Chapter 53 Basic Principles of Deep Brain Stimulation for Movement Disorders, Neuropsychiatric Disorders, and New Frontiers
• The spectrum of movement disorders treated with deep brain stimulation (DBS) includes Parkinson’s disease (PD), essential tremor, and dystonia. The cardinal features of PD are resting tremor, bradykinesia, rigidity, and postural instability. Replacement of dopamine via dopamine agonists and L-dopa is the mainstay of medical treatment. DBS is the neurosurgical therapy of choice for movement disorders. DBS can improve motor symptoms, decrease medication usage, and decrease on/off motor fluctuations in patients with good response to L-dopa.
• In choosing an appropriate target for neuromodulation by DBS, it is important to note that multiple targets can be efficacious for a single disease. For example, Parkinson’s disease responds well to DBS of the globus pallidus interna (GPi) and subthalamic nucleus (STN).
• Deep brain stimulation of the ventralis intermedius nucleus of the thalamus can be used to treat patients with disabling essential tremor. Extremity tremors are more reliably treated than axial tremors.
• Primary generalized and focal dystonias can be treated with deep brain stimulation of the GPi. Some secondary dystonias may respond to deep brain stimulation; however, this is a heterogeneous group of disorders and response to deep brain stimulation may be the exception rather than the rule.
• Deep brain stimulation of the ventral capsule/ventral striatum is approved under a Food and Drug Administration (FDA) humanitarian device exemption for the treatment of severe, treatment-refractory obsessive-compulsive disorder. Active investigation of deep brain stimulation for neuropsychiatric disorders, pain, epilepsy, and others is currently ongoing.
• The pharmacological therapies available for dystonia are limited; therefore, surgical alternatives have been developed. These include intrathecal baclofen pumps, botulinum toxin injections, ablative procedures such as thalamotomy, and neuromodulation procedures such as DBS. The GPi is the DBS “target of choice” for “primary generalized dystonia.”
• Careful selection of appropriate patients is critical to good outcomes in DBS procedures.
• DBS for movement and neuropsychiatric disorders should be performed in the context of a multidisciplinary team.
• In common movement disorders, surgical targets are the STN and GPi for Parkinson’s disease, ventralis intermedius nucleus of the thalamus for essential tremor, GPi for dystonia, and the ventral capsule/ventral striatum (VC/VS) target for neuropsychiatric disorders such as depression and obsessive-compulsive disorder (OCD).
• Targeting of subcortical nuclei in DBS is based on stereotactic principles.
Historical Background
Deep brain stimulation (DBS) is now considered the neurosurgical therapy of choice for movement disorders, with over 80,000 patients having undergone DBS worldwide.1 DBS works through the delivery of therapeutic electrical energy to highly specific targets in the brain. The safety and efficacy of DBS have been validated in many high-quality studies in over 20 years of use. DBS is also the subject of intense intellectual study; a recent PubMed search for “deep brain stimulation” returned over 3500 published studies.2 A particularly exciting aspect of the ongoing research in DBS is evaluation of expanding indications for the therapy, which span neuropsychiatric disorders, epilepsy, and disorders of consciousness, among others. This chapter reviews the historical underpinnings of DBS, indications for use, and future horizons. Additionally, a brief description of DBS components and purported mechanisms of action will be provided.
Historical Beginnings
The modern era of deep brain stimulation has its historical roots in the development of stereotactic surgery, which has its own origins in the work of the French mathematician and philosopher René Descartes. In part II of his 1637 work, Discourse on the Method, Descartes describes a method for specifying the location of a point based on its relationship to two intersecting axes.3 (Of note, this work is best known for its famous quotation, “Je pense, donc je suis” [I think, therefore I am], which occurs in part IV.) This method, known as the Cartesian coordinate system, facilitated the development of calculus by Isaac Newton and Gottfried Wilhelm Leibniz as well as modern stereotactic neurosurgery.
The happy marriage of the fields of stereotaxy, movement disorder surgery, and psychosurgery gave rise to the underlying principles of modern DBS surgery. On the movement disorders front, much work had been done on cortical resections and subcortical lesioning to palliate these disorders. In 1909, Sir Victor Horsley, who described the first stereotactic apparatus in animals, relieved hemiathetosis in a 15-year-old boy by removing the precentral gyrus somatotopically associated with the upper extremity.4 In 1931, Bucy performed the same procedure for seizure relief and relieved the patient’s choreoathetosis in the process.5 He later, in 1937, performed this procedure with Theodore Case for the treatment of post-traumatic tremor.6 These patients had postoperative hemiparesis and dyspraxia that resolved to a great extent postoperatively.7 In 1931, Putnam began sectioning of the extrapyramidal tracts in the high cervical cord, with good results for choreoathetosis but not with parkinsonian tremor.8,9 Given that cortical ablation would relieve tremor, but extrapyramidal ablation would not, he reasoned that impulses in the pyramidal tract were responsible for the production of parkinsonian tremor, and in 1940, he reported the results of lateral pyramidotomy in the high cervical region in seven patients.10 Again, these patients had postoperative improvement of tremor but some resultant hemiplegia.
The search for a suitable procedure to maximize tremor control yet minimize postoperative morbidity led to cerebral pedunculotomy by Walker;11 open, transcortical, transventricular removal of portions of the basal ganglia by Meyers;12 and anterior choroidal artery ligation by Cooper.13–15 (As an aside, Cooper’s procedure was discovered accidentally when he tore the anterior choroidal artery during an aborted cerebral pedunculotomy.) These procedures, while therapeutic, were both variable in their outcomes and suffered from high perioperative morbidity rates, thereby necessitating more precise and less invasive targeting.
Furthermore, proponents of psychosurgery wanted a means for performing controlled prefrontal lobotomy.16 In the 1940s, there was concern among neurosurgeons regarding the high morbidity and mortality rates associated with open psychosurgical procedures including prefrontal leucotomy as introduced by Egas Moniz and prefrontal lobotomy as introduced by Walther Freeman and James Watts.17 There was a general feeling that the principles of psychosurgery were sound, but smaller and more specific structures should be targeted to achieve optimal therapeutic benefit while avoiding unnecessary morbidity and unwanted neurological deficit.
Initial attempts of stereotactic surgery began in the late nineteenth and early twentieth centuries. In 1889, D.N. Zernov, a Russian surgeon in Moscow, used a navigation system (called an encephalometer) based on polar coordinates and referenced to external landmarks to drain a cerebral abscess.18,19 The first truly modern stereotactic apparatus based on Cartesian coordinates and based on internal cerebral landmarks was first described in animals in the early part of the twentieth century by Sir Victor Horsley and Robert Clarke. In 1908, they described a stereotactic apparatus that could introduce a probe into subcortical structures defined in a Cartesian coordinate system.20
The first device developed for inserting an electrode accurately into a chosen structure in the human brain was described by Ernest A. Spiegel, Henry T. Wycis, and others in 194721 (Fig. 53.1). At that time, cerebral anatomical structures were targeted based on location relative to the third ventricle using ventriculogram x-rays. Electrode position in the apparatus of Spiegel and Wycis was adjusted by sliding the carrier along a base plate in the anteroposterior and lateral directions, with vertical adjustments made by a microdrive. Leksell introduced a semicircular arc apparatus in 1949, allowing for the introduction of an electode to a target along any trajectory, because the target now would lie in the center of the arc22 (Fig. 53.2).
The first lesioning procedures performed by Spiegel and Wycis were in the dorsomedial thalamic nucleus for agitation and psychosis, the medial thalamus for epilepsy, and mesencephalic pain pathways for intractable pain.7,23 With the advent of stereotactic lesioning procedures, many new techniques were developed for the management of psychiatric and movement disorders. These included pallidotomy and thalamotomy for the relief of Parkinson’s disease (PD)–associated tremor,24–29 as well as anterior cingulotomy,30,31 anterior capsulotomy,32 subcaudate tractotomy,33 and limbic leucotomy34 in the treatment of affective disorders7,17,35,36Fig. 53.3. However, with the advent of L-dopa in the 1960s for PD, there was a steep decrease in surgery for PD.37 Similarly, the introduction of lithium in the late 1940s and the antipsychotic drug chlorpromazine in the mid-1950s led to a nonsurgical bend in the management of psychiatric disorders.17,36,38 Therefore, the next paradigm shift in the treatment of these disorders did not come until the limitations of these medications with their side effects and lack of sustained benefits were realized, and DBS provided a safer, nondestructive, reversible, and adjustable approach for the treatment of these disorders.
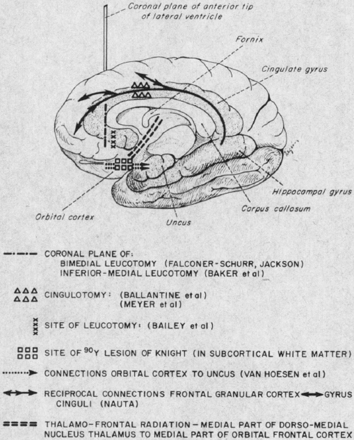
FIGURE 53.3 Classical drawing depicting locations of stereotactic lesioning procedures for psychosurgery.
(Reprinted from Sweet WH. Treatment of medically intractable mental disease by limited frontal leucotomy—justifiable? N Engl J Med. 1973;289(21):1117-1125; used with permission of the Massachusetts Medical Society.)
In 1809, Rolando first demonstrated that electrical impulses could modify the function of brain regions, and prior to that, Aldini attempted stimulation of the brains of criminals immediately following their executions.37,39 Hassler found, during stereotactic exploration of the brain for pallidotomy, that low-frequency stimulation would worsen tremor and high-frequency stimulation would ameliorate it.37,40 This was first put into practice in 1967 by Bechtereva and associates with chronic DBS of the thalamus, striatum, and pallidum for the treatment of movement disorders.41
It was not until the 1970s and 1980s, however, that the modern era of chronic stimulation was ushered in. Hosobuchi and co-workers reported on stimulation of the VPM thalamus for the control of facial anesthesia dolorosa following rhizotomy for trigeminal neuralgia in 1973.42 DBS of ventral periaqueductal gray matter in the midbrain for the treatment of cancer pain was reported by Richardson and Akil in the mid-1970s.43 Chronic DBS for the management of movement disorders was utilized by several groups including Benabid and colleagues,44,45 Blond and Siegfried,46 Siegfried and Shulman,47 and Brice and McLellan.48 DBS has similar efficacy to its corresponding lesioning procedures, but with an improved safety profile, inherent reversibility, and adjustment of the stimulation parameters over time to accommodate the patient’s needs. These factors have led to DBS being adopted as the neurosurgical standard of care for movement disorders as well as the expansion of DBS to the treatment of epilepsy, psychiatric disorders including obsessive-compulsive disorder (OCD) and depression, as well as chronic pain, Tourette’s syndrome, and even disorders of consciousness and addiction.
Basic Neurocircuitry Principles
Parallel cortico-striato-pallido-thalamo-cortical (CSPTC) circuits exist for limbic, associative, and motor function.49 The motor loop is the most commonly described loop and is important in the pathogenesis of PD and the therapeutic efficacy of DBS in this condition.37 Limbic and associative circuits have been implicated in the pathogenesis of neuropsychiatric conditions including OCD and major depressive disorder (MDD), which respond to DBS of critical nodes along the circuit, including the ventral capsule/ventral striatum (VC/VS), anterior limb of the internal capsule (ALIC), nucleus accumbens (NAc), subgenual cingulate cortex (SCC), and inferior thalamic peduncle.49–61 These circuits maintain some degree of topographic segregation, with motor circuitry more dorsolateral, limbic circuitry ventromedial, and associative circuitry in between.62
These circuits are not strictly “parallel.” Haber and associates demonstrated some interface between these circuits in primates with the more medial limbic circuits ultimately influencing more dorsolateral motor circuits.62 This unique arrangement allows for a link between emotion and motivation (limbic circuits) with cognition and planning (associative circuits), which finally manifests with a motor output and behavior (motor circuits).
Both direct and indirect loops exist for the associative and limbic circuits, as they do for the motor circuits.49 The direct loops connect from the striatum to the globus pallidus pars interna (GPi), substantia nigra pars reticulata (SNr), and ventral tegmental area (VTA). From there, the projections head to the thalamus. Indirect loops connect from the striatum to the globus pallidus pars externa (GPe), and then head to the subthalamic nucleus (STN) before going to the GPi, SNr, and VTA (Fig. 53.4).
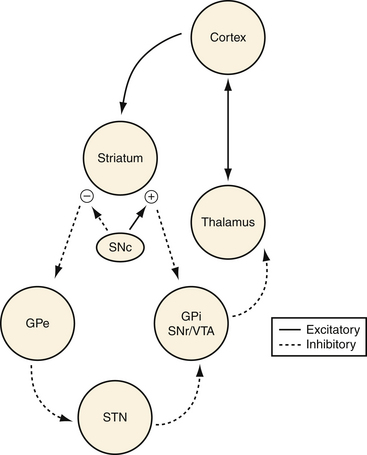
FIGURE 53.4 Schematic of relevant cortico-striato-pallido-thalamo-cortical (CSPTC) neural circuitry.
In choosing an appropriate target for neuromodulation by DBS, it is important to note that multiple targets can be efficacious for a single disease. For example, we know that PD responds well to DBS of the GPi and subthalamic nucleus.37,63 In psychosurgery, multiple targets have been used, for example, in OCD including the ALIC,52,59 VC/VS and NAc,51,57 inferior thalamic peduncle,56 and subthalamic nucleus.58 This is likely secondary to the rich connectivity of the modulated circuits and the mechanism of action of DBS. High-frequency stimulation (HFS) has a net inhibitory effect and low-frequency stimulation (LFS) has a net excitatory effect; however, the mechanism of DBS (high-frequency) is likely more complex with activation of nearby axons and suppression of abnormal impulses through the circuits involved.37
Deep Brain Stimulation Surgery: Indications, Target, and Outcomes
All patients should undergo detailed neuropsychological assessment. The aim is to identify patients who have underlying cognitive deficits or psychiatric conditions that may be worsened by DBS. Severe dementia or cognitive impairment is generally a contraindication to DBS, and patients with even mild deficits in frontal executive function or cognition need especially strong social support following surgery. Psychiatric conditions as depression, mania, and anxiety should be identified and optimized preoperatively, and patients with psychosis or personality disorders should generally be excluded from surgical intervention.37 These factors underscore the importance of a multidisciplinary team devoted to the care of DBS patients. (Selection criteria are summarized in Table 53.1.)
TABLE 53.1 General Principles of Patient Selection in Deep Brain Stimulation Surgery: Predictors of Successful Outcome
Surgical Technique
Preoperative Imaging and Planning
Stereotactic anatomical targeting may be by indirect or direct methods. Indirect targeting utilizes stereotactic atlases based on cadaveric sections. Known distances of the different nuclei from the midcommissural point (MCP), the halfway distance between the anterior commissure (AC) and posterior commissure (PC), are used in determining the position of the anatomical target. Typical anatomical coordinates for the common targets are as follows: STN (10-13 mm lateral to midline, 3-5 mm ventral to the AC-PC plane, and 2-4 mm posterior to the MCP), the GPi (19-21 mm lateral to the midline, 2-3 mm anterior to the MCP, and 4-5 mm ventral to the AC-PC plane), the ventralis intermedius (VIM) (11-12 mm lateral to the wall of the third ventricle, at the level of the AC-PC plane and between 2⁄12 and 3⁄12 of the AC-PC distance anterior to the PC). Table 53.2 shows the typical stereotactic targets for different indications as well as their stereotactic locations with reference to the AC, PC, and MCP.
TABLE 53.2 Deep Brain Stimulation Surgery: Targets, Indications, and Relationship to Anterior Commissure–Posterior Commissure (AC-PC) Line
Target | Indication | Anatomical Coordinates∗ |
---|---|---|
STN | Parkinson’s disease | 11-13 mm lateral to midline 4-5 mm ventral to the AC-PC plane 3-4 mm posterior to the MCP |
GPi | Dystonia | 19-21 mm lateral to the midline 2-3 mm anterior to the MCP 4-5 mm ventral to the AC-PC plane |
VIM | Essential tremor | 11-12 mm lateral to the wall of the third ventricle At the level of the AC-PC plane Between 2⁄12 and 3⁄12 of the AC-PC distance anterior to the PC |
GPi, globus pallidus interna; MCP, midcommissural point; STN, subthalamic nucleus; VIM, ventralis intermedius nucleus of the thalamus.
∗ Relationship to AC-PC line and MCP.
Direct targeting involves direct visualization of the deep nuclei using magnetic resonance imaging (MRI). Although computed tomography (CT) can be used in stereotactic targeting, it is difficult to visualize the various target nuclei or the AC and PC using CT alone. On the other hand, MRI offers multiplanar imaging capabilities with excellent anatomical delineation. The AC and PC can be identified on T1-weighted images while the STN can be identified on T2-weighted images (Fig. 53.5). The MRI studies may then be merged with stereotactically acquired CT images. The evident advantage of direct targeting is that discrete anatomical variation between individuals is taken into account because it is based on the individual’s anatomy as obtained from imaging. Generally, a combination of indirect and direct targeting methods is used. Following the targeting, localization of the target within the stereotactic space may be performed by frame-based or frameless systems.
A variety of stereotactic head frames are available. Figure 53.2 shows the Leksell stereotactic head frame (Elekta, Stockholm, Sweden) that is used in our institution, and Figure 53.6 shows a frameless system. The accuracy of the various systems has been demonstrated in previous studies, and the use of any system depends on surgeon preference, familiarity, and what is available in a particular institution. In addition to stereotactic anatomical targeting, imaging is also used to plan an entry point and trajectory to the target. The entry point and trajectory are chosen with the aim of avoiding cortical, subcortical, and periventricular blood vessels, thus reducing the risk of hemorrhagic complications. Contrast-enhanced T1-weighted MRI or contrast-enhanced CT scans help to delineate vessels along the electrode path and assist with vessel avoidance during planning.
Surgical Procedure
The stereotactic frame is placed (Fig. 53.7) and the patient is positioned supine with the stereotactic head frame fixed to the operating room table after acquisition of a stereotactic CT scan. Typically, the stereotactic CT scan is then merged with the previously acquired MRI scan and the stereotactic coordinates are obtained. The stereotactic coordinates are set on the frame and used to determine the site for skin incision and bur hole based on earlier entry point and trajectory planning. Generally in the planning, a bur hole and entry point anterior to the coronal suture are chosen. After the bur hole is performed, the dura is opened and pia coagulated for corticotomy. The microelectrodes are then inserted with the tip of the electrode placed at a defined offset above the target. At this time microelectrode recording (MER) is commenced as the electrodes are advanced in sub-millimeter steps. All sedation should be stopped before the commencement of microelectrode recording as the sedation may affect the electrophysiological mapping. MER allows for definition and verification of the physiological target. The frequency and pattern of activity of the various nuclei and white matter tracts encountered in the path to the physiological target are noted and may help determine the relationship of the trajectory to the target. These characteristic electrophysiological signatures of the targeted nuclei help confirm the physiological target. Macrostimulation is then performed to determine benefits and side effects of the stimulation.
The main components of the DBS system are an intracranial electrode and an implantable pulse generator, by an extension wire (Fig. 53.8). After the physiological target is confirmed, the electrode is implanted at the target. The two currently available electrodes are the Medtronic 3389, which spans 7.5 mm, and the 3387, which spans 10.5 mm (each model has four contacts which are 1.5 mm in height with 0.5-mm spacing between the contacts in the 3389 and 1.5-mm spacing in the 3387 model) (Fig. 53.9). The implantation can be confirmed by fluoroscopy. After the implantation, intraoperative stimulation is then performed with the DBS lead to assess for clinical improvements and side effects of stimulation.
Complications
Complications associated with DBS may be related to the surgical implantation process, related to the hardware/device, or linked to stimulation. The most serious potential complication associated with DBS electrode implantation is intracranial hemorrhage (ICH), which may result in profound neurological deficits and even death (see Fig. 53.9). ICH is reported to occur between 0.2% and 12.5% of the time.37 Asymptomatic hemorrhage is more common than symptomatic cases and is detected only incidentally on postoperative brain CT imaging. In any case, intracerebral hemorrhage is usually related to direct damage to an arterial blood vessel. Occasionally, it may be due to injury to a cortical draining vein when it may present in a delayed manner with venous infarction. Careful preoperative planning of the entry point and trajectory of approach to the target that avoids evident cortical, subcortical, or periventricular blood vessels on a contrast-enhanced brain MRI scan is a first step in avoiding hemorrhagic complications. Other strategies to prevent intracerebral hemorrhagic complications include tight intraprocedural blood pressure control (maintenance of systolic blood pressure below 130 mm Hg), prevention of entry of large amounts of intracranial air by filling the subdural space with saline and occluding the bur hole with Gelfoam and tissue glue during microelectrode recording, avoiding repeated coughing or sneezing during the procedure, and avoiding the coagulation of cortical veins. There may also be increased risk of hemorrhage based on the choice of target. For example, the GPi is reported to have higher hemorrhage rates than the STN. Binder and co-workers showed a 7% risk of hemorrhage in targeting the GPi versus a 2.2% risk in targeting the STN.64 The DBS study group reported a 9.8% risk of hemorrhage in the GPi versus 2.9% risk in the STN.65
Elderly patients may develop transient episodes of confusion and agitation following DBS, especially with procedures. Staging of bilateral DBS procedures in these elderly patients may reduce the incidence of this complication. Infection of the hardware is an ever-present risk in these procedures. Risk of infection ranges between 1% and 15% in published studies, and most commonly affects the site of the IPG.37 The risk of infection may be reduced by adhering strictly to aseptic principles during the procedure as well as the judicious use of prophylactic antibiotics. Treatment of the infection may require removal of the DBS hardware and use of appropriate antibiotics. For superficial incisional site infections in which there is no evidence of direct hardware involvement, the patient may be treated only with the exhibition of appropriate antibiotics without the need for hardware removal.