Carbohydrate, fat, and protein metabolism
Metabolism comes from the Greek word meaning “to change” and is the totality of chemical reactions within a living organism. Four principles that underlie and guide metabolic functions in humans are “(1) plasma glucose must be maintained within normal limits; (2) an optimal source of glycogen must be maintained as an emergency fuel; (3) an optimal supply of protein must be maintained for use in enzymatic mechanisms of metabolism as well as muscular mobility; excess protein is converted to fat and the nitrogen released is excreted in urine; and (4) protein must be conserved when it is scarce and stored fat used in time of caloric need.”47,p.2
Maternal physiologic adaptations
Metabolic adaptations during pregnancy are directed toward (1) ensuring satisfactory growth and development of the fetus; (2) providing the fetus with adequate stores of energy and substrates needed for transition to extrauterine life; (3) meeting maternal needs to cope with the increased physiologic demands of pregnancy; and (4) providing energy and substrate stores for the demands of pregnancy, labor, and lactation.6 The first two demands compete with the third and fourth demands. As a result, alterations in maternal metabolic processes can significantly affect maternal and fetal health status.
Pregnancy involves a “coordinated series of physiologic adjustments which act in concert to preserve maternal homeostasis while at the same time providing for fetal growth and development.”47 Pregnancy is primarily an anabolic state in which food intake and appetite are increased, activity is decreased, approximately 3.5 kg of fat is deposited, energy reserves of approximately 30,000 kcal (125,550 J) are established, and 900 g of new protein is synthesized by the mother, fetus, and placenta. The overall energy cost of reproduction is estimated at 75,000 to 85,000 kcal (313,875 to 355,725 J).6,80,82 Anabolic aspects are most prominent during the first two trimesters when, due to enhanced lipogenesis, accumulation of maternal fat and increased blood volume lead to maternal weight gain (Figure 16-1).19,78 Protein and glycogen synthesis increase in muscle with increased glycogenolysis (breakdown of glycogen to form glucose) and decreased glycolysis in the liver.78 Insulin increases in response to glucose with a normal or slight increase in peripheral insulin sensitivity and serum glucose levels. This results in uptake of nutrients and maternal fat accumulation. During the third trimester, the woman’s metabolic status becomes more catabolic as stored fat is used (see Figure 16-1). Gluconeogenesis (formation of glucose from amino acids and glycerol) in the liver decreases and intestinal dietary fat absorption increases.78 Lipolytic activity within adipose tissue is enhanced with increases in plasma free fatty acids and glycerol. Maternal ketone production is increased. Counterinsulin hormones increase, leading to insulin resistance. During this phase, maternal weight gain is primarily due to the growing fetus and placenta; the fetus gains 90% of its growth in the last half of pregnancy.12,19,57,80,82
Antepartum period
Pregnancy is associated with major changes in metabolic processes and endocrine function. Pregnancy has been characterized as a metabolic “tug of war” between the competing needs of the mother and the fetus.47 The fetus and placenta influence maternal metabolic alterations in that these tissues become an additional site for metabolism of maternal hormones as well as new sites for hormonal biosynthesis. Many of the metabolic changes during pregnancy are aimed at providing substances (especially glucose, lipids, and amino acids) for the growth and development of the fetus. As the fetus and placenta grow, maternal fuel economy is altered to support this growth.38,109
Basal metabolic rate
The basal metabolic rate (BMR) increases during pregnancy (Figure 16-2). The rate of change varies with maternal prepregnant nutritional status and fetal growth. Significant variations are seen among individual women with up to an eightfold increase reported. Similar variations are reported for fat accretion.17,79,91 If a woman has low energy reserves at conception, there is less of an increase in the BMR and energy is conserved.
The total energy required for pregnancy can be divided into three parts: (1) obligatory energy needed for the fetus, placenta, uterus, and breasts (which is the smallest part); (2) energy for maternal fat storage; and (3) energy maintenance of these new tissues.79 If a woman has lower energy stores, less of the maternal energy intake is needed to maintain new tissues and energy is conserved for maternal basic needs and the fetal-placental unit.79,80,107,109 For example, in undernourished women, fetal weight accounts for 60% of the pregnancy weight gain, versus 25% in a well-nourished woman.109 This energy-sparing response may allow the woman to sustain the pregnancy but is often at the expense of fetal growth, with decreased birth weight and risk of fetal growth restriction.109 Prentice and Goldberg suggest that leptin might monitor a woman’s prepregnancy energy stores and adjust or coordinate maternal metabolic resources.109 Women with large-for-gestational-age (LGA) infants tend to have increased BMR with less maternal energy storage.79,111
The pregnant woman meets the energy demands of pregnancy by increasing her intake, decreasing her activity, or limiting fat storage.80,107 King and colleagues propose three examples of how women in different situations might alter their energy to sustain pregnancy.79 First, an underweight impoverished woman with limited food, poor fat stores, and need for physical work cannot increase food intake or limit physical activity during pregnancy. Her body responds by decreasing basal energy expenditures so that pregnant energy needs are similar to prepregnant needs. Second, a normal weight woman in a developed country with fat stores before pregnancy and adequate nutrition during pregnancy increases fat stores in pregnancy and increases her BMR slightly. Finally, an overweight woman in a developed country increases her BMR by 20% or more, perhaps to reduce additional fat deposition.79
Carbohydrate metabolism
Basal endogenous hepatic glucose production remains sensitive to insulin and increases up to 30% by the third trimester to meet fetal and placental needs.12,16,72,98 Endogenous glucose production increases with gestational age, paralleling fetal and maternal needs.72 Maternal glucose levels are generally 10% to 20% lower than in nonpregnant women. In addition, during the overnight fasting period, maternal plasma glucose values fall to levels 15 to 20 mg/dL (0.8 to 1.1 mmol/L) lower than in nonpregnant women.88 This decrease in glucose leads to lower insulin levels during the postabsorptive state (between meals and overnight) and a tendency toward hypoglycemia and ketosis. The decreased glucose level in the postabsorptive state is due to increased plasma volume in early pregnancy and later to placental transport of glucose to the fetus, which increases during gestation as fetal glucose needs increase.98,101
During the first two trimesters, the pregnant woman is in an anabolic state. Insulin secretion increases with increased peripheral glucose use without increases in insulin resistance.47 As pregnancy progresses, peripheral glucose use by the mother decreases because of increasing insulin resistance. This reduces maternal glucose utilization and makes glucose more readily available to the fetus. The mother compensates by using fat stores to meet her energy needs with breakdown of glycerol to glucose.57 Insulin resistance in the latter part of pregnancy is believed to be a result of a decrease in sensitivity of cell receptors that results from the insulin antagonism effects of hPL, progesterone, and cortisol.16,47 The insulin antagonism is partially modulated by pancreatic β-cell hyperplasia and hypertrophy with increased insulin availability after a meal.16 Pregnancy is also characterized by greater oscillations in insulin and glucagon levels.47 A reduction in the extraction of insulin by the liver may contribute to the peripheral hyperinsulinemia. Variations in hepatic insulin binding may lead to alterations in the ratio of insulin to glucagon.6
Progesterone augments insulin secretion, decreases peripheral insulin effectiveness, and increases insulin levels after a meal. Estrogen increases the level of plasma cortisol (an insulin antagonist), stimulates β-cell hyperplasia (and thus insulin production), and enhances peripheral glucose utilization. Increased levels of both bound and free cortisol decrease hepatic glycogen stores and increase hepatic glucose production. These changes further increase glucose availability for the fetus.88,98
hPL levels correlate with fetal and placental weight and are higher in multiple pregnancies.16 hPL increases synthesis and availability of lipids. Lipids can be used by the mother as an alternative fuel, enhancing availability and transfer of glucose and amino acids to the fetus. A mild form of the metabolic changes seen during pregnancy can be induced by giving hPL to nonpregnant women. However, there is no consistent relationship between hPL levels and insulin requirements in the pregnant diabetic woman.
Protein metabolism
Decreased serum amino acid and serum protein levels are found in pregnancy.6,42,72 This decrease is related to increased placental uptake, increased insulin levels, hepatic diversion of amino acids for gluconeogenesis, and transfer of amino acids to the fetus.6,42 The fetus uses some of these amino acids for glucose formation. Maternal plasma levels of glucogenic amino acids (e.g., those that can be converted into glucose), such as alanine, threonine, glutamate, and serine, are reduced due to placental transfer of these amino acids.31,72 Maternal plasma alanine levels, in particular, are lower because alanine is a key precursor for glucose formation (via gluconeogenesis) by the fetal liver.72
Alterations in protein metabolism during pregnancy have a biphasic pattern.72 During the first half of gestation, maternal protein storage increases, with a net retention of 1.3 g/day of nitrogen.80 Most of this is transported to the fetus, but some is retained in maternal tissues. During the second half, maternal protein use is more economic, with decreased urinary nitrogen excretion, thus conserving protein.86 These changes may be mediated by decreased activity of hepatic enzymes involved in amino acid deamination and urea synthesis.
Lipid metabolism
Pregnancy results in marked alterations in lipid metabolism with a markedly different lipoprotein profile, with marked increases in triglycerides, as well as increases in phospholipids and cholesterol. Basal oxidation of fatty acids increases 70% in pregnancy leading to a relative hyperlipidemia.27 Synthesis of very-low-density lipoprotein (VLDL), low-density lipoprotein (LDL), and high-density lipoprotein (HDL), which are contained in triglycerides, and LDL and HDL, which are found in cholesterol, also increase.59
Lipid metabolism in pregnancy is characterized by two phases and is analogous to the patterns of change in carbohydrate and protein metabolism.19,57 During the first two trimesters, triglyceride synthesis and fat storage increase. VLDL increases threefold in the second and third trimesters. LDL decreases slightly initially, followed by a progressive rise.100 HDL increases progressively to 24 weeks, then decreases to 32 weeks and stabilizes for the remainder of pregnancy.100 Triglycerides increase 40% by 18 weeks and 250% by term.87 Phospholipids and cholesterol levels also increase; the triglyceride-to-cholesterol level remains stable since cholesterol also increases.59,58 By late pregnancy, cholesterol levels are 50% higher than prior to pregnancy, regardless of maternal dietary intake.42 These changes enhance the availability of substrates for the fetus.57
Maternal fat storage is most prominent from 10 to 30 weeks, before the peak of fetal energy demands.79 Promotion of lipogenesis and suppression of lipolysis during this phase are mediated by progressive increases in insulin responsiveness and enhanced by progesterone, cortisol, leptin, and prolactin.6,16,30,42,59 Estrogens decrease lipoprotein lipase activity.18 During this period the pregnant woman experiences a physiologic ketosis with a twofold to threefold increase in baseline ketone body production, with an acute increase after fasting, suggesting enhanced fat utilization.116,130
Lipid metabolism in late pregnancy is illustrated in Figure 16-3. The third trimester is characterized by both lipogenesis and lipolysis, with increased breakdown of fat deposits.19,32,57,58 These changes are mediated by increased adipose tissue lipolytic activity and decreased lipoprotein lipase (LPL) activity (due to estrogens).19,58 LPL, which is present in the capillary endothelium of extrahepatic tissues, hydrolyzes circulating triglycerides, including VLDL, producing free fatty acids and glycerol.58 Adipose tissue is broken down into free fatty acids and glycerol, which circulate to the liver and are converted to active forms (acyl-CoA and glycerol-3-phosphate) and re-esterified into triglycerides (circulate as VLDL).19,58 Glycerol can also be used for glucose synthesis (gluconeogenesis). The increased lipolysis is due to the rise of hPL levels with its antiinsulinogenic and lipolytic effects, as well as the effects of cortisol, glucagon, and prolactin, resulting in increased lipolytic activity in adipose tissue.16,19,57,58,78 Enhanced ketogenesis in the liver is a consequence of increased oxidation of free fatty acids for energy and release of ketone bodies.58 The fat mobilization is associated with increased glucose and amino acid uptake by the fetus. Thus fats are used by the mother as an alternative energy substrate, allowing the mother to conserve glucose for the fetus and her central nervous system (CNS) during the second half of gestation.6,16,59,58 Fetal glucose and ketone uptake increases. Ketones are used by the fetus for oxidative metabolism, lipogenesis, triglyceride production, and as substrate for brain lipid synthesis.58,78,116,130
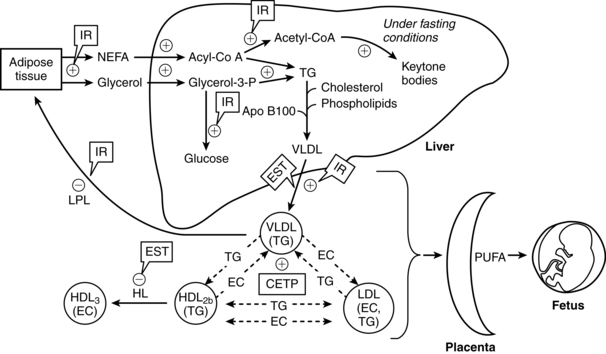
The changes in lipid metabolism during pregnancy are reflected in changes in maternal serum free fatty acid concentrations as well as plasma triglyceride, cholesterol, and phospholipid levels. These changes may be exaggerated in obese women.27 The minimal change in free fatty acids during early pregnancy is probably due to increased fat storage and augmented fat utilization. As maternal fat stores are mobilized, serum levels of triglycerides, free fatty acids, glycerol, and triglyceride-rich lipoproteins (VLDL) increase to peak near term.19,58,59 Because elevations in free fatty acids are due to catabolism of stored triglycerides (into free fatty acid and glycerol), changes in free fatty acids are mirrored by changes in glycerol.6 Hypertriglyceridemia during the third trimester is primarily due to increases in VLDLs and decreased peripheral clearance.16,19,58,59 The increase is due to reduced VLDL clearance secondary to decreased activity of LPL in the liver and adipose tissue, enhanced activity of cholesterol ester transferase, increased gastrointestinal absorption of lipids, and increased hepatic triglyceride production.16,32,58,59 The decrease in lipoprotein lipase is due to increases in estrogens in late pregnancy and the increasing insulin resistance.58,78 Near term, lipoprotein lipase activity increases in the mammary glands. This enhances availability of triglycerides for milk production.57
Changes in lipid metabolism are accompanied by functional and morphologic changes in the adipocytes. Hypertrophy of these cells accommodates the increased fat storage during the first two thirds of pregnancy. In the last trimester, maximal glucose transport, glucose oxidation, and lipogenesis within the adipocytes decrease.42 The number of insulin receptors on the adipocytes increases in the first part of pregnancy and returns to prepregnant levels by term.6,42 Because responsiveness of adipose tissue to insulin is not diminished as much as that of other tissues, these changes in the adipocytes facilitate fat storage.42 After a meal, maternal fat stores are replenished by increased glucose uptake, incorporation of glucose into glycerol, and esterification of fatty acids by adipocytes.6
Insulin
Insulin levels and responsiveness of tissues to insulin change dramatically during pregnancy, leading to peripheral insulin resistance. Peripheral insulin resistance is “the decreased ability of insulin to affect glucose uptake, primarily in skeletal muscle and to a lesser degree in adipose tissue.”98 Actions of insulin are summarized in Table 16-1.40 Insulin production and sensitivity during pregnancy differ in early compared to later pregnancy.16 During early pregnancy (up to 12 to 14 weeks), insulin responses are enhanced, sending glucose to the embryo and young fetus. The pregnant woman has a normal glucose tolerance, basal glucose production, and peripheral muscle sensitivity to insulin.16,110 Adipose tissue is more sensitive to insulin during this period, resulting in lipogenesis and fat storage.110
Table 16-1
Compiled from Guyton, A.C., & Hall, J.E. (2010). Textbook of medical physiology (12th ed.). Philadelphia: Saunders Elsevier; Widmaier, E., Raff, H., & Strang, K.T. (2005). Vander’s Human physiology: The mechanism of body function (10th ed.). New York: McGraw-Hill.
In later pregnancy (from 20 weeks to term), insulin sensitivity decreases and insulin secretion and resistance increase with decreased glucose uptake by muscle and adipose tissue.16,131 Maternal insulin levels increase 2.5- to threefold by the third trimester.47,78,98 These changes are accompanied by maternal pancreatic hypertrophy and hyperplasia.110 Increased insulin secretion ensures adequate maternal protein synthesis in the face of increasing resistance of peripheral tissues to the effects of insulin. With increasing gestation, mean insulin sensitivity decreases up to 50% to 70%.78 Tissue resistance is most prominent in liver, adipose, and muscle cells, and is further altered in women with preeclampsia.119 The increasing insulin resistance promotes nutrient flux from the mother to the fetus and promotion of adipose tissue accumulation.78 Insulin resistance is often exaggerated in overweight and obese women.27
Insulin receptor binding does not change significantly during pregnancy, however, the postreceptor insulin signaling cascade, and thus handling of glucose by cells, is altered in skeletal muscle and adipose tissue.110 During pregnancy insulin receptor substrate-1 (IRS-1) is down-regulated, affecting insulin uptake and use by cells.98 The postreceptor handling of glucose during pregnancy is altered by decreased IRS-1 expression (especially in skeletal muscle), altered expression of tyrosine kinase activity, and decreased expression of GLUT-4 glucose transporter protein in adipose tissue, which normally promotes glucose uptake.98,110
Insulin resistance is mediated by the increasing levels of placental hormones, especially estrogens, progesterone, and hPL, as well as prolactin and cortisol, and is minimally affected by changes in blood glucose levels.98 Other factors that play an important role in the insulin resistance of pregnancy are tumor necrosis factor-α and other cytokines, circulating free fatty acids, leptin, and placental growth hormone, which stimulates insulin-like growth factor-I (IGF-I).15,78,197,124 In late pregnancy, although basal insulin levels are elevated, maternal blood glucose values are similar to prepregnant levels.6 Increased insulin secretion after a meal (in response to the higher blood glucose) offsets the contrainsulin effects of the placental hormones and facilitates movement of nutrients to the fetus. The insulin resistance of late pregnancy enhances maternal fat breakdown and increased gluconeogenesis and ketogenesis in the postabsorptive state, the state in which changes in insulin response are most apparent.16,47,58 If the pregnant woman is not able to elevate her insulin secretion to overcome the increasing pregnancy-induced insulin resistance, maternal and fetal hyperglycemia will result and metabolic abnormalities such as gestational diabetes may develop or existing metabolic problems such as diabetes will be aggravated.
Alterations in insulin production and responsiveness are critical in integrating changes in carbohydrate and fat metabolism throughout the course of pregnancy. Baird summarizes these interactions as follows: During early pregnancy, increased insulin in response to glucose, minimal changes in insulin sensitivity, and increased number of insulin receptors on the adipocytes result in normal or slightly enhanced carbohydrate tolerance. The increased hepatic synthesis and secretion of triglycerides during this period, along with a normal or slightly elevated removal of triglycerides from the circulation, lead to a net storage of fat. During late pregnancy, the elevated plasma insulin, decrease in numbers of adipocyte insulin receptors to prepregnant levels, and increasing insulin resistance result in reduced assimilation of glucose and triglycerides by maternal tissues, greater transfer of these substances to the fetus, and increased lipolysis. The net result is a decrease in maternal blood glucose, increased glucose turnover, and greater maternal reliance on lipid catabolism for energy.6 Changes in lipid, carbohydrate, and protein metabolism are summarized in Table 16-2.
Table 16-2
Maternal Metabolic Processes during Pregnancy: Relationship between Hormonal and Metabolic Changes
HORMONAL CHANGE | EFFECT | METABOLIC CHANGE |
Increased hPL | ||
Increased prolactin | Insulin resistance | |
Increased bound and free cortisol | Ensures glucose and amino acids to fetus | |
Increased estrogen, progesterone, and insulin during early pregnancy | Anabolic fat storage during early pregnancy | |
Increased hPL in late pregnancy | Lipolysis | Catabolic fat mobilization in late pregnancy |
Adapted from Moore, T.R. (2004). Diabetes and pregnancy. In R.K. Creasy, R. Resnik, & J.D. Iams (Eds.), Maternal-fetal medicine: Principles and practice (5th ed.). Philadelphia: Saunders. hPL, human placental lactogen.
Absorptive versus postabsorptive states
In addition to phasic changes in metabolic processes in early versus late pregnancy, metabolism of amino acids, carbohydrates, and fats also varies on a daily basis depending on whether the mother is in the absorptive (fed) or postabsorptive state (Figures 16-4 and16-5). As a result of these changes, pregnancy has been characterized as a time of both “accelerated starvation” and “facilitated anabolism.”96
Absorptive state.
During the absorptive (fed) state, ingested nutrients (amino acid, glucose, triglyceride) are entering the blood from the gastrointestinal tract (see Chapter 12) and must be oxidized for energy, used for protein synthesis, or stored. The average meal takes 4 to 6 hours for complete absorption. In this state anabolism exceeds catabolism and glucose is the major energy source. Small amounts of amino acid and fat are converted into energy or used to resynthesize body proteins or for structural fat. Most of the amino acid and fat and any extra carbohydrate are transformed into adipose tissue; carbohydrate is also stored as glycogen.
Insulin has an anabolic and anticatabolic role during this state.78 Insulin secretion increases and plasma insulin levels rise. Insulin promotes glucose uptake by the hepatocytes and peripheral tissues, inhibits glycogen breakdown, and inhibits lipolysis in adipose tissue. Glucose is converted to glycogen for storage in the liver, cardiac muscle, and skeletal muscle. Muscle amino acid uptake is enhanced and proteolysis inhibited.78
After a meal the pregnant woman has higher glucose, insulin, and triglyceride levels and suppression of glycogen compared to nonpregnant women. Thus the absorptive state during pregnancy (see Figure 16-4, A) is characterized by relative hyperinsulinemia (related to decreased insulin sensitivity), hyperglycemia (due to failure of liver glucose uptake), insulin resistance (especially in skeletal muscle), hypertriglyceridemia, and lipogenesis (more glucose is converted to triglyceride for storage).6,16,78,119,129 Pregnancy has been called a state of facilitated anabolism to describe these metabolic alterations that conserve energy, especially during early pregnancy.94 These changes increase glucose availability for transport to the fetus; increase availability of an alternate energy source (triglycerides) for maternal needs; and provide fewer stimuli for maternal gluconeogenesis, glycogenolysis, and ketogenesis.42,47
Maternal blood glucose levels may rise transiently to 130 to 140 mg/dL (7.2 to 7.8 mmol/L) (see Figure 16-5). Gluconeogenesis and circulating free fatty acids are decreased. Under the influence of placental hormones, resistance of the liver and peripheral tissues to insulin is increased by as much as 60% to 70%.119,129 The hyperinsulinemic response is most marked during the third trimester because of hypertrophy and hyperplasia of islet β cells. These cells become more responsive to alterations in blood glucose and amino acid levels. The increased insulin levels after eating overcome the insulin resistance to allow glucose uptake by muscles for storage as glycogen.119 Even with increased production of insulin, however, overall glucose levels are maintained, although at a relatively lower level than in nonpregnant women because of the counterbalancing effects of estrogen, progesterone, and hPL.
Postabsorptive and fasting state.
In the postabsorptive state (i.e., when nutrients are not entering the blood from the intestines, beginning 4 to 6 hours after a meal) and fasting state (12 or more hours after the last meal), energy must be supplied by body stores. Insulin levels are low. Fat and protein synthesis are decreased and catabolism exceeds anabolism. Gluconeogenesis (production of new glucose from lactate, amino acids [especially alanine], and glycerol) and catabolism of fat are the main sources of energy.78 Plasma glucose levels are maintained during the postabsorptive state by use of these alternate sources of glucose and glucose-sparing or fat-utilization reactions.16,42 The CNS continues to use glucose, while other organs and tissues become glucose sparing, depending on fat as the primary energy source.
During the fasting state (e.g., overnight between the evening meal and breakfast), plasma glucose levels decline. The magnitude of the decline is greater in pregnant women than in nonpregnant women, due to the continuous transfer of glucose to the fetus, and is associated with a more rapid conversion to fat metabolism. Fatty acids are liberated by breakdown of triglycerides. Lipolysis yields glycerol (converted to glucose by the liver) and free fatty acids, which are catabolized to ketone bodies (oxidized for energy). Under homeostatic conditions ketones do not accumulate in the body to produce ketoacidosis because excess ketones not needed for energy are rapidly cleared by the kidneys.78
This response is an exaggeration of the changes normally seen during the overnight fast in nonpregnant women. These changes would normally raise blood glucose levels, but in the pregnant woman the fasting glucose level tends to be lower because of the limited availability of substrate for gluconeogenesis. For example, as early as 15 weeks’ gestation, maternal glucose levels after a 12- to 14-hour overnight fast are 15 to 20 mg (0.8 to 1.1 mmol/L) lower than levels in nonpregnant women. The decrease in glucose during the overnight “fasting” period is especially prominent during the second and third trimesters.6,78
The exaggerated maternal responses during the fasting state are influenced by (1) continuous placental uptake of glucose and amino acids from the maternal circulation; (2) decreased peripheral utilization of glucose as plasma concentrations of ketones and free fatty acids increase; (3) decreased renal absorption of glucose; and (4) decreased hepatic glucose production. This response, seen primarily in late pregnancy, is characterized by lower fasting glucose and amino acid levels, increased blood glucose levels after eating, and increased plasma free fatty acids, triglycerides, ketones, and insulin secretion in response to glucose.12,42,78 Thus the postabsorptive fasting state in pregnancy is characterized by a relative hypoglycemia (due to the fetal siphon, increased renal losses, and decreased liver production), hyperketonuria (ketones used as an alternative energy source), hypoaminoacidemia (due to placental transfer for use in fetal glucose production), and hypoinsulinemia (see Figures 16-4, B, and 16-5).12,24,57,72,78,98 Hypoalaninemia also develops because maternal protein stores can provide only limited substrate, which is insufficient to meet both maternal and fetal amino acid needs.47 Increased insulin during the postabsorptive state enhances uptake of glucose into the maternal skeletal muscle and adipose tissue. In lean women, this leads to suppression of hepatic glucose production in late pregnancy. Less suppression is seen in obese women, therefore the alteration in insulin sensitivity is greater in obese than nonobese women.98
Levels of lipoprotein lipase are increased, enhancing triglyceride breakdown with release of free fatty acids and glycerol (which is broken down into glucose) and production of ketone bodies to provide energy when plasma glucose supply is low.16 Glycerol is a source for hepatic gluconeogenesis. The elevated free fatty acids prevent glucose uptake and oxidation by maternal cells, thus preserving glucose for the maternal CNS and the fetus.12 In the nonpregnant woman this switch to fat oxidation occurs after 14 to 18 hours of fasting; during pregnancy the switch occurs after 2 to 3 hours and has been termed accelerated starvation.12,19,42,96
Metabolic changes characteristic of this state are primarily due to hPL, which promotes lipolysis to increase free fatty acid levels and opposes insulin action, thus increasing glucose availability to the fetus. Other factors influencing this response include increased glucose utilization by the fetus (“the fetal siphon”) and mother, along with an increase in the volume of distribution for glucose (i.e., hemodilution).78 During the second half of pregnancy, effectiveness of insulin in translocating glucose into cells is reduced.78 Because insulin is the ultimate arbitrator of both the absorptive and postabsorptive states, alterations in insulin secretion alter substrate availability to the mother and fetus.47 The insulin antagonism in pregnancy is progressive, paralleling the growth of the fetoplacental unit, and disappears immediately after delivery. Placental hormones and other substances are major factors in producing this insulin antagonism.
Drainage of glucose and amino acids by the fetus may lead to increased maternal appetite and a feeling of faintness sometimes experienced in pregnancy. Pregnant women may experience more rapid development of ketosis and fasting hypoglycemia after food deprivation. With greater maternal reliance on fat utilization during pregnancy, production of ketone bodies and risks of abnormalities such as acidosis are increased. Dieting and caloric restriction during pregnancy should be considered potentially dangerous to both the mother and fetus.37
Effects of placental hormones and other substances
The phasic changes in carbohydrate, lipid, and protein metabolism during pregnancy are due to the interplay of placental hormones, especially estrogen, progesterone, hPL, and leptin.75,98 During the first half of pregnancy, metabolism is affected primarily by estrogens and progesterone. In late pregnancy the influences of increasing concentrations of hPL and leptin become more prominent. Maternal metabolic changes are also influenced by prolactin and cortisol.12
Estrogen stimulates islet β-cell hyperplasia and insulin secretion, enhances glucose utilization in peripheral tissues, and increases plasma cortisol, an insulin antagonist. As a result, particularly in the first half of gestation, estrogen decreases fasting glucose levels, improves glucose tolerance, and increases glycogen storage.78 Progesterone augments insulin secretion, increases fasting plasma insulin concentrations, and diminishes peripheral insulin effectiveness. Cortisol mediates these changes by inhibiting glucose uptake and oxidation, increasing liver glucose production, and possibly augmenting glucagon secretion.12 Cortisol increases to 2.5 times normal levels by late pregnancy.12,131 Prolactin increases fivefold to 10-fold and stimulates insulin production and, at least in animal models, increases the number of β-cell receptors.131
Human placental lactogen (hPL), also called human chorionic somatomammotropin (hCS), is a polypeptide hormone produced by the syncytiotrophoblast (see Chapter 3). hPL is the most potent insulin antagonist of the placental hormones. This hormone is secreted primarily into the maternal circulation although some is also secreted into fetal circulation after around 6 weeks.43 Levels of hPL increase markedly after 20 weeks. Because effects of hPL are similar to those of growth hormone, it has been called the “growth hormone” of the second half of pregnancy.12 hPL action increases availability of maternal glucose and amino acids to the fetus. Other effects of hPL include diminished tissue response to insulin; increased β-cell mass; lipolysis, which increases plasma free fatty acids; enhanced nitrogen retention; decreased urinary potassium excretion; and increased calcium excretion. The major effect is sparing of maternal carbohydrate (glucose) by providing alternative energy sources such as free fatty acids for the mother.16
Several adipokines, including leptin, adiponectin, and retinal binding protein 4 (RBP4), are also important for ensuring adequate substrate for fetal growth.58 Leptin, a protein product of the obese (ob) gene, was identified in 1994. It is produced and secreted by adipose tissue and other tissues, including the placenta during pregnancy. Leptin is involved in regulating appetite and food intake and enhancing energy expenditure, and may also be important in signaling readiness for sexual maturation at puberty.128 Leptin receptors are found in the hypothalamus, placenta, muscle, liver, lymphoid tissue, uterus, chorion, amnion, pancreas, ovary, and adipose tissue.78,124 Placental leptin is secreted into both maternal and fetal circulations.86
Leptin plays a role in maturation and regulation of reproduction and “may serve as a detector of long-term metabolic fuel availability signaling the presence of significant maternal fat stores to initiate reproduction.”16 Leptin modulates glucose metabolism, insulin sensitivity, and adipose tissue lipolysis during pregnancy, enhancing breakdown of maternal fat stores in the second half of pregnancy and availability of glucose and lipids for the fetus.58 Other roles of leptin in pregnancy may be to mediate changes in appetite, thermogenesis, and lipid metabolism.16,49 Leptin may also modulate fetal growth. Concentrations of leptin increase from 6 to 8 weeks’ gestation, rising further in the second and (especially) third trimesters.81 The increase is probably primarily due to increased placental leptin production. Levels in early pregnancy correlate with maternal weight and body mass index; this correlation is not found in later pregnancy and may reflect a form of leptin resistance (similar to that seen with obesity).16,81
Adiponectin is a protein, secreted by adipose tissue, that is involved in modulating glucose and lipid metabolism and enhancing insulin sensitivity.58 Changes in leptin and adiponectin have been reported in complicated pregnancies. For example, both leptin and adiponectin are increased in women with severe preeclampsia.7,84,86 Adiponectin is decreased in women with gestational diabetes mellitus, whereas tumor necrosis factor-α (an inflammatory marker) and cord blood leptin are increased.55,58,90,112 Low levels of adiponectin in women with gestational diabetes may play a role in the increased insulin resistance seen in these women.58 Low leptin levels are associated with spontaneous abortion.83 RBP4 may also have a role in glucose metabolism and insulin sensitivity. RBP4 increases with increasing gestational age (probably due to placental production) and is increased in pregnancies complicated by gestational diabetes.58
Intrapartum period
The processes of parturition are dependent on an available supply of glucose and triglycerides as energy sources. In addition, essential fatty acids are important as precursors of prostaglandins (arachidonic acid, from which prostaglandins are derived, is a derivative of essential fatty acid), which are critical to the onset of labor. These relationships are described in Chapter 4.
During labor and delivery, maternal glucose consumption increases markedly to produce the energy required by the uterus and skeletal muscles.47 As a result, maternal insulin requirements fall. Oxytocin may augment or supplant insulin during this period. In animals, oxytocin has been demonstrated to act similarly to insulin; that is, oxytocin stimulates glucose oxidation, lipogenesis, glycogen synthesis, and protein formation.47
Postpartum period
With removal of the placenta, concentrations of placental hormones such as hPL, estrogens, and progesterone fall rapidly within hours after delivery (see Chapter 5). The postpartum woman is in a state of relative hypopituitarism with blunted production of gonadotropins and growth hormone.47 This hypopituitarism may result from the feedback effects of elevated hPL and prolactin levels during pregnancy on the pituitary gland. hPL is similar to growth hormone, and its disappearance with removal of the placenta leaves the woman without its contrainsulin effects during a period of relative deficiency of growth hormone.47 Plasma leptin levels decrease by 24 hours after delivery.83
Fasting plasma glucose levels fall within a few days, then increase reaching late pregnancy fasting levels by 5 days.42 It is unclear when these levels return to prepregnant values.42 The insulin resistance of late pregnancy is reversed soon after delivery.98
Plasma free fatty acids fall to prepregnant levels by 3 days; triglycerides by 2 weeks.18,42 During the first week the decrease in triglycerides coincides with the fall in hPL. This fall is more rapid in women who are breastfeeding.18,58 In these women, free fatty acids rise to late pregnancy levels by 6 weeks, followed by a decrease to prepregnant levels by 3 to 6 months.6 The increasing levels of fatty acids from 1 to 6 weeks postpartum may reflect maternal use of other nutrients for milk production.6 Cholesterol levels slowly decrease to prepregnant levels over the first few weeks postpartum.42 Maternal plasma amino acid levels return to prepregnant values of approximately 4.3 mg/dL (0.043 g/L) (versus pregnant values of 3.5 mg/dL [0.35 g/L]) by several days after birth.1,35
Clinical implications for the pregnant woman and her fetus
The metabolic adaptations of pregnancy safeguard against variations in maternal caloric intake, changes in activity, increased metabolic efficiency, and changes in the metabolism of carbohydrates, fats, and proteins.6 These metabolic changes occur in a phasic pattern—probably programmed by placental hormones—that spreads the energy costs and protein requirements of pregnancy over the entire 9 months of gestation. In early pregnancy, energy is conserved (facilitated anabolism), followed by later redirection of energy (glucose) to the fetus (“accelerated starvation”), whereas throughout pregnancy the mother uses protein more economically to provide adequate amino acids for development of the fetal brain and other organs.6,47 Pregnancy has also been characterized as a diabetogenic state. This state is reflected in the elevated blood glucose levels in association with increasing insulin resistance. This state is described in this section along with the basis for alterations in the glucose tolerance test (GTT) and the effects of the normal metabolic changes of pregnancy on the diabetic woman and her fetus.
The diabetogenic effects of pregnancy are reflected by alterations in the GTT, with higher glucose values after a meal reflecting an acquired resistance to insulin. The alterations in carbohydrate metabolism are most evident during late pregnancy in the absorptive state (see Figure 16-4, A). When the woman is in this state and glucose is being added to the plasma from the gut, her blood glucose levels do not drop as rapidly as usual, even in the face of higher circulating insulin levels. This response results from decreased maternal sensitivity to insulin due to the action of hormones such as hPL, progesterone, and cortisol. Secretion of these hormones increases during the second half of pregnancy; therefore diabetogenic effects are most prominent during this period. Insulin resistance is somewhat compensated for by increased plasma insulin concentrations.78
Effects of metabolic changes on glucose tolerance tests
Two methods commonly used to evaluate glucose tolerance in pregnancy are (1) a one-step 75-g, 2-hour test (recommended by the World Health Organization); and (2) a two-step test with a 1-hour 50-g glucose challenge (screening test) followed by a 100-g, 3-hour oral glucose tolerance test (OGTT) if challenge levels are either 135 mg/dL (7.5 mmol/L) or greater (more women meet criteria for the 3-hour test, but a greater proportion of women with gestational diabetes mellitus [GDM] are identified) or 140 mg/dL (7.8 mmol/L).78,98 The one-step testing has a lower detection rate for GDM.97 The two-step test has been used most commonly in the United States, whereas the one-step test is used most commonly in other countries.95,98 The Fifth International Workshop Conference on Gestational Diabees Mellitus recommended the two-step test.95 However, following the Hyperglycemia and Adverse Pregnancy Outcome (HAPO) study, the International Association of Diabetes and Pregnancy Study Groups (IADPSG) recommended use of a 75-g glucose load followed by fasting, 12-, and 2-hour plasma glucose concentrations.64
During pregnancy, the initial fasting blood glucose value is lower than in nonpregnant individuals, because of decreased glucose utilization and increased fat utilization by the mother (making increased glucose available to the fetus) and the subsequent effects of the fetal siphon. Blood glucose levels tend to remain high after ingestion of carbohydrates for a longer period of time secondary to insulin antagonism and decreased insulin sensitivity. Normally the magnitude of the increase in blood glucose after a carbohydrate feeding is a reflection of failure in glucose uptake by the liver. During pregnancy the increased glucose response in the face of increased endogenous insulin confirms the relative insensitivity and resistance of the liver (as well as peripheral tissues such as muscle and adipose tissue) to insulin.78,98
Maternal-fetal relationships
Growth and development of the fetus is dependent upon the availability of a constant supply of glucose, amino acids, and lipids from the mother for energy, protein synthesis, and production of new tissues. The fetus must also develop adequate stores of these substances to meet the demands of the intrapartum period and transition to extrauterine life. Placental transfer of selected nutrients and hormones is summarized in Figure 16-6. Fetal requirements for substrates involved in carbohydrate, fat, and protein metabolism are discussed in the next section.
Alterations in maternal metabolic processes or in placental transfer of essential nutrients that increase the availability of specific substrates are usually an advantage to the fetus but can be a disadvantage in altered maternal metabolic states. For example, because fetal energy requirements are met almost exclusively by glucose, the metabolic changes in pregnancy increase availability of glucose in maternal plasma for placental transfer by reducing the efficiency of maternal glucose storage. If the usual metabolic changes of pregnancy or placental function are altered, however, variations in fetal growth—such as occur in the infant of a diabetic mother or the an infant with fetal growth restriction—may develop.122 Maternal glucose infusions during the intrapartum period can lead to a fetal hyperglycemia that stimulates insulin and inhibits glucagon secretion. This may delay gluconeogenesis after birth and increase the risk of neonatal hypoglycemia.
The placenta is a highly metabolic organ with its own substrate needs. Placental metabolic activities include glycolysis, gluconeogenesis, glycogenesis, oxidation, protein synthesis, amino acid interconversion, triglyceride synthesis, and alterations in the length of fatty acid chains (see Chapter 3). The placenta can modify nutrient uptake to meet fetal growth demands.115 The degree of glucose uptake by the placenta is similar to that of the brain. Cholesterol from the mother is essential for placental synthesis of estrogens and progesterone. Fatty acids are needed by the placenta for oxidation and membrane formation.16 Leptin may have a role in coordinating placental metabolism.81
Glucose is the nutrient that crosses the placenta in highest concentrations. The increased maternal reliance on fats as an alternative energy source, especially during the maternal postabsorptive fasting state, conserves maternal glucose for transfer to the fetus. The fetal-placental unit uses approximately 50% of the total maternal glucose needed for pregnancy.57 Glucose crosses via facilitated transport by insulin dependent glucose transporters.81 Amino acids are transferred via active transport because levels are higher in the fetus than in the mother. Amino acids are removed from maternal circulation and concentrated in the placental intercellular matrix. As fetal amino acid levels fall, these placental stores are transferred to the fetus. Mechanisms for amino acid transfer include direct transfer from mother to fetus without modification in the placenta, metabolism of maternally derived amino acids by the placenta to produce other amino acids (which are then transferred to the fetus), and production of new amino acids by the placenta for fetal transfer.57,61