15 Basic Electromyography
Analysis of Motor Unit Action Potentials
Physiology
The basic component of the peripheral nervous system is the motor unit, defined as an individual motor neuron, its axon, and associated neuromuscular junctions (NMJs) and muscle fibers. The extracellular needle EMG recording of a motor unit is the MUAP (Figure 15–1). The number of muscle fibers per motor unit varies greatly, from 5 to 10 in laryngeal muscles to a couple of thousand in the soleus. The transverse territory of a motor unit usually ranges from 5 to 10 mm in adults, with many motor unit territories overlapping with one another. Because of this overlap, two muscle fibers from the same motor unit rarely lie adjacent to each other. Transverse motor unit territory increases greatly with age, doubling from birth to adulthood, mostly because of the increase in individual muscle fiber size.
The “size principle” governs many of the properties of motor units (Figure 15–2). The size of the motor neuron is directly related to (1) the size of the axon, (2) the thickness of the myelin sheath, (3) the conduction velocity of the axon, (4) the threshold to depolarization, and (5) the metabolic type of muscle fibers that are innervated. The larger motor neurons have larger axons, with the thickest myelin sheath (hence, the fastest conduction velocity), highest threshold to depolarization, and connections to type II, fast twitch muscle fibers. Conversely, the smaller motor neurons have smaller axons, less myelin sheath, slower conduction velocity, lower threshold to depolarization, and, in general, connections to type I, slow twitch muscle fibers. Thus, with voluntary contraction, the smallest motor units with the lower thresholds fire first. As contraction increases, progressively larger motor units begin to fire. The largest type II motor units fire with maximum contraction. During routine needle EMG, most MUAPs analyzed are thus from the smaller motor units that innervate type I muscle fibers.
Morphology
MUAP properties vary widely both within and between different muscles. Even within a muscle, there is a wide range of normal motor unit morphology, with MUAP size following a bell-shaped distribution curve (Figure 15–3). Due to this normal variability, normal values of MUAP morphology are based on the mean of many different MUAPs. The analysis of MUAP morphology can be performed on either a qualitative or a quantitative basis. To perform quantitative MUAP analysis, one must isolate 20 different MUAPs for each muscle being studied and measure their individual durations, amplitudes, and number of phases. From these values, the mean duration, amplitude, and number of phases are calculated and compared with a set of normal values for that particular muscle and age group. MUAP morphology varies depending on the muscle being studied and the patient’s age. This is particularly true of MUAP duration (Table 15–1). In general, MUAPs in proximal muscles tend to be shorter in duration than those in more distal muscles. MUAP size in adults is larger than in children, primarily because of an increase in the size of muscle fibers during development. In addition, MUAP size is generally larger in older individuals, probably as the result of dropout of motor units from the normal effects of aging, leading to some compensatory “normal” reinnervation. The loss of motor units has been estimated to be approximately 1% per year, beginning in the third decade of life, which then increases rapidly after age 60.
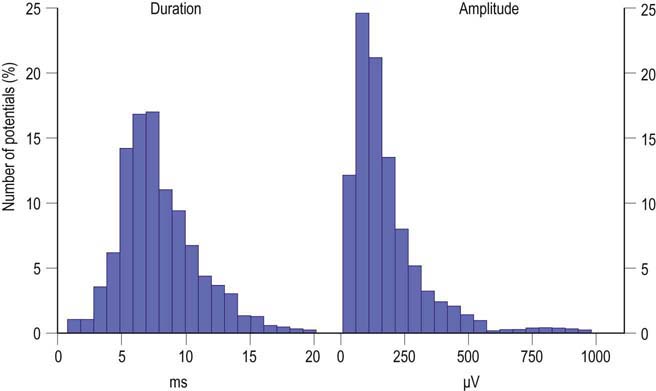
FIGURE 15–3 Range of normal motor unit action potential (MUAP) duration and amplitude.
(Reprinted with permission from Buchthal, F., Guld, C., Rosenfalck, P., 1954. Action potential parameters in normal human muscle and their dependence on physical variables. Acta Physiol Scand 32, 200.)
Duration
MUAP duration is the parameter that best reflects the number of muscle fibers within a motor unit (Figure 15–4). Typical MUAP duration is between 5 and 15 ms. Duration is defined as the time from the initial deflection from baseline to the final return of the MUAP to baseline. It depends primarily on the number of muscle fibers within the motor unit and the dispersion of their depolarizations over time. Dispersion in turn depends on the longitudinal and transverse scatter of endplates and on variations in terminal distances and conduction velocities. Duration lengthens as the number of fibers and the territory of a motor unit increase; it varies directly with age (increased age, increased duration) and inversely with temperature (decreased temperature, increased duration) and depends on the individual muscle being studied. Proximal and bulbofacial muscles in general have MUAPs of shorter duration. When performing EMG, it often is more rewarding to listen to the potential than to see it. This is especially true when evaluating MUAP duration, because duration correlates with pitch. Long-duration MUAPs (low frequencies) sound dull and thuddy, whereas short-duration MUAPs (higher frequencies) sound crisp and static-like. As the electromyographer gains experience, the sound of a long-duration versus a short-duration MUAP becomes unmistakable.
Polyphasia, Serrations, and Satellite Potentials
Polyphasia is a measure of synchrony, that is, the extent to which the muscle fibers within a motor unit fire more or less at the same time. This is a nonspecific measure and may be abnormal in both myopathic and neuropathic disorders. The number of phases can be easily calculated by counting the number of baseline crossings of the MUAP and adding one (Figure 15–4). Normally, MUAPs have two to four phases. However, increased polyphasia may be seen in up to 5 to 10% of the MUAPs in any muscle and is considered normal. The one exception is the deltoid, where up to 25% polyphasia may be normal. Increased polyphasia beyond 10% in most muscles and 25% in the deltoid is always abnormal. Through the speaker, polyphasic MUAPs are recognized as a high-frequency “clicking” sound.
Satellite potentials (also known as linked potentials or parasite potentials) are interesting phenomena seen in early reinnervation. After denervation, muscle fibers often are reinnervated by collateral sprouts from adjacent intact motor units. The newly formed sprout often is small, unmyelinated or thinly myelinated, and therefore very slowly conducting. Because of the slow conduction time and increased distance, reinnervated muscle fibers are seen as time-locked potentials that trail the main MUAP (Figures 15–5 and 15–6). These satellite potentials are extremely unstable (see section on Stability) and may vary slightly in their firing rate or may block and not fire at all (Figure 15–7). Over time, the sprout matures, and the thickness of the myelin and consequently the conduction velocity increase. The satellite potential then fires more closely to the main potential and ultimately will become an additional phase or serration within the main complex. It is usually necessary to put the main MUAP on a delay line to appreciate a satellite potential and to demonstrate that it is time locked to the main potential.
Amplitude
MUAP amplitude varies widely among normal subjects. Most MUAPs have an amplitude greater than 100 µV and less than 2 mV. Amplitude is generally measured from peak to peak of the MUAP (Figure 15–4). Amplitude is essentially a high-frequency response. Tissue between the needle and muscle fibers effectively acts as a high-frequency filter. Thus, unlike duration, most muscle fibers of a motor unit contribute little to the amplitude. MUAP amplitude reflects only those few fibers nearest to the needle (only 2–12 fibers). Hence, amplitude is not as helpful as duration in judging motor unit size. Several factors are associated with increased amplitude, including (1) the proximity of the needle to the motor unit (Figure 15–8), (2) increased number of muscle fibers in a motor unit, (3) increased diameter of muscle fibers (i.e., muscle fiber hypertrophy), and (4) more synchronized firing of the muscle fibers. Listening to the EMG, the amplitude of MUAPs is correlated not with pitch but with volume.