Chapter 25 Cardiac arrhythmia
Some physiology and pathophysiology
There are broadly two types of cardiac tissue.
Ionic movements into and out of cardiac cells
In the resting state the interior of the cell (conducting and contracting types) is electrically negative with respect to the exterior, owing to the disposition of ions (mainly sodium, potassium and calcium) across its membrane, i.e. it is polarised. The ionic changes of the action potential first result in a rapid redistribution of ions such that the potential alters to positive within the cell (depolarisation); subsequent and slower flows of ions then restore the resting potential (repolarisation). These ionic movements separate into phases, which are briefly described here and in Figure 25.1, as they help to explain the actions of antiarrhythmic drugs.1
Classification of antiarrhythmic drugs
The classification still used partially relates to the phases of the cardiac cycle depicted in Figure 25.1.
Phase 3 is a second period of rapid repolarisation during which potassium ions move out of the cell.
Most cardiac arrhythmias are due to either:
• slowed conduction in part of the system leading to the formation of re-entry circuits (more than 90% of tachycardias), or
• altered rate of spontaneous discharge in conducting tissue. Some ectopic pacemakers appear to depend on adrenergic drive.
Classification of drugs
The Vaughan–Williams2 classification of antiarrhythmic drugs is still commonly used despite its many peculiarities, and on occasion provides a useful shorthand for referring to particular groups or actions of drugs.
Class I: sodium channel blockade
1A. lengthen action potential duration and refractoriness (adjunctive class III action), e.g. quinidine, disopyramide, procainamide
1B. shorten action potential duration and refractoriness, e.g. lidocaine and mexiletine
1C. have negligible effect on action potential duration and refractoriness, e.g. flecainide, propafenone.
Principal drugs by class
For further data see Table 25.1.
Class 1A (sodium channel blockade with lengthened refractoriness)
Quinidine
Quinidine is considered the prototype antiarrhythmic drug,3 although it is now used quite rarely and indeed is not available in some jurisdictions. It has a newly identified use that is unique in that it appears to be effective in reducing the risks of sudden cardiac death in those with Brugada syndrome.4 In addition to its class IA activity, quinidine slightly enhances contractility of the myocardium (positive inotropic effect) and reduces vagus nerve activity on the heart (antimuscarinic effect).
Adverse reactions
Quinidine must not be used alone to treat atrial fibrillation or flutter as its antimuscarinic action enhances AV conduction and the heart rate may accelerate. Other cardiac effects include serious ventricular tachyarrhythmias associated with electrocardiographic QT prolongation, i.e. torsade de pointes (Fig. 25.2), the cause of ‘quinidine syncope’. Non-cardiac effects, called ‘cinchonism’, include diarrhoea and other gastrointestinal symptoms, rashes, thromobocytopenia and fever, and these have substantially limited its use.
Class IB (sodium channel blockade with shortened refractoriness)
Class IC (sodium channel blockade with minimal effect on refractoriness)
Flecainide
One common indication – indeed where it is the drug of choice – is atrioventricular (AV) re-entrant tachycardia, such as AV nodal tachycardia or in the tachycardias associated with the WPW syndrome or similar conditions with anomalous pathways. This should be as a prelude to definitive treatment with radiofrequency ablation, which is the overall treatment approach of choice. Flecainide is also very useful in patients with paroxysmal atrial fibrillation, used in conjunction with an agent that blocks the AV node to protect against rapid conduction to the ventricle. Following the salutary findings of the CAST study,5 flecainide is now restricted to patients without evidence of coronary or structural heart disease. Indeed before it is used an echocardiogram is essential, and in patients at potential risk of coronary artery disease an exercise test or an alternative test of ischaemia is often conducted.
Class II (catecholamine blockade)
β-Adrenoceptor antagonists
• The rate of automatic firing of the SA node is accelerated by β-adrenoceptor activation and this effect is abolished by β-blockers. Some ectopic pacemakers appear to be dependent on adrenergic drive.
• β-blockers prolong the refractoriness of the AV node, which may prevent re-entrant tachycardias that are dependent on the AV node for their perpetuation.
• Many β-blocking drugs (propranolol, oxprenolol, acebutolol, labetalol) also possess membrane stabilising (class I) properties. Sotalol also prolongs cardiac refractoriness (class III effect) but has no class I effects; it is often preferred when a β-blocker is indicated for arrhythmias, but should be used with care. Esmolol (below) is a short-acting β1-selective agent, whose sole use is in the treatment of arrhythmias. Its short duration and β1 selectivity make it an option for some patients with contraindications to other β-blocking drugs.
• β-Adrenoceptor antagonists are effective for a range of supraventricular arrhythmias, in particular those associated with exercise, emotion or hyperthyroidism. Sotalol finds use to suppress ventricular ectopic beats and ventricular tachycardia although care should be taken with careful monitoring of the QT interval whenever it is used.
Pharmacokinetics
For long-term use, any of the oral preparations of β-blocker are suitable. In emergencies, esmolol is used (see Table 25.1), its short t½ (9 min) rendering it suitable for administration by intravenous infusion with rapid alterations in dose, according to response.
Class III (lengthening of refractoriness due to potassium channel blockade)
Amiodarone
Pharmacokinetics
Amiodarone is effective given orally; its enormous apparent distribution volume (70 L/kg) indicates that little remains in the blood. It is stored in fat and many other tissues and the t½ of 54 days after multiple dosing signifies slow release from these sites (and slow accumulation to steady state means that a loading dose is necessary; see Table 25.1). The drug is metabolised in the liver and eliminated through the biliary and intestinal tracts.
Adverse reactions
Cardiovascular effects include bradycardia, heart block and induction of ventricular arrhythmia associated with QT prolongation. Other effects include nausea, vomiting, taste disturbances and the development of corneal microdeposits, which may rarely cause visual halos, night glare and photophobia; the latter are dose related, resolve on discontinuation and do not threaten vision. Sleep disturbance and vivid dreams may be prominent and problematic. Plasma transaminase levels may rise (requiring dose reduction or withdrawal if accompanied by acute liver disorders). Amiodarone contains iodine, and both hyperthyroidism and hypothyroidism are quite common; monitoring thyroid function before and during therapy is essential (see chapter 37).
Dronedarone
Dronedarone has been shown to reduce the time to first recurrence of atrial fibrillation. In the EURIDIS and ADONIS clinical trials,6 patients taking dronedarone had a 25% reduction in the risk of AF recurrence over one year, compared with placebo. In the ATHENA study,7 dronedarone also reduced the combined risk of cardiovascular hospitalisation or all-cause death by 24% in patients with current or recent AF and an additional risk factor for death, compared with placebo. A post hoc analysis found that dronedarone, compared with placebo, was associated with a significant reduction in the risk of stroke in paroxysmal and persistent AF patients. Data from the DIONYSOS trial8 suggest that dronedarone may have an improved safety profile when compared to amiodarone, mainly driven by fewer thyroid and neurologic events and less premature discontinuation due to adverse events.
Class IV (calcium channel blockade)
Calcium is involved in the contraction of cardiac and vascular smooth muscle cells, and in the automaticity of cardiac pacemaker cells. Actions of calcium channel blockers on vascular smooth muscle cells appear with the main account of these drugs in Chapter 24. Although the three classes of calcium channel blocker have similar effects on vascular smooth muscle in the arterial tree, their cardiac actions differ. The phenylalkylamine, verapamil, depresses myocardial contraction more than the others, and both verapamil and diltiazem slow conduction in the AV node.
Calcium and cardiac cells
Cardiac muscle cells are normally depolarised by the fast inward flow of sodium ions, following which there is a slow inward flow of calcium ions through the L-type calcium channels (phase 2 in Figure 25.1); the consequent rise in free intracellular calcium ions activates the contractile mechanism.
Verapamil
Verapamil (see also p. 397) prolongs conduction and refractoriness in the AV node and depresses the rate of discharge of the SA node. If adenosine is not available, verapamil is a very attractive and, with due care, safe alternative for terminating narrow complex paroxysmal supraventricular tachycardia. Verapamil should not be given intravenously to patients with broad complex tachyarrhythmias whatever the presumptive mechanism, for it may prove lethal.
Other antiarrhythmics
Digoxin and other cardiac glycosides9
Uses
• Atrial fibrillation, benefiting chiefly by the vagal effect on the AV node, reducing conduction through it and thus slowing the ventricular rate. Its use in this setting is limited and treatment with β-blockers and calcium channel blockers is generally preferred as they are more effective and less likely to cause adverse effects.
• Atrial flutter, benefiting by the vagus nerve action of shortening the refractory period of the atrial muscle so that flutter may occasionally be converted to fibrillation (in which state the ventricular rate is more readily controlled). Electrical cardioversion followed by referral for radiofrequency ablation is the preferred option when ablation is available.
• Heart failure, benefiting chiefly by the direct action to increase myocardial contractility. Digoxin is still used occasionally in chronic heart failure due to ischaemic, hypertensive or valvular heart disease, especially in the short term. This is no longer a major indication following the introduction of other groups of drugs.
Dose and therapeutic plasma concentration
(See Table 25.1.) Reduced dose of digoxin is necessary in: renal impairment (see above); the elderly (probably due to the decline in renal clearance with age); electrolyte disturbances (hypokalaemia accentuates the potential for adverse effects of digoxin, as does hypomagnesaemia); hypothyroid patients (who are intolerant of digoxin).
Adverse effects
Acute digoxin poisoning causes initial nausea and vomiting and hyperkalaemia because inhibition of the Na+, K+-ATPase pump prevents intracellular accumulation of potassium. The ECG changes (see Table 25.1) of prolonged use of digoxin may be absent. There may be exaggerated sinus arrhythmia, bradycardia and ectopic rhythms with or without heart block.
Cardiac effects of the autonomic nervous system
Proarrhythmic drug effects
All antiarrhythmic drugs can also cause arrhythmia; they should be used with care and almost invariably following advice from a specialist (heart rhythm specialist/electrophysiologist). Such proarrhythmic effects most commonly occur with drugs that prolong the QTc interval or QRS complex of the ECG; hypokalaemia aggravates the danger. Quinidine may cause tachyarrhythmias, torsade de pointes (see Fig. 25.2) in an estimated 4–6% of patients. The Cardiac Arrhythmia Suppression Trial (CAST) revealed a probable pro-arrhythmic effect of flecainide resulting in fatalities (see p. 432). Digoxin can induce a variety of bradyarrhythmias and tachyarrhythmias (see above).
Choice between drugs and electroconversion
Electrical conversion has the advantage that it is immediate, unlike drugs, which may take days or longer to act; also, the effective doses and adverse effects of drugs are largely unpredictable, and can be serious.10
Uses of electrical conversion: in supraventricular and ventricular tachycardia, ventricular fibrillation and atrial fibrillation and flutter. Drugs can be useful to prevent a relapse, e.g. sotalol, amiodarone. See also the UK Resuscitation Council’s guidelines (Figs 25.3–25.5).
Specific treatments11
Atrial fibrillation (AF)
What management options are available?
The information that should be considered is extensive and includes:
In patients who have reverted to AF after previous conversions, amiodarone is the drug of choice prior to further attempts at cardioversion. Amiodarone may also be used to suppress episodes of paroxysmal atrial fibrillation, but dronedarone, sotalol or flecainide are preferred12 (Fig. 25.6). Radiofrequency ablation is now established as the treatment of choice in many patients with both paroxysmal and persistent atrial fibrillation and patients with symptomatic atrial fibrillation should ideally be referred to heart rhythm specialists for advice on further management.
Ventricular fibrillation and cardiac arrest
Ventricular fibrillation is usually caused by myocardial infarction or ischaemia, or serious organic heart disease and is the main cause of cardiac arrest. Guidelines for the management of peri-arrest arrhythmias and cardiac arrest are issued by the UK Resuscitation Council and appear in Figures 25.3, 25.4 and 25.5. Patients suffering ‘failed’ sudden cardiac death (SCD) should be considered for the insertion of an ICD.
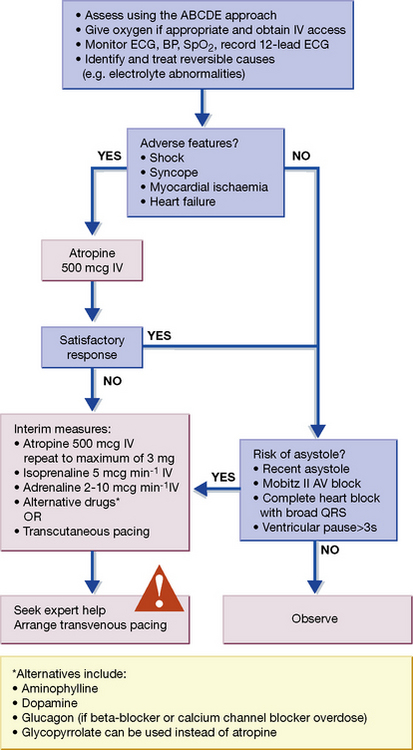
Fig. 25.3 Algorithm for the management of acute bradycardia. mcg, micrograms. Reproduced with the kind permission of the Resuscitation Council (UK). The latest version can be found at: http://www.resus.org.uk
Long QT syndromes
These are caused by malfunction of ion channels, leading to impaired ventricular repolarisation (expressed as prolongation of the QT interval) and a characteristic ventricular tachycardia, torsade de pointes (see Fig. 25.2).13 The symptoms range from episodes of syncope to cardiac arrest. Several drugs are responsible for the acquired form of the condition including antiarrhythmic drugs (see above), antimicrobials, histamine H1-receptor antagonists and serotonin receptor antagonists; predisposing factors are female sex, recent heart rate slowing, and hypokalaemia.14 Congenital forms of the long QT syndrome are due to mutations of the genes encoding ion channels, and exposure to drugs reveals some of these.
• The treatment of cardiac arrhythmias has advanced enormously and can be directly physical, electrical, pharmacological or surgical. Radiofrequency ablation and devices such as permanent pacemakers and ICDs increasingly provide the preferred approaches. The use of drugs alone is declining but they often constitute adjunctive treatments.
• The choice among drugs follows partly from theoretical predictions of their action on the cardiac cycle but substantially from short- and long-term observations of their efficacy and safety.
• All antiarrhythmics can be potentially dangerous, and should be used only in patients who have been properly and fully assessed.
• Adenosine is the treatment of choice for diagnosis and reversal of supraventricular arrhythmias. Verapamil is an alternative for the management of narrow complex tachycardias.
• Amiodarone is the most effective drug for reversing and preventing atrial fibrillation, and for preventing ventricular arrhythmias, but it has notable adverse effects. Dronedarone has been designed to provide the actions of amiodarone without the side-effects but is not so effective.
• New drugs are needed and several are in the pipeline.
• In view of the increasing complexity and range of treatment options all patients should be considered for referral to a heart rhythm specialist for a full discussion of the options available.
Camm A.J., Kirchhof P., Lip G.Y., et al. Guidelines for the management of atrial fibrillation: the Task Force for the Management of Atrial Fibrillation of the European Society of Cardiology (ESC). Eur. Heart J.. 2010;31:2369–2429.
Crystal E., Connolly S.J. Role of oral anticoagulation in management of atrial fibrillation. Heart. 2004;90:813–817.
Delacretaz E. Clinical practice. Supraventricular tachycardia. N. Engl. J. Med.. 2006;354:1039–1051.
Dobrev D., Nattel S. New antiarrhythmic drugs for treatment of atrial fibrillation. Lancet. 2010;375:1212–1223.
Lip G.Y., Halperin J.L. Improving stroke risk stratification in atrial fibrillation. Am. J. Med.. 2010;123:484–488.
Morady F. Catheter ablation of supraventricular arrhythmias: state of the art. J. Cardiovasc. Electrophysiol.. 2004;15(1):124–139.
Page R.L., Roden D.M. Drug therapy for atrial fibrillation: where do we go from here? Nat. Rev. Drug. Discov.. 2005;4(11):899–910.
Torp-Pedersen C., Pedersen O.D., Kober L. Antiarrhythmic drugs: safety first. J. Am. Coll. Cardiol.. 2010;55:1577–1579.
Zimetbaum P. Amiodarone for atrial fibrillation. N. Engl. J. Med.. 2007;356:935–941.
1 Dobrev D, Nattel S 2010 New antiarrhythmic drugs for treatment of atrial fibrillation. Lancet 375:1212–1223.
2 Roden D M 2003 Antiarrhythmic drugs: past, present and future. Journal of Cardiovascular Electrophysiology 14:1389–1396.
3 In 1912, K F Wenckebach, a Dutch physician (who described ‘Wenckebach block’) was visited by a merchant who wished to get rid of an attack of atrial fibrillation (he had recurrent attacks which, although they did not unduly inconvenience him, offended his notions of good order in life’s affairs). On receiving a guarded prognosis, the merchant inquired why there were heart specialists if they could not accomplish what he himself had already achieved. In the face of Wenckebach’s incredulity he promised to return the next day with a regular pulse, which he did, at the same time revealing that he had done it with quinine (an optical isomer of quinidine). Examination of quinine derivatives led to the introduction of quinidine in 1918 (Wenckebach K F 1923 Journal of the American Medical Association 81:472).
4 An inherited condition that is the major cause of sudden unexpected death syndrome (SUDS), commonly in young men.
5 Flecainide, encainide and moricizine underwent clinical trial to establish whether suppression of asymptomatic premature beats with antiarrhythmic drugs would reduce the risk of death from arrhythmia after myocardial infarction. The study was terminated after preliminary analysis of 1727 patients revealed that the mortality rate in patients treated with flecainide or encainide was 7.7% compared with 3.0% in controls. The most likely explanation for the result was the induction of fatal ventricular arrhythmias, possibly in conjunction with ischaemia by flecainide and encainide, i.e. a proarrhythmic effect (Cardiac Arrhythmia Suppression Trial (CAST) Investigators 1989 Preliminary report: effect of encainide and flecainide on mortality in a randomised trial of arrhythmia suppression after myocardial infarction. New England Journal of Medicine 321:406–412).
6 Singh B N, Connolly S J, Crijns H J et al; EURIDIS and ADONIS Investigators 2007 Dronedarone for maintenance of sinus rhythm in atrial fibrillation or flutter. New England Journal of Medicine 357:987–999.
7 Hohnloser S H, Crijns H J, van Eickels M et al; ATHENA Investigators 2009 Effect of dronedarone on cardiovascular events in atrial fibrillation. New England Journal of Medicine 360:668–678.
8 Le Heuzey J Y, De Ferrari G M, Radzik D et al 2010 A short-term, randomised, double-blind, parallel-group study to evaluate the efficacy and safety of dronedarone versus amiodarone in patients with persistent atrial fibrillation: the DIONYSOS study. Journal of Cardiovascular Electrophysiology 21:597–605.
9 In 1775 Dr William Withering was making a routine journey from Birmingham (England), his home, to see patients at the Stafford Infirmary. While the carriage horses were being changed half-way, he was asked to see an old dropsical (oedematous) woman. He thought she would die and so some weeks later, when he heard of her recovery, was interested enough to enquire into the cause. Recovery was attributed to a herb tea containing some 20 ingredients, among which Withering, already the author of a botanical textbook, found it ‘not very difficult … to perceive that the active herb could be no other than the foxglove’. He began to investigate its properties, trying it on the poor of Birmingham, whom he used to see without fee each day. The results were inconclusive and his interest flagged until one day he heard that the principal of an Oxford College had been cured by foxglove after ‘some of the first physicians of the age had declared that they could do no more for him’. This put a new complexion on the matter and, pursuing his investigation, Withering found that foxglove extract caused diuresis in some oedematous patients. He defined the type of patient who might benefit from it and, equally importantly, he standardised his foxglove leaf preparations and was able to lay down accurate dosage schedules. His advice, with little amplification, served until relatively recently (Withering W 1785 An Account of the Foxglove. Robinson, London).
10 To the layperson, ‘shock’ treatment could be interpreted as frights (which stimulate the vagus, as described above), or as the electrical sort. Dr James Le Fanu quotes a Belfast doctor who reported a farmer with a solution that covered both possibilities. He had suffered from episodes of palpitations and dizziness for 30 years. When he first got them, he would jump from a barrel and thump his feet hard on the ground at landing. This became less effective with time. His next ‘cure’ was to remove his clothes, climb a ladder and jump from a considerable height into a cold water tank on the farm. Later, he discovered the best and simplest treatment was to grab hold of his high-voltage electrified cattle fence – although if he was wearing Wellington (rubber) boots he found he had to earth the shock, so besides grabbing the fence with one hand he simultaneously shoved a finger of the other hand into the ground.
11 See also UK Resuscitation Council guidelines (Figs 25.3–25.5).
12 The Task Force for the Management of Atrial Fibrillation of the European Society of Cardiology (ESC) has issued guidance preferring these agents to amiodarone depending on the characteristics of the patient (see Fig. 25.6). A J Camm et al 2010 European Heart Journal 31:2369–2429.
13 French: torsade, twist; pointe, point. ‘Twisting of the points’, referring to the characteristic sequence of ‘up’ followed by ‘down’ QRS complexes. The appearance has been called a ‘cardiac ballet’.
14 Roden D M 2004 Drug-induced prolongation of the QT interval. New England Journal of Medicine 350:1013–1022.