure 4-5 can contribute to an increase in automaticity. In contrast, automaticity resulting from abnormal ionic mechanisms, even if occurring in cells that are usually considered automatic (e.g., Purkinje fibers), is referred to as “abnormal automaticity.” Abnormal automaticity also may occur in cells in which automaticity is not normally observed (e.g., ventricular myocardium).
Arrhythmias arising from a “triggered” mechanism are initiated from cells that experience repetitive afterdepolarizations. Afterdepolarizations are oscillations in the transmembrane potential that occur either before (early afterdepolarizations) or after (delayed afterdepolarizations) membrane repolarization. Different ionic mechanisms are responsible for each form of afterdepolarization, and if the oscillations in membrane potential reach the threshold potential, a triggered cardiac impulse can be generated.13 Triggered activity is often considered an abnormal form of automaticity. However, because triggered activity requires a prior cardiac impulse (in contrast with automaticity), this abnormal electrophysiologic event cannot purely be considered a form of automaticity.
Reentry is a condition in which a cardiac impulse persists to re-excite myocardium that is no longer refractory.10 Unidirectional block of impulse conduction is a necessary condition for reentry. This unidirectional block may be in the form of differences in membrane refractoriness (dispersion of refractoriness) such that some areas of myocardium are unexcitable, whereas other areas allow impulse propagation. On repolarization, previously refractory membranes will be available for depolarization if the initial impulse has found an alternate route of propagation and returns to the prior site of conduction block. For reentry to occur, slowed conduction in the alternate pathway must exceed the refractory period of cells at the site of unidirectional block. Partial depolarization of fast-response fibers (depressed fast response) results in reduced Na+ channel availability with consequent reduced rate of phase 0 of the action potential. This reduced rate of action potential upstroke of phase 0 can result in slowed conduction and contribute to the above conditions conducive to reentry. Arrhythmias produced by reentrant or triggered mechanisms, but not those secondary to increased automaticity, can be induced with programmed stimulation in the setting of a diagnostic electrophysiology study (EPS). Pacemaker-induced overdrive suppression is a characteristic of arrhythmias produced by automaticity (see Chapter 25).

Diagnostic Evaluation
The history of symptoms often can provide clues in determining the cause of a patient’s palpitations. Abrupt onset and abrupt termination of regular palpitations, for example, are consistent with a paroxysmal SVT most often caused by atrioventricular nodal reentrant tachycardia (AVNRT), atrioventricular reentrant tachycardia (AVRT) associated with an accessory AV bypass tract, or atrial tachycardia. Although a history of syncope does not definitively point toward a ventricular or supraventricular cause, its presence is helpful in determining how urgently this condition should be evaluated. Whether palpitations are regular or irregular is useful in differentiating atrial fibrillation as a cause of the symptoms. Precipitating events, number and duration of episodes, presence of dyspnea, fatigue, or other constitutional symptoms should be sought from the history (Box 4-3).
A 12-lead electrocardiogram (ECG) should be obtained during tachycardia whenever possible and compared with baseline sinus rhythm ECGs. It also is helpful to run a rhythm strip during periods of intervention such as carotid sinus massage or adenosine administration. Patients with a history of pre-excitation presenting with an arrhythmia should be evaluated immediately because atrial fibrillation in the presence of an accessory pathway can lead to sudden death. For all patients undergoing evaluation of an arrhythmia, an echocardiogram is essential to evaluate for cardiac structural abnormalities and ventricular function. The latter is particularly germane for patients with persistent tachycardia because this can lead to tachycardia-associated cardiomyopathy.34 Twenty-four-hour Holter monitoring of patient-triggered events also may be useful in some patients with frequent but transient symptoms. Other evaluations such as exercise or pharmacologic stress testing also have been used to elicit episodes of tachycardia or determine how robust pre-excitation is present with increasing heart rates.
The ultimate diagnosis of the underlying mechanisms of the arrhythmia may require invasive electrophysiologic testing. These studies involve percutaneous introduction of catheters capable of electrical stimulation and recording of electrograms from various intra-cardiac sites. Initial recording sites often include the high right atrium, bundle of His, coronary sinus, and the right ventricle10,35 (Figures 4-6 and 4-7). The sequence of cardiac activation can be discerned from these recordings together with the surface ECG. This is illustrated in Figure 4-8 from a patient undergoing evaluation for an accessory AV conduction pathway. The sequence of the activation is observed by noting the timing of depolarization recorded by the respective electrodes positioned fluoroscopically at various anatomic sites. An example of a recording obtained during diagnostic evaluation of a patient with a ventricular arrhythmia is shown in Figure 4-9.
The catheters are most often introduced via the femoral vessels under local anesthesia. Systemic heparinization is required, particularly when catheters are introduced into the left atrium or left ventricle. The most common complications from electrophysiologic testing are those associated with vascular catheterization.10,36 Other complications include hypotension (in 1% of patients), hemorrhage, deep venous thrombosis (in 0.4% of patients), embolic phenomena (0.4%), infection (0.2%), and cardiac perforation (0.1%).10,37 Proper application of adhesive cardioversion electrodes before the procedure facilitates rapid cardioversion/defibrillation in the event of persistent or hemodynamically unstable tachyarrhythmia resulting from stimulation protocols.

Principles of Electrophysiologic Treatment
The paradigm for ablative treatment of cardiac arrhythmias evolved from the surgical treatment of Wolff–Parkinson–White (WPW) syndrome and then ventricular tachycardia (VT) developed by Sealy, Boineau, and colleagues.38–40 The fundamental paradigm for this approach is precise localization of the electrophysiologic substrate for the arrhythmia and then ablating the pathway. In the case of WPW syndrome, the accessory pathway is identified with intraoperative electrophysiologic mapping that initially used handheld electrodes.10,41 Development of multichannel computer-based mapping systems allowed for the identification of both the mechanisms for many arrhythmias, including VT, and their termination by interruption of the underlying substrate. Experience and insights into arrhythmia mechanisms led to the development of catheter-based methods now routinely used for a variety of supraventricular and ventricular arrhythmias. General indications for ablative treatments include drug-resistant arrhythmias, drug intolerance, severe symptoms, and desire to avoid lifelong drug treatments (Box 4-4).
Manipulation of catheter electrodes in the heart for precise mapping and treatment of arrhythmias can be laborious and time consuming. Newer catheters, as well as robotically and magnetically driven navigational systems, have been developed to facilitate this process and improve both catheter positioning and stability. With these navigational systems, the catheter tip is localized with three-dimensional fluoroscopy and/or advanced three-dimensional mapping applications and precisely moved to the myocardial area of interest using either a robotic arm or a magnetic field42 (Figure 4-10).
Specific Arrhythmias

Supraventricular Tachyarrhythmias
Supraventricular arrhythmias are defined as cardiac rhythms with a heart rate greater than 100 beats/min originating above the division of the common bundle of His. These arrhythmias are often seen as a narrow-complex tachycardia and, in some cases, can be hemodynamically unstable in the presence of structural heart disease. Further, persistent tachycardias for weeks to months may lead to tachycardia-associated cardiomyopathy and to disabling symptoms.34 The differential diagnosis of SVTs includes atrioventricular reciprocating tachycardia (AVRT), AVNRT, atrial tachycardia, inappropriate sinus tachycardia or sinus node reentry, atrial flutter, and atrial fibrillation. Antiarrhythmic medications traditionally have been used with mixed success. Hence surgical and catheter-based procedures have been developed for the management of these arrhythmias.

Atrioventricular Reciprocating Tachycardia
Accessory pathways are abnormal strands of myocardium connecting the atria and ventricles across the AV groove, providing alternate routes for conduction that bypass the AV node and bundle of His (Box 4-5). Various classifications are used to describe accessory pathways and are based on their location (e.g., tricuspid, mitral), whether they are manifest or concealed on a surface ECG, and the conduction properties exhibited by the pathway (e.g., antegrade, retrograde, decremental, nondecremental).42 Decremental conduction along any myocardial tissue refers to the concept that conduction through that tissue is slower as the frequency of impulses reaching it increases. Accessory pathways are more often nondecremental, meaning that regardless of how quickly impulses reach the pathway, the conduction velocity across the pathway remains the same. Concealed pathways refer to the situation in which the accessory pathway only exhibits retrograde conduction; thus, there is no conduction from the atrium to the ventricles through the pathway, thereby showing no evidence of ventricular pre-excitation. This is in contrast with manifest pathways displaying antegrade conduction from the atrium to the ventricles. Because electrical signals can enter the ventricles both from the AV node and the accessory pathway, ventricular pre-excitation will be “manifest” on the surface ECG as delta waves. Manifest pathways typically conduct in both antegrade and retrograde directions. The presence of a manifest pathway allows for the ventricle to be depolarized or “pre-excited” before that occurring via the normal route of conduction through the AV node (Figures 4-11 and 4-12). During pre-excitation, an activation wavefront propagates simultaneously to the ventricles across the bundle of His and the accessory pathway. Because anterograde conduction is delayed at the AV node but not the accessory pathway, the impulse passing through the accessory pathway initiates ventricular depolarization before the impulse traveling via the normal AV conduction system. The ventricle is thus pre-excited, resulting in a delta wave preceding the QRS complex (see Figure 4-11). These ECG findings (short PR interval and delta wave) were noted by Wolff, Parkinson, and White in the 1930s in association with SVT.44 WPW syndrome describes the condition of pre-excitation when accompanied by tachyarrhythmias caused by reentry via the accessory pathway. Not all individuals with the classic WPW ECG findings experience tachyarrhythmias. In fact, it is estimated that about 30% of individuals with WPW ECG findings exhibit tachyarrhythmias. Individuals with WPW ECG findings but without tachyarrhythmias are said to have the WPW signature. AVRT occurs in the absence of the WPW syndrome when the pathway is concealed, and not all tachyarrhythmias in patients with WPW result from the AVRT mechanism.
BOX 4-5. Atrioventricular Reciprocating Tachycardia Accessory Pathway Characteristics
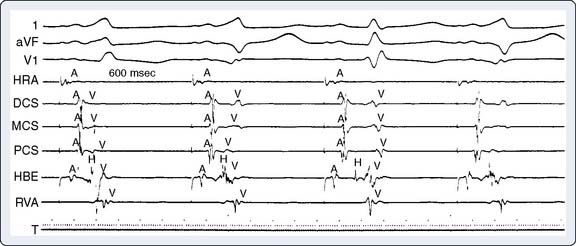
Figure 4-11 The presence of two accessory pathways is shown during pacing. The site of earliest ventricular activation is noted with the distal coronary sinus (DCS) electrode, indicating left free-wall accessory pathway. The second paced beat shows the site of earliest ventricular activation from the proximal coronary sinus (PCS) electrode, indicating posterior septal accessory pathway. After the third paced beat, neither site is activated due to anterograde conduction block. In this instance, conduction follows the normal AV-His bundle and bundle-branch pathways. Surface electrocardiogram leads and intracardiac electrograms are organized as in Figure 4-8. HBE, His-bundle region; HRA, high right atrium; MCA, mid-coronary sinus; RVA, right ventricular apex.
(From Cain ME, Cox JL: Surgical treatment of supraventricular tachyarrhythmias. In Platia EV [ed]: Management of Cardiac Arrhythmias. Philadelphia: JB Lippincott, 1987, p 312.)
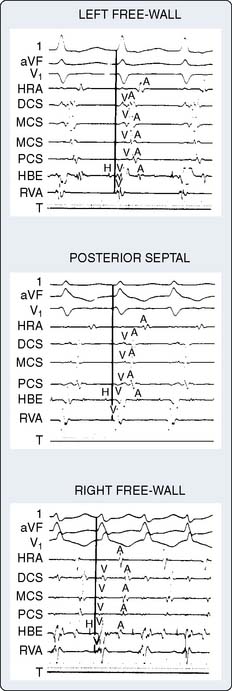
Figure 4-12 Atrial activation recordings from three different patients during orthodromic tachycardia via accessory pathways at distinct locations. Using the solid vertical line as a reference for the QRS complex from the surface electrocardiogram (ECG), the first example demonstrates the earliest atrial activation at the distal coronary sinus (DCS) site, indicating a left free-wall accessory pathway. The posterior septal accessory pathway is indicated by earliest activation of the electrode located in the proximal coronary sinus (PCS). In the last example, atrial activation at the high right atrium (HRA) and bundle of His area (HBE) occurs before all the coronary sinus recording sites, indicative of a right free-wall accessory pathway. Surface ECG leads and intracardiac electrograms are organized as in Figure 4-8. MCA, mid-coronary sinus; RVA, right ventricular apex.
(From Cain ME, Cox JL: Surgical treatment of supraventricular tachyarrhythmias. In Platia EV [ed]: Management of Cardiac Arrhythmias. Philadelphia: JB Lippincott, 1987, p 313.)
By noting polarity of the delta wave (QRS axis) and precordial R-wave progression, the resting 12-lead ECG can provide clues about the location of the accessory pathway in either the left lateral, left posterior, posterior septal, right free wall, or anterior septal regions45 (Table 4-1). Precise localization, though, is dependent on EPS. Additional information provided by such investigation includes documentation of the mechanism for the arrhythmia (AV vs. other mechanism) and the conduction properties of the accessory pathways. The atrial and ventricular insertion sites of the accessory pathway are identified by observing ventricular activation patterns during sinus rhythm and during atrial pacing (see Figure 4-11). In the presence of an accessory pathway, the interval between the deflection denoting activation of the bundle of His and the earliest ventricular activation (delta wave) is less than the H-V interval. The area with the shortest delta-to-V interval localizes the accessory pathway’s ventricular insertion. More than one accessory pathway may be present, which is suggested by observing different delta-wave morphology with increasing atrial pacing rates or with introduced atrial premature beats (see Figure 4-12). Observing atrial activation patterns during ventricular pacing, after a ventricular premature beat or during induced orthodromic SVT, can identify the location of the atrial insertion sites.
AVRT can occur in one of two fashions: orthodromic reciprocating tachycardia (ORT) and antidromic reciprocating tachycardia (ART)46–48 (Figure 4-13). ORT is by far the most common type and involves antegrade conduction via the normal AV nodal conduction system and retrograde conduction via the accessory pathway. ART, in contrast, involves antegrade conduction down the accessory pathway and retrograde conduction via the AV node. As suggested by these mechanisms, ORT appears as a narrow-complex tachycardia, whereas ART appears as a wide-complex tachycardia that at times can be difficult to distinguish from VT. Importantly, atrial fibrillation occurring in patients with a pathway capable of conducting in an antegrade fashion run the risk for rapid conduction to the ventricles and development of ventricular fibrillation and sudden death. The potential for sudden death caused by atrial fibrillation in patients with WPW provides an argument for aggressive ablative treatment when the procedure can be performed in centers with low periprocedural morbidity.

Catheter-Based Therapy for Accessory Pathways
Percutaneous catheter ablation of accessory pathways has largely supplanted the surgical approach to treatment. RF ablation is typically performed during EPS once the accessory pathway has been localized. Transseptal or retrograde aortic catheter approaches are used to ablate left-sided accessory pathways, and right-heart catheterization via a venous approach is used to ablate right-sided pathways. Success rates of 95% have been reported using these methods.43,49–51 Recurrence rates after successful catheter ablation of an accessory pathway are generally less than 5% and are a function of pathway location, as well as stability of the catheter during energy delivery. Overall, reported complications are low and include those related to vascular access such as hematoma and AV fistula. Other complications are related to catheter manipulations of the left- and right-sided circulation such as valvular or cardiac damage from the catheter, systemic and cerebral embolization caused by catheter manipulation in the aorta, coronary sinus damage, coronary thrombosis and dissection, cardiac perforation, and cardiac tamponade. Complete AV block, cardiac perforation, and coronary spasm caused by RF also may occur. A 1995 survey involving 5427 patients reported serious complications from catheter ablation of accessory pathways in 1.8% of patients and procedure-related mortality in 0.08%.43,49 Complete AV block is more common with ablation of accessory pathways close to the bundle of His. Procedural success with catheter ablation methods is reported to be 87% to 99%.43,50,51 In a randomized study comparing ablation with drug treatment, quality of life, symptom scores, and exercise performance were improved with successful RF ablation.52

Atrioventricular Nodal Reentrant Tachycardia
AVNRT is due to altered electrophysiologic properties of the anterior fast pathway and posterior slow pathway fibers providing input to the AV node.10,43,51 In the past, the only treatment for recurrent SVT caused by AVNRT was total ablation of the His bundle and permanent pacemaker insertion. Surgical techniques developed in the 1980s provided an alternate treatment that was associated with high procedural success, acceptable morbidity, and preservation of AV conduction.53–56 Fundamentals developed with this surgical approach and increased understanding of the physiologic basis of AVNRT led to the development of percutaneous catheter-based treatments. Interruption of either the slow or fast pathway with RF ablation can eliminate AVNRT, with greater success rates reported for ablation of the slow pathway (slow-pathway ablation [68% to 100%] vs. fast-pathway ablation [46% to 94%]).43,57–60 Complication rates are lower with slow-pathway RF ablation and include AV block requiring pacemaker insertion (1%)43 (Box 4-6).
BOX 4-6. Atrioventricular Nodal Reentrant Tachycardia
Catheter-Based Therapy for Atrioventricular Nodal Reentrant Tachycardia
Historically, fast-pathway ablation is performed by positioning the catheter adjacent to the AV node–His bundle anterosuperior to the tricuspid valve annulus. The catheter is withdrawn until the atrial electrogram is larger than the ventricular electrogram and the His recording small or absent. The ECG is closely monitored as RF energy is applied for PR prolongation/heart block. The energy is delivered until there is PR prolongation or the retrograde fast-pathway conduction is eliminated. Noninducibility of AVNRT then is confirmed. Given the increased incidence of complete heart block with fast-pathway ablation, most electrophysiologists have adopted ablation of the slow pathway as a safer alternative. Slow-pathway ablation is performed by identifying the pathway along the posteromedial tricuspid annulus near the coronary sinus. One approach using fluoroscopy is to divide the level of the coronary sinus os and His bundle recordings into six anatomic regions61 (Figure 4-14). Lesions then are placed beginning with the most posterior region moving anteriorly. Rather than the anatomic approach, the slow pathway can be mapped and then ablated by performing ventricular pacing. The end point of slow-pathway ablation is elimination of induced AVNRT.43,57–60 The development of junctional ectopy during RF ablation of the slow pathway is associated with successful slow-pathway ablation.10