CHAPTER 11 Biochemical Genetics
In this chapter, we consider single-gene biochemical or metabolic diseases, including mitochondrial disorders. The range of known disorders is vast, so only an overview is possible, but it is hoped that the reader will gain a flavor of this fascinating area of medicine. At the beginning of the twentieth century, Garrod introduced the concept of ‘chemical individuality’, leading in turn to the concept of the inborn error of metabolism (IEM). Beadle and Tatum later developed the idea that metabolic processes, whether in humans or any other organism, proceed by steps. They proposed that each step was controlled by a particular enzyme and that this, in turn, was the product of a particular gene. This was referred to as the one gene–one enzyme (or protein) concept.
Inborn Errors of Metabolism
In excess of 200 IEMs are known that can be grouped by either the metabolite, metabolic pathway, function of the enzyme, or cellular organelle involved (Table 11.1). Most follow autosomal recessive or X-linked recessive inheritance, with only a few being autosomal dominant. This is because the defective protein in most cases is a diffusible enzyme, and there is usually sufficient residual activity in the heterozygous state (loss-of-function, see p. 26) for the enzyme to function normally in most situations. If, however, the reaction catalysed by an enzyme is rate limiting (haploinsufficiency, see p. 26) or the gene product is part of a multimeric complex (dominant-negative, see p. 26), the disorder can manifest in the heterozygous state and follow dominant inheritance (p. 109).
Disorders of Amino Acid Metabolism
Phenylketonuria
Children with phenylketonuria (PKU), if untreated, are severely intellectually impaired and often develop seizures. There is a deficiency of the enzyme required for the conversion of phenylalanine to tyrosine, phenylalanine hydroxylase (PAH)—causing a ‘genetic block’ in the metabolic pathway (Figure 11.1).
PKU was the first genetic disorder in humans shown to be caused by a specific enzyme deficiency, by Jervis in 1953. As a result of the enzyme defect, phenylalanine accumulates and is converted into phenylpyruvic acid and other metabolites that are excreted in the urine. The enzyme block leads to a deficiency of tyrosine, with a consequent reduction in melanin formation, and children therefore often have blond hair and blue eyes (Figure 11.2). In addition, areas of the brain that are usually pigmented, such as the substantia nigra, may also lack pigment.
Treatment of PKU
An obvious method of treating children with PKU would be to replace the missing enzyme, but this is not simply achieved (p. 349). Bickel, just 1 year after the enzyme deficiency had been identified, suggested that PKU could be treated by removal of phenylalanine from the diet and this has proved effective. If PKU is detected early enough in infancy, intellectual impairment can be prevented by giving a phenylalanine restricted diet. Phenylalanine is an essential amino acid and therefore cannot be removed entirely from the diet. By monitoring the level of phenylalanine in the blood, it is possible to supply sufficient amounts to meet normal requirements but avoiding toxic levels, resulting in mental retardation. After brain development is complete, dietary restriction can be relaxed—from adolescence onward.
Diagnosis of PKU
PKU affects approximately 1 in 10,000 people in western Europe and was the first IEM routinely screened for in newborns. The test detects the presence of the metabolite of phenylalanine, phenylpyruvic acid, in the urine by its reaction with ferric chloride, or through increased levels of phenylalanine in the blood. The latter, known as the Guthrie test, involved analysing blood from newborns and comparing the amount of growth induced by the sample, against standards, in a strain of the bacterium Bacillus subtilis, which requires phenylalanine for growth. This has been replaced by the use of a variety of biochemical assays of phenylalanine levels.
Mutational Basis of PKU
More than 450 different mutations in the PAH gene have been identified. Certain mutations are more prevalent in specific population groups and, in western Europeans with PKU, they are found on the background of a limited number of DNA haplotypes. Interestingly though, a variety of different individual mutations has been found in association with some of these haplotypes.
Alkaptonuria
Alkaptonuria was the original autosomal recessive IEM described by Garrod. Here there is a block in the breakdown of homogentisic acid, a metabolite of tyrosine, because of a deficiency of the enzyme homogentisic acid oxidase (see Figure 11.1). As a consequence, homogentisic acid accumulates and is excreted in the urine, which then darkens on exposure to air. Dark pigment is also deposited in certain tissues, such as the ear wax, cartilage, and joints, where it is known as ochronosis, which in joints can lead to arthritis later in life.
Oculocutaneous Albinism
Oculocutaneous albinism (OCA) is an autosomal recessive disorder resulting from a deficiency of the enzyme tyrosinase, which is necessary for the formation of melanin from tyrosine (see Figure 11.1). In OCA there is a lack of pigment in the skin, hair, iris, and ocular fundus (Figure 11.3), and the lack of eye pigment results in poor visual acuity and uncontrolled pendular eye movements—nystagmus. Reduced fundal pigmentation leads to underdevelopment of part of retina for fine vision—the fovea—and abnormal projection of the visual pathways to the optic cortex.
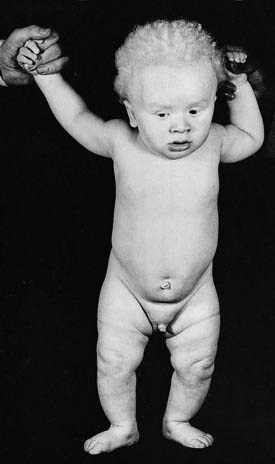
FIGURE 11.3 Oculocutaneous albinism in a child of Afro-Caribbean origin.
(Courtesy of Dr V. A. McKusick.)
Homocystinuria
Homocystinuria is a recessively inherited sulfur amino-acid IEM characterized by learning disability, seizures, thrombophilia, osteoporosis, scoliosis, pectus excavatum, long fingers and toes (arachnodactyly), and a tendency to dislocation of the lenses. The somatic features therefore resemble the autosomal dominant disorder Marfan syndrome (p. 300).
Urea Cycle Disorders
The urea cycle is a five-step metabolic pathway that takes place primarily in liver cells for the removal of waste nitrogen from the amino groups of amino acids arising from the normal turnover of protein. It converts two molecules of ammonia and one of bicarbonate into urea (Figure 11.4). Deficiencies of enzymes in the urea cycle result in intolerance to protein from the accumulation of ammonia in the body—hyperammonemia. Increased ammonia levels are toxic to the central nervous system and can lead to coma and, with some untreated urea cycle disorders, death. They are collectively and individually rare and, with the exception of X-linked ornithine transcarbamylase deficiency, inherited as autosomal recessive traits.
Disorders of Carbohydrate Metabolism
Glycogen Storage Diseases
Glycogen Storage Diseases that Primarily Affect Liver
Glycogen Storage Diseases that Primarily Affect Muscle
Pompe disease (GSD-II)
Infants with Pompe disease usually present in the first few months of life with floppiness (hypotonia) and delay in the gross motor milestones because of muscle weakness. They then develop an enlarged heart and die from cardiac failure in the first or second year. Voluntary and cardiac muscle accumulates glycogen because of a deficiency of the lysosomal enzyme α-1,4-glucosidase, which is needed to break down glycogen. The diagnosis can be confirmed by enzyme assay of white blood cells or fibroblasts. Early reports of enzyme replacement therapy appear promising.
Disorders of Steroid Metabolism
The disorders of steroid metabolism include a number of autosomal recessive inborn errors of the biosynthetic pathways of cortisol. Virilization of a female fetus may occur together with salt loss in infants of either sex from a deficiency of the hormone aldosterone. In addition, defects of the androgen receptor result in lack of virilization of chromosomally male individuals (Figure 11.5).
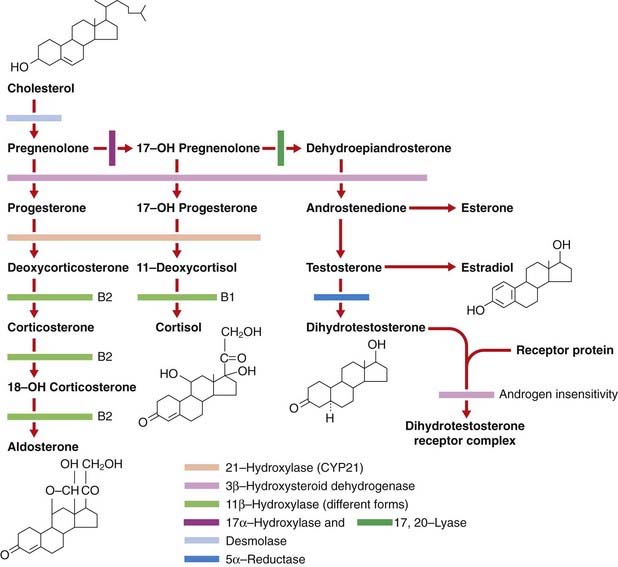
FIGURE 11.5 Steroid biosynthesis indicating the site of the common inborn errors of steroid biosynthesis.
Congenital Adrenal Hyperplasia (Adrenogenital Syndrome)
The diagnosis of congenital adrenal hyperplasia (CAH) should be considered in any newborn female infant presenting with virilization of the external genitalia, because this is the most common cause of ambiguous genitalia in female newborns (p. 288) (Figure 11.6). 21-Hydroxylase deficiency accounts for more than 90% of cases. Approximately 25% have the salt-losing form, presenting in the second or third week of life with circulatory collapse, hyponatremia, and hyperkalemia. Less commonly, CAH is a result of deficiency of the enzymes 11β-hydroxylase or 3β-dehydrogenase, and very rarely occurs as a result of deficiencies of enzymes 17α-hydroxylase and 17,20-lyase. Desmolase deficiency is very rare, with all pathways blocked, causing a reversed phenotype of ambiguous genitalia in males, and severe addisonian crises. Males with the rare 5α-reductase deficiency are significantly under-masculinized but do not suffer other metabolic problems and are likely to be raised as females. At puberty, however, the surge in androgen production is sufficient to stimulate growth of the phallus, making gender identity and assignment problematic.
Affected females with classic CAH are virilized from accumulation of the adrenocortical steroids proximal to the enzyme block in the steroid biosynthetic pathway, many of which have testosterone-like activity (see Figure 11.5