Chapter 40 Atrioventricular Block
In 1852, Stannius noted that placing a ligature between the atria and the ventricles could cause bradycardia in a frog’s heart.1 In the late 1800s, Tawara and His identified the atrioventricular (AV) node and His bundle as the normal conduction axis between the atria and the ventricles in humans, and Wenckebach suggested blocked AV conduction as a cause for slow and irregular pulses.2–4
Epidemiology of Atrioventricular Block
Transient AV block can be observed in children and young adults during sleep5; persistent AV block is unusual. This type of AV block is usually caused by increased vagal tone and is often a normal finding. In a continuous monitoring study of 100 healthy teenaged boys, transient first-degree AV block was observed in 12% and second-degree AV block in 11%.5 In young adults the incidence of transient AV block decreases to about 4% in women and 6% in men.6,7 In the normal older adult population, transient type I (Wenckebach) second-degree AV block is seen only rarely (1%), and higher-grade AV block is not observed.8
Persistent first-degree AV block is rarely seen in young adults. Review of more than 70,000 electrocardiograms (ECGs) from young men entering the Canadian and U.S. military demonstrated a prevalence of first-degree AV block of less than 1%.9,10 Electrocardiographic studies have shown increased P-R intervals and an increased incidence of first-degree AV block with aging.9–14 While approximately 2% of adults older than 20 years of age have first-degree AV block, the incidence increases to more than 5% in people older than 50 years of age.11,13 With increasing age, the development of AV conduction disorders is more common; in one epidemiologic study of 1500 patients older than 65 years of age, AV conduction and intraventricular conduction defects were identified in 30% of patients.14 Using high-resolution electrocardiographic techniques, the increased P-R interval associated with aging was found to be caused by delay in conduction in the AV node or the proximal portion of the His bundle.11 In people younger than 60 years of age, it is uncommon (4%) for persistent first-degree AV block to progress to second-degree or higher-grade AV block in the absence of associated disease.13
Acquired persistent second-degree and third-degree AV blocks are almost never observed in normal populations regardless of age. The incidence of symptomatic high-grade AV block is currently estimated to be 200 per million per year.15
Isolated congenital complete (third-degree) AV block is a well-described problem that occurs in approximately 1 in every 20,000 live births.16 Congenital complete AV block is the most common manifestation of neonatal lupus erythematosus and appears to be associated with the development of autoantibodies in the maternal circulation. Other hereditary conditions associated with AV block are the Kearns-Sayre syndrome (ophthalmoplegia, retinitis pigmentosa) and myotonic dystrophy.17
Anatomy
Anatomy and Blood Supply
The AV system of specialized myocytes is the only muscular bridge through the insulating fibro-fatty tissue plane that separates the atrial myocardial masses from the ventricular myocardial masses. The AV system begins with the atrioventricular node at its atrial end. The compact node is recognizable, when seen in cross-sections, as a half-oval shaped structure adjacent to the central fibrous body in the young (Figure 40-1, A).18 With increasing age, the shape changes to become spindle-like.19 When traced inferiorly, toward the base of Koch’s triangle, the compact area diverges into two prongs, usually with the artery supplying the node running in between. The extent of the prongs varies from heart to heart; the right extension increases with age.20 Interposing between the compact node and the working atrial myocardium is a zone of transitional cells. These cells are histologically distinct from the cells of the compact node as well as the working cells but are not insulated from the surrounding myocardium. They approach the compact node superiorly, inferiorly, and from the left and right sides of the atrial septum. In the vestibular area of the right atrium, an overlay of ordinary myocytes is present in the subendocardium from the atrial wall in front of the oval fossa that streams over the zone of transitional cells. The transitional cells, therefore, provide the crucial proximal bridge between the ordinary and the specialized myocardium. Fibro-fatty tissue increases with age in this region, and the transitional cell zone becomes wider.19 When the conduction system is traced into the penetrating bundle of His, little difference is seen in the cellular composition of the two areas (see Figure 40-1, B). The specialized myocardial cells themselves, however, become aligned in a more parallel fashion distally into the AV conduction bundle. The AV node, therefore, is an integral part of the atrial musculature (see Figure 40-1, A), in contrast to the atrioventricular bundle, which, in passing through the central fibrous body, is insulated from the adjacent myocardium (see Figure 40-1, B). The His bundle, approximately 2 mm in cross-sectional diameter, is the only bridge of myocardial continuity between the atria and the ventricles. The common AV bundle and its continuation, the branching bundle, and bundle branches are also ensheathed in fibrous tissue. In the normal heart, the AV-branching bundle is sandwiched between the membranous septum and the muscular ventricular septum, usually a little to the left of the septal crest, giving origin to the left and right bundle branches (see Figures 40-1, C to E). The AV bundle lies in the subendocardium of the left ventricle beneath the commissure between the right-coronary and noncoronary leaflets of the aortic valve. In most hearts, the right bundle branch passes through the septal crest to emerge subendocardially in the septal aspect of the right ventricle (see Figure 40-1, E). Distally, the bundle branches divide into increasingly finer branches. Eventually, they ramify into the so-called Purkinje network and lose the fibrous sheaths at the interface with the ordinary ventricular myocardium.18
Most frequently, the arterial supply to the AV node is a branch from the right coronary artery, whereas it is a branch from the circumflex artery in nearly 20%. When the dominant right coronary artery extends beyond the cardiac crux, the nodal artery originates distal to the U loop of the dominant artery.20,21 In most hearts, the penetrating bundle and AV conduction bundle are supplied by a branch from the posterior descending coronary artery. The right bundle branch is supplied by the first septal perforating artery arising from the anterior descending coronary artery, which also supplies the AV conduction bundle in some hearts.22,23
Congenital Complete Atrioventricular Block
Congenital complete heart block can occur in congenitally malformed hearts or in otherwise normal hearts.24 Complete AV block associated with a cardiac defect is most frequently seen in the anomaly of congenitally corrected transposition, in isomeric arrangement of the atrial appendages, and in some AV septal defects. Although complete AV block may be present at birth, it is more usual for the arrhythmia to be progressive.
When occurring in an otherwise structurally normal heart, the pattern of the cardiac conduction system can take one of three anatomic forms according to the location of the interruption: (1) atrial-axis discontinuity, (2) nodal-ventricular discontinuity, or (3) intraventricular discontinuity.25–27 The last form is extremely rare.28 The AV node is deficient or even absent in the most common form, which is described as atrial-axis discontinuity.25,29 The block is proximal to the His bundle. In the extreme form, the area that is normally occupied by the compact AV node and the zone of transitional cells is replaced by fibrous tissue or fibro-fatty tissue (Figure 40-2, A). In others, variable remnants of the nodal tissues appear as islands in the fibrous or fibro-fatty tissues.
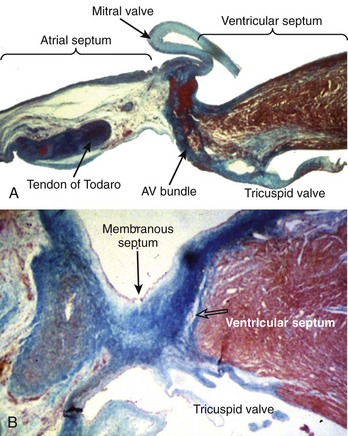
Figure 40-2 Histologic sections from two infants with congenital complete heart block cut in similar planes as those shown in Figure 40-1. A, An extreme case of atrial-axis discontinuity. The atrial septum totally lacks the myocardium in the region of the atrioventricular node and the node is missing. B, An example of intraventricular discontinuity. Only a small island of the branching bundle buried in the fibrous tissue remains (open arrow) (trichrome stain: myocardium in red; fibrous tissue in green-blue).
The rarest form, intraventricular discontinuity of the conduction bundle, may occur in families.28 The branching bundle or the proximal parts of the bundle branches are replaced by fat or fibrous tissue (see Figure 40-2, B).
The association of congenital complete heart block with maternal connective tissue disease is well documented.30–32 The presence of anti-Ro(SS-A) antigen in maternal serum is a marker for isolated congenital heart block.32 A study of hearts from 8 afflicted children has shown the lack of an AV node in 7, whose maternal sera were anti-Ro positive, and nodal-ventricular discontinuity in the remaining 1, whose maternal serum was anti-Ro negative.33 However, Chow et al described two cases with a combination of nodal-ventricular and intraventricular discontinuities demonstrating that morphologic patterns are not always clear cut.34 Furthermore, anti-Ro antibodies were not exclusively associated with atrial-axis discontinuity, since maternal serum was anti-Ro positive in one of these two cases.
Another study compared the anatomic substrate producing complete heart block in normally structured hearts and in hearts with isomerism of the atrial appendages.35 The pattern of AV block in the cases with left isomerism was nodal-ventricular discontinuity, whereas all but one of the normally structured hearts showed atrial-axis discontinuity with the anticipated nodal area filled with fibro-fatty tissue. The remaining normally structured heart showed the rarest substrate—intraventricular discontinuity. Interestingly, associated fibrosis of several of the sinus nodes was present, suggesting some sort of target mechanism or deficiency specific to the conduction tissues.
The association of complete AV block with congenitally corrected transposition of the great arteries is well recognized.36 The block may be congenital because of deficiency of the AV node or bundle. More commonly, AV block is progressive in adults. When congenitally corrected transposition occurs with usual arrangement of the atria, the AV conduction system is abnormally located. The AV node is displaced anteriorly, and the AV conduction bundle courses anterosuperiorly, in the trigonal region of fibrous continuity between the pulmonary and mitral valves, related to the anterocephalad border of the left ventricular outflow tract before entering the ventricular septum. The long bundle renders it particularly vulnerable to fibrotic changes with increasing age, eventually resulting in interruption.
Acquired Complete Atrioventricular Block
Complete AV block can be categorized into four major pathologic groups and a further group with miscellaneous conditions.37 An autopsy series of hearts from 200 patients who had AV block of more than 1-month duration found idiopathic bilateral bundle branch fibrosis in 38%, coronary artery disease in 17.5%, cardiomyopathy in 13%, calcific AV block in 11%, and other causes in 20.5%.37
Idiopathic Bilateral Bundle Branch Fibrosis
The crest of the muscular ventricular septum undergoes degenerative changes, including fibrosis, fatty infiltration, and focal microscopic dystrophic calcification with advancing age. The normal aging process begins in the fourth decade of life but may be accelerated by coronary artery disease, diabetes mellitus, and hypertensive heart disease. Since the branching AV bundle and the beginning of the main bundle branches are located in this area, they are susceptible to being disrupted (Figure 40-3). Two forms of bundle branch fibrosis, focal and diffuse—dubbed Lev disease and Lenègres disease, respectively—are recognized, but they probably represent extreme ends of a pathologic spectrum.38,39 In both groups the mean ages are reported to be in the sixth and seventh decades. Damage to the distal branches is minimal in the focal form, whereas damage in the diffuse form can extend to the middle and distal portions of both left and right bundle branches.
Coronary Artery Disease
Complete AV block can occur in acute or chronic coronary artery disease. Since different segments of the proximal portion of the AV conduction system are supplied by the right or the left coronary systems, the site of infarction related to coronary occlusion is important. When AV block complicates acute posteroseptal myocardial infarction, the occluded coronary artery is always the artery that ultimately gives origin to the AV nodal artery. Most cases reveal only small focal areas of necrosis in the node, the AV bundle, or both. Massive necrosis of the AV node and its approaches, inflammatory cell infiltration, or occlusion of the nodal artery is rare. In some cases, no obvious pathologic changes are seen in conduction tissues. By contrast, anteroseptal infarction associated with occlusion of the anterior descending coronary artery is more extensive and causes necrotic damage to the branching and bundle branches. The AV node may or may not be spared, although careful studies have shown no arterial lesions in the supply to the AV node.40
Chronic coronary insufficiency, with or without previous myocardial infarction, can affect various parts of the AV conduction system. The damage predominantly to the bundle branches may, over time, progress to cause complete AV block. The most common pattern in autopsy series is destruction of both bundle branches.39,41 Pathologic studies suggest that the majority of patients with chronic AV block do not have more coronary atherosclerosis than do normal patients without AV block.41,42 Some cases may be associated with small vessel disease.
Cardiomyopathy
Hypertrophic cardiomyopathy is rarely associated with chronic AV block. Histologic investigations have shown interstitial fibrosis or necrosis in the conduction system, interruption of the His bundle, abnormally small intramural coronary arteries with thick walls, and narrow lumens.43–45
Miscellaneous
This heterogeneous group includes amyloidosis, hemochromatosis, connective tissue disease, myocarditis familial cardiomyopathy and skeletal myopathy, infective endocarditis, and tumors involving the conduction system. In amyloidosis, heavy deposits are found in the sinus node with relatively small amounts in the AV node and main conduction bundles. In patients with AV block, deposits are present in the ventricular myocardium, which also shows extensive scarring. Involvement of the small arteries suggest fibrosis and attenuation of the conduction bundles are more related to the scarring process.41 Myocarditis of any etiology, whether acute or chronic, may cause complete AV block. In the acute phase, inflammatory changes are predominantly in the ventricular myocardium affecting the distal bundle branches. Rarely, the conduction system is more involved than is the surrounding myocardium.41 When the conduction system is involved, the changes include degeneration, vacuolization, and necrosis of cells of the bundle branches. Infective endocarditis affecting the aortic valve, the mitral valve, or both may extend to the central fibrous body, membranous septum, and crest of the muscular ventricular septum to involve the AV bundles. Any type of tumor that metastasizes to the heart has the potential to affect the conduction system to varying degrees. Metastatic tumor deposits in the ventricular septum can destroy the bundle branches. One particular benign tumor, a mesothelioma, has an affinity for the AV node and its approaches. It is a slow-growing cystic mass located in this region of the atrial septum, hence also known as cystic tumor of the AV node. A female-to-male ratio of approximately 3 : 1 and a mean age of 38 years at presentation are seen. Some patients may die suddenly without any history of heart problems.46
Surgical and Interventional
Complete AV block may be a consequence of interventional procedures to correct congenital heart malformation. Damage may be caused by sutures or incisions into the musculature containing the conduction bundles, AV node, or their arterial supply. Although surgically induced iatrogenic block is rare nowadays, the shift toward trans-catheter device closure for ventricular septal defects has again put the spotlight on the potential risk to the AV conduction system.47,48 AV block may also be a complication following isolated myectomy or alcohol ablation for hypertrophic obstructive cardiomyopathy.49,50
Basic Electrophysiology
It is generally accepted that the AV junction consists of the AV node with its atrial connections of transitional fibers and the bundle of His.51 In electrophysiological considerations of AV block, it is useful to define the AV junction more broadly, including the atrial connections to the AV node, the AV node, the bundle of His, and the proximal portions of the bundle branches that are insulated from the ventricular myocardium by fibrous matrix. Heart block can occur from interruption of conduction in any of these components of the AV junction as defined broadly. In this array of components, great electrophysiological and histologic diversity is seen (Figure 40-4). The range of conduction velocities, resting and action potential (AP) amplitudes, AP durations, and intercellular conductivity observed within the AV junction is greater than in the remainder of the atrial and ventricular myocardium.
The Compact Atrioventricular Node
Within the central compact node are cells with distinct electrophysiological characteristics. These characteristics of the prototypical AV nodal cells manifested by intracellular recordings in multicellular preparations have been replicated in cells isolated from the AV node, allowing determination of the activities of specific currents, pumps, and exchangers.52,53 The amplitudes of resting potentials (–60 mV), APs (–85 mV), and upstroke velocities (<10 V/sec) are reduced. These cells manifest diastolic depolarization, a high total transmembrane (input) resistance, and relative insensitivity to changes in extracellular K+. They have post-repolarization refractoriness; the absolute refractory period outlasts the AP. The relative refractory period, during which relatively diminished, more slowly conducting APs are generated, is prolonged well into diastole. The amplitudes and upstroke velocities of APs and conduction velocity are strongly modulated by autonomic activity, increasing with adrenergic (sympathetic) stimulation and decreasing with cholinergic (vagal) stimulation. The AV junction is richly innervated with vagal and sympathetic fibers, but recent observations with high-resolution staining for neural tissues indicate that innervation of the compact node may be more sparse than that of the transitional fibers or the nodal extensions.53,54
AV nodal cells, like sinoatrial nodal cells, lack sodium (Na+) channels, which provide the excitatory current for atrial and ventricular cells; some cells may have channels that are inactivated at the low resting potentials.55,56 The excitatory current for AV nodal cells is ICaL, a calcium current that generates a slow upstroke because it is slower and less intense than the Na+ current. L-type calcium ion (Ca2+