Chapter 52A Cancer and the Nervous System
Epidemiology of Brain Tumors
Primary brain tumors are a diverse group of neoplasms arising from different cells of the central nervous system (CNS). Light microscopy classifies these tumors according to predominant cell type and grades them for malignancy based on the presence or absence of standard histopathological features. Gliomas are the most common malignant primary brain tumors (80%) and constitute 32% of all brain and CNS tumors; these tumors arise from astrocytes (astrocytomas), oligodendrocytes (oligodendrogliomas), or ependymal cells (ependymomas). Glioblastoma accounts for approximately half of gliomas (54%), and astrocytomas (including glioblastoma) account for approximately three-quarters of gliomas (76%) (CBTRUS, 2010). Meningiomas, which arise in the meninges, are also common brain tumors (33%), but unlike gliomas, they demonstrate mostly nonmalignant behavior (CBTRUS, 2010). The etiology of brain tumors remains unknown despite a large number of epidemiological studies. This type of cancer is associated with a unique set of challenges for observational study designs (Box 52A.1). Despite a slow start, observational brain tumor studies are likely to yield important clues into the pathogenesis of this cancer.
Classification
The different cellular origins of brain tumors contribute to the difficulty in achieving a single, widely accepted classification system. Historical attempts at developing a classification system for brain tumors date back to the 1830s. The most widely accepted classification for primary CNS tumors is the World Health Organization (WHO) system, developed in 1979 and subsequently revised in 1993, 2000, and 2007 (Kleihues et al., 2000; Louis et al., 2007). In this system, all CNS tumors are assigned different grades (I to IV) representing an estimate of malignancy. Molecular subclassification of specific primary CNS tumors has become routine in practice and may better predict prognosis in some cases. Chapter 52B contains a comprehensive discussion and history of brain tumor classification.
Descriptive Epidemiology
Incidence
The traditional source of descriptive data on brain tumors has been the Surveillance, Epidemiology, and End Results (SEER) program sponsored by the National Cancer Institute (NCI). This program collects population-based cancer data on approximately 26% of the U.S. population (including cancer registries for nine states) to gauge national trends in cancer incidence and survival (National Cancer Institute, 2010). Until recently these data encompassed only malignant tumors; however, effective January 2004, all cancer surveillance registries are required to expand their primary brain tumor data collection to include tumors of benign or uncertain behavior (Benign Brain Tumor Cancer Registries Amendment Act; Public Law 107-260). The Central Brain Tumor Registry of the United States (CBTRUS) established in 1992 is the nation’s largest population-based registry of primary brain and central nervous system tumors, compiling information from 47 population-based cancer registries (CBTRUS, 2010).
Incidence estimates differ according to the inclusion or exclusion of nonmalignant brain tumors. The American Cancer Society estimated the diagnosis of approximately 22,020 new cases of malignant primary CNS tumors in the United States in 2010 (American Cancer Society, 2010). For 2010, CBTRUS estimates approximately 23,720 new cases of malignant and 39,210 nonmalignant primary CNS tumors in the United States (CBTRUS, 2010). The annual incidence rate for malignant brain cancer for all races from 2004 to 2006 was 7.2 per 100,000 person-years and 11.5 per 100,000 person-years for primary nonmalignant brain tumors (CBTRUS, 2010).
Mortality and Prognostic Factors
Although primary malignant brain tumors account for only 2% of all cancers and are one-fifth as common as breast or lung cancer, they contribute to substantial morbidity, and prognosis is poor. The 5-year survival rate for primary malignant brain tumors (36% between 1999 and 2005) is the sixth lowest among all types of cancer (following pancreas, liver, esophagus, lung, stomach, respectively) despite having increased over the past 30 years (from 24%) (American Cancer Society, 2010). The 5-year survival rates vary substantially by histological subtypes, 79.1% for oligodendroglioma, 27.4% for anaplastic astrocytoma, and 4.5% for glioblastoma (CBTRUS, 2010). Malignant CNS tumors accounted for approximately 13,140 deaths in 2010 (CBTRUS, 2010). Age-specific mortality rates from brain tumors among all races demonstrate gradual increases with each decade until age 55, after which the rate increases dramatically (Fig. 52A.1).
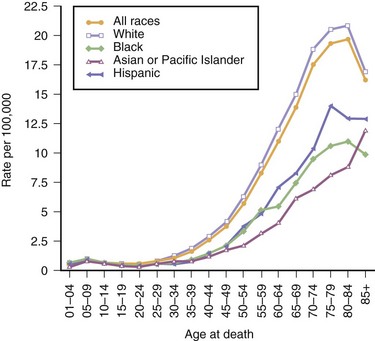
Surveillance, Epidemiology, and End Results (SEER) Program (www.seer.cancer.gov) SEER*Stat Database: Mortality—All COD, Public-Use With State, Total U.S. for Expanded Races/Hispanics (1990-2006), National Cancer Institute, DCCPS, Surveillance Research Program, Cancer Statistics Branch, released April 2009. Underlying mortality data provided by NCHS (www.cdc.gov/nchs).
Young age and lower pathological grade are favorable prognostic factors for primary brain tumors (CBTRUS, 2010). Less significant predictors of favorable prognosis include long duration of symptoms, absence of mental changes at the time of diagnosis, cerebellar location of the tumor, small preoperative tumor size, and completeness of surgical resection.
Gender and Race
A slight male predominance exists in the incidence of malignant CNS tumors (7.5 per 100,000 person-years for men versus 5.2 per 100,000 person-years for women) (SEERS, 2010). However, when assessing nonmalignant and malignant CNS tumor types together, the incidence rate in men is lower than in women (14.4 per 100,000 person-years for men versus 16.9 per 100,000 person-years for women; SEERS, 2010). A partial explanation of this gender disparity is the predominance of meningiomas in women (8.2 per 100,000 person-years) compared to men (3.7 per 100,000 person-years) (CBTRUS, 2010). Fig. 52A.2 compares the incidence rates of malignant brain tumors among whites and blacks. Compared to blacks, whites have a higher incidence of malignant brain tumors for both sexes; white males have an incidence rate of 8.4 per 100,000 person-years compared to black males at 3.7 per 100,000 person-years, whereas white females have an annual incidence rate of 6.5 per 100,000 person-years versus an annual incidence rate of 3.3 per 100,000 person-years among black females (Ries et al., 2006). Mortality rates among whites is higher (5.0 per 100,000 persons; 2002-2006) than among blacks (2.0 per 100,000 persons; 2002-2006) (SEERS, 2009), and 5-year relative survival rates are lower among whites (35%) than blacks (41%) (American Cancer Society, 2010). Comparisons between other racial groups within the United States are difficult because of the small numbers of cases, but incidence rates of malignant brain tumors among Asian Americans and Native Americans are lower than for either white or black Americans.
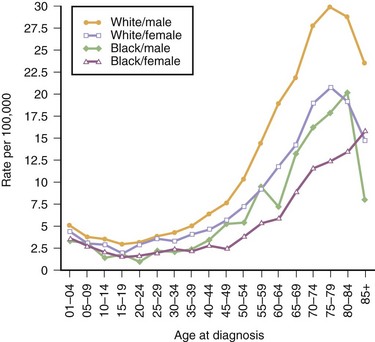
Fig. 52A.2 United States incidence rates for malignant brain cancer for 2000-2007, by race and sex.
Surveillance, Epidemiology, and End Results (SEER) Program (www.seer.cancer.gov) SEER*Stat Database: Incidence—SEER 9 Regs Public-Use, Nov 2009 Sub (1973-2007), National Cancer Institute, DCCPS, Surveillance Research Program, Cancer Statistics Branch, released April 2010, based on the November 2009 submission.
Temporal Trends
Several studies have documented rising incidence rates for brain tumors in a number of industrialized countries. These increases appear confined mainly to the elderly population, with no clear ethnic, gender, or geographical differences. Overall, incidence rates among whites in the United States increased by 1.5% annually between 1975 and 1988 and leveled off between 1988 and 2001 (SEERS, 2010).
The increases in incidence observed in the United States are in accordance with observations in other parts of the world. The responsible factors remain unclear, but at least part of the increase is the result of more complete case ascertainment because of improved diagnostic technology and better access to health care and clinical specialization. The increase in brain tumor incidence correlates with the introduction of noninvasive diagnostic technology including computed tomography (CT) in the 1970s and magnetic resonance imaging (MRI) in the 1980s. In a study using CBTRUS data, the authors reported increases in the incidence of most glioma subgroups but decreases in the incidence of “not otherwise specified” (NOS) subgroups, suggesting that changes in classification and coding of brain tumors are likely to be responsible for some of the observed time trends (Jukich et al., 2001). However, the same study identified increases in the incidence of ependymomas and nerve sheath tumors, which were less likely to be artifacts of improvements in diagnosis.
Geographical Trends and Migrant Studies
Many other countries also experienced increased brain tumor incidence rates over the past 30 years. The highest rates are in industrialized nations such as the United States, Canada, Australia, and the United Kingdom; developing nations have lower incidence rates (Parkin et al., 2005). Generally, brain cancer incidence appears associated with level of economic development; concomitant differences in the availability of diagnostic technology (CT, MRI, neurosurgical technology) and access to health care may account for some of the observed disparities. However, even within the United States, a significant variation in the incidence of primary brain tumors exists among the states. Hawaii has the lowest rate (3.9 per 100,000 person-years), and Maine has the highest rate (8.0 per 100,000 person-years) (State Cancer Profile, 2010) (Fig. 52A.3). In contrast to international trends, a clear relationship between these rates and economic conditions does not exist.
In a study of Italians who migrated to Argentina, brain tumor mortality rates were lower among the migrants than in the host country (Roman, 1998). This suggests environmental factors may influence the development of brain tumors. However, disparities in completeness of case ascertainment in the countries under study complicate the interpretation of this study.
Primary Central Nervous System Lymphoma
The epidemiology of primary central nervous system lymphoma (PCNSL), a type of non-Hodgkin lymphoma, deserves special comment. A dramatic increase in the incidence of PCNSL occurred over the past few decades. This parallels a doubling of the incidence rate of systemic non-Hodgkin lymphoma over the past 4 decades. The incidence peaked in the early 1990s and has since decreased. The primary factor behind the rising incidence is the acquired immune deficiency syndrome (AIDS) epidemic. From 2% to 6% of individuals with AIDS develop PCNSL at some point in their disease course (Fine and Loeffler, 1999). The incidence of AIDS has decreased along with the rate of PCNSL since the beginning of the 1990s. However, AIDS does not fully account for the total increase in the incidence of PCNSL (Rubenstein et al., 2008). Recent studies suggest a continuing increased PCNSL incidence among non-AIDS populations (Haldorsen et al., 2007; Makino et al., 2006).
Analytical Epidemiology
Radiation
Ionizing Radiation
Ionizing radiation exposure from radiation therapy or among atomic bomb survivors is an established cause of certain types of brain tumors, especially meningiomas and nerve sheath tumors. The latency between irradiation and the development of brain tumors may be as short as 5 years or as long as many decades. In a cohort study of 10,834 children treated with cranial radiation for tinea capitis in the 1950s (mean estimated radiation dose, 1.5 Gy), elevated risks were found for nerve sheath tumors (relative risk [RR] 18.8), meningiomas (RR 9.5), and malignant gliomas (RR 2.6) (Ron et al., 1988). A strong dose-response relationship exists such that those who received an estimated dose of 2.5 Gy had relative risks approaching 20 for all brain tumors. With an additional 16 years of follow-up, the relative risk for meningioma remained high (overall, RR 4.63; 95% CI, 2.43-9.12) and was elevated regardless of age at exposure or latency period (Sadetzki et al., 2005). In the same study, the highest risk of malignant glioma was observed among children who were exposed when they were less than 5 years old (RR 3.56; 95% CI, 0.96-9.91). Reports of less dramatic but still significant elevations of risk for meningioma and nerve sheath tumors exist among individuals treated with variable doses of ionizing radiation for thymic enlargement, enlarged tonsils and adenoids, and thyroid and nasopharyngeal conditions. In a mutagen sensitivity assay, peripheral blood lymphocytes from glioma subjects were more prone to chromosomal damage when exposed to gamma radiation. In this study, mutagen sensitivity of lymphocytes was associated with an increased risk of glioma (OR 2.09; 95% CI, 1.43-3.06) (Bondy et al., 2001).
Radiation exposure from diagnostic x-rays is substantially lower than from therapeutic radiation; consequently, the association with brain tumors is not established. Prior to the introduction of fast-speed film in 1956, exposures were several orders of magnitude higher than those used today. Therefore, despite some reports of positive associations between diagnostic x-rays and certain brain tumors (mostly meningioma), most of these included periods of exposure when diagnostic radiation levels were higher. In a recent study with detailed information on dental history, an elevated risk of meningioma was observed among individuals who reported six or more x-rays involving full-mouth series (OR 2.06; 95% CI, 1.03-4.17), but the associations were strongest among those who had frequent dental x-rays in years when doses were higher (Longstreth et al., 2004). In the same study, associations were not observed for bitewings, lateral cephalometric, and panoramic radiographs. For gliomas, past and current studies consistently show no increase in risk with dental x-rays.
Electromagnetic Field Radiation
Numerous studies examining low-frequency electromagnetic field (EMF) radiation and risk of brain cancer have yielded inconsistent results. Several observations cast doubt on the possibility that EMF exposure causes brain tumors; biological plausibility is not established, results of subsequent studies are conflicting, and bias and confounding played a role in earlier studies. Electrical workers have been the subject of several occupational cohort studies based on exposure to EMFs. The possible role of EMFs as a cause of brain tumors is in question. Measuring magnetic field exposure is extremely challenging because there is great variability in field intensity, frequency, and temporal patterns. Earlier studies on this topic did not accurately measure EMF exposure. Some recent studies using accurate estimates of total EMF exposure show a slight increase in risk for brain tumors among workers with very high EMF exposures (Savitz and Loomis, 1995; Savitz et al., 2000), while others did not. Overall, the findings are insufficiently robust to establish causation and, unfortunately, improvements in measurement assessment are unlikely to exceed those currently existing (Ahlbom et al., 2001).
Radiofrequency and Cellular Telephone
Radiofrequency (RF) radiation exposures mainly involve microwave and radar equipment, as well as occupational exposures (sealers, plastic welders, amateur radio operators, medical personnel, and telecommunications workers). Cellular telephones are a source of RF radiation exposure and have received significant attention as a potential risk factor for brain tumors. Despite lack of biological plausibility, coverage in the popular media has caused public concern, and contradictory reports frequently appear in the news. Numerous epidemiological studies have addressed this association, and the results are largely inconsistent. The controversy stems from the many biases in these studies, including recall bias (especially with respect to side of use), selection bias (e.g., controls tend to be more educated and own phones), and measurement error (e.g., reported phone usage does not correlate well with actual use determined from phone records). Overall, most studies report no association between the use of cellular or portable phones and the risk of glioma or meningioma (Christensen et al., 2005; Inskip et al., 2001; Johansen et al., 2001; Lonn et al., 2005; Rothman et al., 1996). Results from a recent and long-awaited international cellular phone case-control study (INTERPHONE) suggest a possible higher risk of glioma among those with the highest exposure levels (INTERPHONE study group, 2010). Once again, bias can not be excluded. In a case-control study of acoustic neuroma, an increased risk was observed among individuals who had 10 or more years of mobile phone use as compared to those who did not use mobile phones (OR 1.8; 95% CI, 1.1-3.1) (Schoemaker et al., 2005). However, a different study found no association (Christensen et al., 2004). The current epidemiological data do not support a causal association between cellular phones and brain tumors when examined critically (Ahlbom et al., 2009); however, several respectable epidemiologists argue that only time will provide the answer, as years of exposure to high levels of cell phone usage is still relatively low (Saracci and Samet, 2010).
Occupational Studies
Several observational studies have found that workers in “white collar” professions have higher rates of brain tumors (Brownson et al., 1990; Carozza et al., 2000). Some of these occupations, such as laboratory research and health professions, involve radiation or chemical exposures, which suggests a possible biological plausibility for a causal relationship. Alternatively, detection bias may account for the excess risk in professionals, because they are more likely to have health insurance and seek medical attention. However, higher rates of brain tumors among professionals in Sweden, where health care is free and available to all citizens, suggests that detection bias may not fully account for the observations (McLaughlin et al., 1987).
Farming as an occupation and residence on a farm have been associated with an elevated risk of brain tumors in several epidemiological studies (meta-analysis RR 1.30; 95% CI, 1.09-1.56) (Khuder et al., 1998). Agricultural workers have exposures to several chemicals in the form of pesticides, herbicides, and fungicides. Similar to other occupational studies, one limitation of studies on agricultural workers is the use of job classification as a proxy for nonspecific chemical exposures. Studies with more detail on individual exposure to pesticides and herbicides have not reported convincing associations with brain tumors (Ruder et al., 2009; Samanic et al., 2008). Reports of increased risks for brain tumors also exist for workers in the vinyl chloride, petrochemical, and rubber industries. Other studies have found an increased risk among aircraft pilots, firefighters, welders, glass manufacturers, tile makers, and metal cutters. However, these findings have been inconsistent over time (Samkange-Zeeb et al., 2010).
Head Trauma
Head trauma is a potential risk factor for brain tumor development. The evidence is strongest for meningiomas and less convincing for gliomas (Wrensch et al., 2000). Anecdotal reports of brain tumors arising after head trauma date back to Harvey Cushing in 1922. Cushing reported the presence of a head scar or skull depression in 8% of meningioma patients. Experimental studies have also implicated physical trauma as a co-carcinogen. An excess risk of meningiomas in persons with a history of serious or repetitive head trauma has been observed in several case-control studies (Preston-Martin et al., 1989, 1998). Other studies have not confirmed an increased risk among children with head injuries (Gousias et al., 2009; Gurney et al., 1996).
Ionizing radiation may be a confounding factor in studies of head trauma and brain tumors. Individuals with a history of head trauma are more likely to have had skull x-rays. Recall bias is another factor complicating interpretation of case-control studies of head trauma and risk of brain tumors. Persons with brain tumors may be more likely to recall minor and major episodes of head injury compared to controls. One study reporting a positive association between head trauma and risk of brain tumors found no association when the definition of head trauma was restricted to episodes requiring medical attention. In a cohort study with an average of 8 years of follow-up, individuals hospitalized for head injury were not at higher risk for subsequent nonmalignant and malignant brain tumors after removing the first year of follow-up; detection bias was likely responsible for the higher risks observed in the first year (Inskip et al., 1998). No association was established between head trauma and brain tumor in a similar cohort with an average follow-up of 10 years (Nygren et al., 2001).
Acoustic neuroma (nerve sheath tumor of cranial nerve VIII) was associated with acoustic noise (trauma) of 10 years’ duration in a case-control study (OR 2.2; 95% CI, 1.12-4.67) (Preston-Martin et al., 1989). A blinded review of job histories was the basis for noise exposure. In a study with a larger sample, a similarly elevated risk of acoustic neuroma was observed among individuals reporting loud noise exposure from any source (OR 1.55; 95% CI, 1.04-2.30) (Edwards et al., 2006). The association was stronger among individuals with exposure to loud noise from machines, power tools, or construction equipment (OR 1.79; 95% CI, 1.11-2.89) or to loud music (OR 2.25; 95% CI, 1.20-4.23) (Edwards et al., 2006). Experimental studies of tissue destruction and repair following acoustic trauma support the biological plausibility of this association.
N-Nitroso Compounds
N-nitroso compounds (NOC) are potent carcinogens in animal models. N-nitroso compounds include nitrosamines, which require metabolic activation to a carcinogenic form, and nitrosamides, which do not require activation. It is well established that nitrosamines can form endogenously from foods treated with sodium nitrite and that certain foods such as bacon and beer contain preformed nitrosamines (Lijinsky, 1999). Although preformed nitrosamine levels in beer and nitrite levels have declined substantially since the 1980s, the small amounts of nitrosamines in food are nonetheless significant because of the possibility that humans are more sensitive to these carcinogens than laboratory rodents (Lijinsky, 1999). A long-standing hypothesis in the epidemiology of gliomas is that NOC exposure may increase risk. Transplacental exposure to ethylnitrosourea, a nitrosamide, results in formation of brain tumors including gliomas in rodents and primates. The addition of vitamin C to the diet prevents tumor formation in this model. Human NOC exposure divides equally between exogenous and endogenous sources. Nitrosamines in tobacco (mainly sidestream smoke), cosmetics, automobile interiors, and cured meats are the best-established exogenous (environmental) sources of NOC exposure. Other sources include rubber products (baby pacifiers, bottle nipples) and certain drugs including antihistamines, diuretics, oral hypoglycemic agents, antibiotics, tranquilizers, and narcotics. N-nitrodiethanolamine, a carcinogen in animal models, occurs mainly as a contaminant in cosmetic products, soaps, shampoos, and hand lotions. Some environmental sources may contain both nitrosamines and nitrosamides. Endogenous formation of NOCs is a complex process that occurs in the stomach. This process is dependent upon the presence of NOC precursors (i.e., nitrates and nitrites), gastric pH, the presence of bacteria, and other physiological parameters.
Epidemiological support for NOC exposure as a risk factor for brain tumors comes mostly from studies of pediatric brain tumors and maternal diet. Several studies have assessed the association between maternal diet and childhood brain tumor risk (Dietrich et al., 2005). A meta-analysis determined that frequent intake of processed meats during pregnancy was associated with an elevated risk of childhood brain tumors (RR 1.68; 95% CI, 1.30-2.17) (Huncharek et al., 2003). However, the authors of this meta-analysis warn not to draw definitive conclusions, based on several study-design limitations such as recall bias and selection bias.
Case-control studies on dietary intakes of foods containing preformed nitrosamines or compounds that can be converted to nitrosamines yield inconsistent results. A few case-control studies reported strong positive associations (e.g., Blowers et al., 1997; Lee et al., 1997), but the potential for bias in these studies is high. A large prospective study with 335 incident cases of glioma examined meats and foods high in nitrites or nitrates and reported no increased risk for any of those foods (Michaud et al., 2009). Overall, despite some suggestion from experimental and observational data that NOC exposure may increase the risk of glioma, this hypothesis remains tenuous.
Vitamins
Vitamins C and E inhibit nitrosation reactions in vivo, and intake of these vitamins can reduce the endogenous formation of NOC in the stomach. Epidemiological studies have demonstrated that consumption of vitamin C reduces the risk of gastric cancer (La Vecchia and Franceschi, 2000), a tumor for which NOC may be a risk factor (Neugut et al., 1996). Statistically significant inverse associations with dietary vitamin C or supplemental intake have been observed in a few case-control studies. Furthermore, no associations were observed in a large prospective cohort study examining intakes of vitamins C, E, and a total antioxidant score and risk of glioma (Michaud et al., 2009). Currently, evidence does not support causation for vitamins and risk of glioma.
As with vitamin intake, findings on fruit and vegetable intake have been inconsistent. Inconsistent results for fruit, vegetable, and vitamin intakes are not surprising; in addition to the problems inherent in case-control designs, the initial design of several studies was not to test a dietary hypothesis. Consequently, most were limited in their ability to capture dietary exposures. Furthermore, the majority of studies on diet and gliomas have limited statistical power, making it difficult to draw conclusions from those findings. In a large prospective cohort study, fruit and vegetable intake, overall or within subgroups, was not associated with risk of glioma (Holick et al., 2007).
Tobacco and Alcohol
The presence of nitrosamines in tobacco smoke has stimulated interest in tobacco exposure as a potential risk factor for primary brain tumors. Several case-control and cohort studies have assessed the relationship between cigarette smoking and the risk of adult glioma; few have reported any positive association with cigarette smoking. Overall, the 2004 Surgeon General’s Report on the Health Consequences of Smoking concluded that “the evidence is suggestive of no causal relationship between smoking cigarettes and brain cancer in men and women” (U.S. Department of Health and Human Services, 2004). Findings from studies of maternal smoking (active and passive) and risk of primary brain tumors in offspring have been inconsistent, but overall the data do not support an increase in brain tumor risk with maternal smoking (Norman et al., 1996).
Allergies
In a meta-analysis based on 3450 cases of glioma, a 39% decrease in risk of glioma was observed among those reporting any form of allergy (RR 0.61; 95% CI, 0.55-0.67); the association was similar for asthma or eczema (Linos et al., 2007). The association is weaker for meningiomas.
Two studies focused on allergies to specific exposures (e.g., medicine, foods) and reported inverse associations for most types of allergies (Brenner et al., 2002; Wiemels et al., 2002). In one of these studies (Brenner et al., 2002), self-report of any type of allergy was associated with a 33% reduction in the risk of glioma (OR 0.67), and the OR for individual allergies ranged from 0.23 (allergy to chemicals) to 0.97 (hay fever). In the other study (Wiemels et al., 2002), a dose-response relationship was observed between increasing numbers of specific allergens and risk of glioma, with an OR of 0.37 for five or more described allergens compared to none.
In a case-control study (Wiemels et al., 2004), measures of biological markers of allergies reduced the bias that arises from self-reporting in a case-control setting and increased the specificity of exposure assessment. Serum samples collected from 226 adult glioma cases and 289 age-gender-ethnicity frequency-matched controls measured immunoglobulin (Ig)E, a clinical marker of atopic allergies. Compared to normal levels (<25 kU/L), individuals with elevated IgE levels (>100 kU/L) had an OR of 0.37 (95% CI, 0.22-0.64), and individuals with intermediate IgE levels (25-100 kU/L) had an OR of 0.64 (95% CI, 0.42-0.96) for glioma risk. Chemotherapy, radiation, surgery, and medication use (including nonsteroidal antiinflammatory drugs, allergy drugs, steroids, and antibiotics) did not affect IgE levels in cases or controls in this study. A similar finding was observable in a follow-up study on IgE levels and risk of glioma, although the association was weaker than in the first study, and drug treatment might have been a confounding factor (Wiemels et al., 2009).
The high degree of consistency in all reports, despite differences in study design (hospital, friend, and population controls), percentage of proxy interviews, and geographical location of the study, suggests that an etiological basis for allergies may exist. Moreover, the fact that allergy was not a risk factor for meningioma in most studies (Brenner et al., 2002; Schlehofer et al., 1999) argues against bias as being responsible for the inverse association with glioma risk.
Infections
The detection of viruses and virus-like particles in brain tumor specimens has resulted in speculation that viral infections may be involved in brain tumor development. Interest in simian virus 40 (SV40), a polyomavirus, was stimulated by animal studies documenting brain tumor development after intracerebral inoculation with SV40 and human studies in which SV40 was isolated from brain tumor tissue. Accidental contamination of administered poliomyelitis vaccine with SV40 occurred between 1955 and 1962. Follow-up studies of these vaccinated individuals did not find an overall increase in the risk of brain tumors (Engels, 2005). Furthermore, no significant association was observed between antibodies to SV40 (or two other polyomaviruses, JC virus and BK virus) and primary malignant brain tumors when measured directly in serum collected prior to cancer diagnosis (Rollison et al., 2003).
Several studies have suggested that certain infections may reduce the risk of adult glioma. In one study (Schlehofer et al., 1999), subjects who reported a history of infectious diseases (e.g., colds, flu) had a 30% reduction in risk (RR 0.72; 95% CI, 0.61-0.85) compared to those with no such history. A possible protective role for antecedent infection with chickenpox (varicella-zoster virus) reported in two different case-control studies used a self-report and serological measures of the antibodies to varicella-zoster virus (Wrensch et al., 1997, 2001, 2005). In one analysis of 229 adults with glioma and 289 controls, individuals with a history of chickenpox had a lower risk of glioma (OR 0.59; 95% CI, 0.40-0.86), and individuals with higher levels of immunoglobulin directed against varicella-zoster virus were at lower risk of glioma (OR 0.41; 95% CI, 0.24-0.70, comparing highest to lowest quartile of serological IgG) (Wrensch et al., 2005). Similar associations were not seen with three other herpesviruses (Epstein-Barr virus, cytomegalovirus, and herpes simplex virus) (Wrensch et al., 2001, 2005).
Genetic Syndromes
Genetic syndromes that increase the risk of nervous system tumors cause approximately 1% to 5% of brain tumors (Kleihues et al., 2000). Neurofibromatosis type 1 (NF1; see Chapter 65) occurs in 1 in 4000 newborns and is linked to a gene on chromosome 17. Approximately 5% to 10% of persons with NF1 develop brain tumors, including malignant peripheral nerve sheath tumors, pilocytic astrocytomas of the optic nerve, and other astrocytomas. Neurofibromatosis type 2 (NF2) occurs in 1 in 40,000 newborns and has a corresponding link to a gene on chromosome 22. The presence of bilateral acoustic neuromas is the defining feature. Astrocytomas and other brain tumor types occur.
The Li-Fraumeni syndrome, an autosomal dominant condition, may involve a mutation in the tumor suppressor gene TP53, conferring an increased risk of soft-tissue sarcomas, osteosarcomas, breast cancer, and brain tumors. Approximately 12% of cancers in families with TP53 germline mutations are brain tumors; astrocytomas are the predominant type (Kleihues et al., 1997). Turcot syndrome is associated with adenomatous polyps and increased risk of medulloblastoma and glioblastoma. Persons with nevoid basal cell carcinoma (Gorlin syndrome) and Wilms tumor have an increased risk of medulloblastoma. Table 52A.1 summarizes inherited syndromes associated with an increased risk of nervous system tumors.
Genetic Polymorphisms
Recently, researchers have focused on genetic factors of brain cancers as a result of the increased availability of new technologies that allow rapid, effective, and affordable measurement of thousands of genetic variants. Genome-wide association studies (GWAS) involve the analysis of genetic variants in the whole genome and their relation to disease onset. Several GWAS have been conducted for glioma, providing new clues into possible genetic regions that may play a role in this disease. In one study, 5 susceptibility loci in genes TERT, CCDC26, CDKN2A/CDKN2B, RTEL1, and PHLDB1 were identified by genotyping 550K tagging single-nucleotide polymorphisms (SNPs) in a total of 2545 glioma cases and 2953 controls (Shete et al., 2009). In another study, the identification of 2 susceptibility loci (CDKN2B and RTEL1) was based on the analysis of 275,895 SNPs in 692 adult high-grade glioma cases and 174 controls (Wrensch et al., 2009). More GWAS currently underway may reveal additional new genetic regions important for glioma. Genetic polymorphisms in specific detoxification/metabolic enzymes have been implicated in the pathogenesis of certain cancers. The association between chemical carcinogens and brain tumors in experimental models raises the possibility that susceptibility may be related to genetic polymorphisms at loci encoding phase I cytochrome P450 (CYP450) and phase II glutathione S-transferase (GST) enzymes. Several potential neurocarcinogens (polycyclic aromatic hydrocarbons, nitrosoureas, and methyl halide) are substrates for these enzymes. Several case-control studies of brain tumors have assessed polymorphic enzymes (e.g., GSTT1, GSTM1, GSTP1, CYP2D6, NAT2, HRAS1) implicated in the activation or detoxification of potential neurocarcinogens; results from these studies are inconsistent. A meta-analysis of eight studies concluded that no evidence exists for an association between GST variants and risk of glioma (Lai et al. 2005). Other studies have been conducted on genetic polymorphisms involved in DNA repair, immune function, inflammation and allergies; results from these studies need to be replicated.
Ahlbom I.C., Cardis E., Green A., et al. Review of the epidemiologic literature on EMF and health. Environ Health Perspect. 2001;109(6):911-933.
Ahlbom A., Feychting M., Green A., et al. Epidemiologic evidence on mobile phones and tumor risk: a review. Epidemiology. 2009;20:639-652.
American Cancer Society. 2010. Cancer Facts & Figures 2010, Atlanta, American Cancer Society.
Blowers L., Preston-Martin S., Mack W.J. Dietary and other lifestyle factors of women with brain gliomas in Los Angeles County (California, USA). Cancer Causes Control. 1997;8:5-12.
Bondy M.L., Wang L.E., El-Zein R., et al. Gamma-radiation sensitivity and risk of glioma. J Natl Cancer Inst. 2001;93:1553-1557.
Brain tumour risk in relation to mobile telephone use. Jun 2010. results of the INTERPHONE international case-control study. Int J Epidemiol. Jun 2010;39(3):675-694.
Brenner A.V., Linet M.S., Fine H.A., et al. History of allergies and autoimmune diseases and risk of brain tumors in adults. Int J Cancer. 2002;99:252-259.
Brownson R.C., Reif J.S., Chang J.C., et al. An analysis of occupational risks for brain cancer. Am J Public Health. Feb 1990;80(2):169-172.
Carozza S.E., Wrensch M., Miike R. Occupation and adult gliomas. Am J Epidemiol. 2000;152:838-846.
CBTRUS Statistical Report, 2010. Primary Brain and Central Nervous System Tumors Diagnosed in the United States in 2004-2006: Central Brain Tumor Registry of the United States.
Christensen H.C., Schuz J., Kosteljanetz M., et al. Cellular telephone use and risk of acoustic neuroma. Am J Epidemiol. 2004;159:277-283.
Christensen H.C., Schuz J., Kosteljanetz M., et al. Cellular telephones and risk for brain tumors: a population-based, incident case-control study. Neurology. 2005;64:1189-1195.
Dietrich M., Block G., Pogoda J.M., et al. A review: dietary and endogenously formed N-nitroso compounds and risk of childhood brain tumors. Cancer Causes Control. 2005;16:619-635.
Edwards C.G., Schwartzbaum J.A., Lonn S., et al. Exposure to loud noise and risk of acoustic neuroma. Am J Epidemiol. 2006;163:327-333.
Engels E.A. Cancer risk associated with receipt of vaccines contaminated with simian virus 40: epidemiologic research. Expert Rev Vaccines. 2005;4:197-206.
Fine H.A., Loeffler J.S. Primary Central Nervous System. Philadelphia: WB Saunders; 1999.
Gousias K., Markou M., Voulgaris S., et al. Descriptive epidemiology of cerebral gliomas in northwest Greece and study of potential predisposing factors, 2005-2007. Neuroepidemiology. 2009;33(2):89-95.
Gurney J.G, Preston-Martin S., McDaniel A.M., et al. Head injury as a risk factor for brain tumors in children: results from a multicenter case-control study. Epidemiology. 1996;7(5):485-489.
Haldorsen I.S., Krossnes B.K., Aarseth J.H., et al. Increasing incidence and continued dismal outcome of primary central nervous system lymphoma in Norway 1989-2003: time trends in a 15-year national survey. Cancer. 2007;110(8):1803-1814.
Holick C.N., Giovannucci E.L., Rosner B., et al. Prospective study of intake of fruit, vegetables, and carotenoids and the risk of adult glioma. Am J Clin Nutr. 2007;85(3):877-886.
Huncharek M., Kupelnick B., Wheeler L. Dietary cured meat and the risk of adult glioma: a meta-analysis of nine observational studies. J Environ Pathol Toxicol Oncol. 2003;22:129-137.
Inskip P.D., Mellemkjaer L., Gridley G., et al. Incidence of intracranial tumors following hospitalization for head injuries (Denmark). Cancer Causes Control. 1998;9:109-116.
Inskip P.D., Tarone R.E., Hatch E.E., et al. Cellular-telephone use and brain tumors. N Engl J Med. 2001;344:79-86.
Johansen C., Boice J.Jr., McLaughlin J., et al. Cellular telephones and cancer—a nationwide cohort study in Denmark. J Natl Cancer Inst. 2001;93:203-207.
Jukich P.J., McCarthy B.J., Surawicz T.S., et al. Trends in incidence of primary brain tumors in the United States, 1985-1994. Neuro Oncol. 2001;3:141-151.
Khuder S.A., Mutgi A.B., Schaub E.A. Meta-analyses of brain cancer and farming. Am J Ind Med. 1998;34:252-260.
Kleihues P., Burger P.C., Collins V.P., et al. Tumours of the Nervous System. Lyon, France: IARC; 2000.
Kleihues P., Schauble B., zur Hausen A., et al. Tumors associated with p53 germline mutations: a synopsis of 91 families. Am J Pathol. 1997;150:1-13.
Lai K.C., Chen W.C., Jeng L.B., et al. Association of genetic polymorphisms of MK, IL-4, p16, p21, p53 genes and human gastric cancer in Taiwan. Eur J Surg Oncol. 2005;31(10):1135-1140.
La Vecchia C., Franceschi S. Nutrition and gastric cancer. Can J Gastroenterol. 2000;14(D):51D-54D.
Lee M., Wrensch M., Miike R. Dietary and tobacco risk factors for adult onset glioma in the San Francisco Bay Area (California, USA). Cancer Causes Control. 1997;8:13-24.
Lijinsky W. N-Nitroso compounds in the diet. Mutat Res. 1999;443:129-138.
Linos E., Raine T., Alonso A., et al. Atopy and risk of brain tumors: a meta-analysis. J Natl Cancer Inst. 2007;99(20):1544-1550.
Longstreth W.T.Jr., Phillips L.E., Drangsholt M., et al. Dental x-rays and the risk of intracranial meningioma: a population-based case-control study. Cancer. 2004;100:1026-1034.
Lonn S., Ahlbom A., Hall P., et al. Long-term mobile phone use and brain tumor risk. Am J Epidemiol. 2005;161:526-535.
Louis D.N., Ohgaki H., Wiestler O.D., et al. The 2007 WHO classification of tumours of the central nervous system (vol 114, pg 97, 2007). Acta Neuropathol. 114(5), 2007. 547–547
Makino K., Nakamura H., Kino T., et al. Rising incidence of primary central nervous system lymphoma in Kumamoto, Japan. Surg Neurol. 2006;66(5):503-506.
McLaughlin J.K., Malker H.S., Blot W.J., et al. Occupational risks for intracranial gliomas in Sweden. J Natl Cancer Inst. 1987;78:253-257.
Michaud D.S., Holick C.N., Batchelor T.T., et al. Prospective study of meat intake and dietary nitrates, nitrites, and nitrosamines and risk of adult glioma. Am J Clin Nutr. 2009;90(3):570-577.
Neugut A.I., Hayek M., Howe G. Epidemiology of gastric cancer. Semin Oncol. 1996;23:281-291.
Norman M.A., Holly E.A., Preston-Martin S. Childhood brain tumors and exposure to tobacco smoke. Cancer Epidemiol Biomarkers Prev. 1996;5:85-91.
Nygren C., Adami J., Ye W., et al. Primary brain tumors following traumatic brain injury—a population-based cohort study in Sweden. Cancer Causes Control. 2001;12:733-737.
Parkin D.M., Bray F., Ferlay J., et al. Global cancer statistics, 2002. CA Cancer J Clin. 2005;55:74-108.
Preston-Martin S., Mack W., Henderson B.E. Risk factors for gliomas and meningiomas in males in Los Angeles County. Cancer Res. 1989;49:6137-6143.
Preston-Martin S., Pogoda J.M., Schlehofer B., et al. An international case-control study of adult glioma and meningioma: the role of head trauma. Int J Epidemiol. 1998;27:579-586.
Ries L., Eisner M., Kosary C., et al. SEER Cancer Statistics Review, 1975-2003, In. Bethesda, MD: National Cancer Institute. 2006.
Rollison D.E., Helzlsouer K.J., Alberg A.J., et al. Serum antibodies to JC virus, BK virus, simian virus 40, and the risk of incident adult astrocytic brain tumors. Cancer Epidemiol Biomarkers Prev. 2003;12:460-463.
Roman G.C. Gene-environment interactions in the Italy-Argentina “Colombo 2000” project. Funct Neurol. 1998;13:249-252.
Ron E., Modan B., Boice J.D.Jr., et al. Tumors of the brain and nervous system after radiotherapy in childhood. N Engl J Med. 1988;319:1033-1039.
Rothman K.J., Loughlin J.E., Funch D.P., et al. Overall mortality of cellular telephone customers. Epidemiology. 1996;7:303-305.
Rubenstein J., Ferreri A.J., Pittaluga S. Primary lymphoma of the central nervous system: epidemiology, pathology and current approaches to diagnosis, prognosis and treatment. Leuk Lymphoma. 2008;49(1)):43-51.
Ruder A.M., Carreon T., Butler M.A., et al. Exposure to farm crops, livestock, and farm tasks and risk of glioma. Am J Epidemiol. 2009;169:1479-1491.
Sadetzki S., Chetrit A., Freedman L., et al. Long-term follow-up for brain tumor development after childhood exposure to ionizing radiation for tinea capitis. Radiat Res. 2005;163:424-432.
Samanic C.M., De Roos A.J., Stewart P.A., et al. Occupational exposure to pesticides and risk of adult brain tumors. Am J Epidemiol. 2008;167(8):976-985.
Samkange-Zeeb F., Schlehofer B., Schuz J., et al. Occupation and risk of glioma, meningioma and acoustic neuroma: results from a German case-control study (interphone study group, Germany). Cancer Epidemiol. 2010;34(1):55-61.
Saracci R., Samet J. Commentary: call me on my mobile phone or better not?—a look at the INTERPHONE study results. Int J Epidemiol. 2010;39(3):695-698.
Savitz D.A., Cai J., van Wijngaarden E., et al. Case-cohort analysis of brain cancer and leukemia in electric utility workers using a refined magnetic field job-exposure matrix. Am J Ind Med. 2000;38:417-425.
Savitz D.A., Loomis D.P. Magnetic field exposure in relation to leukemia and brain cancer mortality among electric utility workers. Am J Epidemiol. 1995;141:123-134.
Schlehofer B., Blettner M., Preston-Martin S., et al. Role of medical history in brain tumour development. Results from the International Adult Brain Tumour Study. Int J Cancer. 1999;82:155-160.
Schoemaker M.J., Swerdlow A.J., Ahlbom A., et al. Mobile phone use and risk of acoustic neuroma: results of the Interphone case-control study in five North European countries. Br J Cancer. 2005;93:842-848.
SEER Cancer Statistics Review. 1975-2006 http://seer.cancer.gov/csr/1975_2006/, 2009.
SEER Cancer Statistics Review. 1975-2007 http://seer.cancer.gov/csr/1975_2007/index.html, 2010.
Shete S., Hosking F.J., Robertson L.B., et al. Genome-wide association study identifies five susceptibility loci for glioma. Nat Genet. 2009;41(8):899-904.
State Cancer Profiles, http://statecancerprofiles.cancer.gov/index.html, 2010.
U.S. Department of Health and Human Services, Office of the Surgeon General. The Health Consequences of Smoking: a Report of the Surgeon General 2004. Washington, DC: DHHS; 2004.
Wiemels J.L., Wiencke J.K., Patoka J., et al. Reduced immunoglobulin E and allergy among adults with glioma compared with controls. Cancer Res. 2004;64:8468-8473.
Wiemels J.L., Wiencke J.K., Sison J.D., et al. History of allergies among adults with glioma and controls. Int J Cancer. 2002;98:609-615.
Wiemels J.L., Wilson D., Patil C., et al. IgE, allergy, and risk of glioma: update from the San Francisco Bay Area Adult Glioma Study in the temozolomide era. Int J Cancer. 2009;125(3):680-687.
Wrensch M., Jenkins R.B., Chang J.S., et al. Variants in the CDKN2B and RTEL1 regions are associated with high-grade glioma susceptibility. Nat Genet. 2009;41(8):905-908.
Wrensch M., Miike R., Lee M., et al. Are prior head injuries or diagnostic x-rays associated with glioma in adults? The effects of control selection bias. Neuroepidemiology. 2000;19:234-244.
Wrensch M., Weinberg A., Wiencke J., et al. Does prior infection with varicella-zoster virus influence risk of adult glioma? Am J Epidemiol. 1997;145:594-597.
Wrensch M., Weinberg A., Wiencke J., et al. Prevalence of antibodies to four herpesviruses among adults with glioma and controls. Am J Epidemiol. 2001;15:161-165.
Wrensch M., Weinberg A., Wiencke J., et al. History of chickenpox and shingles and prevalence of antibodies to varicella-zoster virus and three other herpesviruses among adults with glioma and controls. Am J Epidemiol. 2005;161:929-938.