Antigens and Antibodies
At the conclusion of this chapter, the reader should be able to:
• Define the terms antigen and antibody.
• Compare the characteristics of major histocompatibility complex (MHC) classes I and II.
• Name and compare the characteristics of each of the five immunoglobulin classes.
• Draw and describe a typical immunoglobulin G (IgG) molecular structure.
• Name the four phases of an antibody response.
• Describe the characteristics of a primary and secondary (anamnestic) response.
• Compare the terms antibody avidity and antibody affinity.
• Describe the method of production of a monoclonal antibody.
• Analyze a case study related to antigens or antibodies.
• Correctly answer case study related multiple choice questions.
• Be prepared to participate in a discussion of critical thinking questions.
• Describe the principle and agglutination reactions in ABO blood grouping.
• Describe the principle, expected results, reference values, and clinical interpretation of the serum protein electrophoresis procedure.
Antigen Characteristics
General Characteristics of Immunogens and Antigens
Histocompatibility Antigens
Nucleated cells such as leukocytes and tissues possess many cell surface–protein antigens that readily provoke an immune response if transferred into a genetically different (allogenic) individual of the same species. Some of these antigens, which constitute the major histocompatibility complex (MHC) (see Color Plate 2), are more potent than others in provoking an immune response. The MHC is referred to as the human leukocyte antigen (HLA) system in humans because its gene products were originally identified on white blood cells (WBCs, leukocytes). These antigens are second only to the ABO antigens in influencing the survival or graft rejection of transplanted organs. HLAs are the subject of numerous scientific investigations because of the strong association between individual HLAs and immunologic disorders (see Chapter 31 for more discussion of the MHC).
Major Histocompatibility Complex Regions
The MHC is divided into four major regions (Fig. 2-1)—D, B, C, and A. The A, B, and C regions are the classic or class Ia genes that code for class I molecules. The D region codes for class II molecules. Class I includes HLA-A, HLA-B, and HLA-C. The three principal loci (A, B, and C) and their respective antigens are numbered, for example, as 1, 2, 3. The class II gene region antigens are encoded in the HLA-D region and can be subdivided into three families, HLA-DR, HLA-DC (DQ), and HLA-SB (DP).
Classes of HLA Molecules
Structurally, there are two classes of HLA molecules, class I and class II (Table 2-1). Both class I and class II antigens function as targets of T lymphocytes (see Chapter 4 for a further discussion of lymphocytes) that regulate the immune response (Fig. 2-2). Class I molecules regulate interaction between cytolytic T cells and target cells and class II molecules restrict the activity of regulatory T cells. Thus, class II molecules regulate the interaction between helper T cells and antigen-presenting cells (APCs). Cytotoxic T cells directed against class I antigens are inhibited by CD8 cells; cytotoxic T cells directed against class II antigens are inhibited by CD4 cells. Many genes in the class I and class II gene families have no known function.
Table 2-1
Comparison of MHC Class I and Class II
Class I | Class II | |
Loci | HLA-A, -B, and -C | HLA-DN, -DO, -DP, -DQ, and -DR |
Distribution | Most nucleated cells | B lymphocytes, macrophages, other antigen-presenting cells, activated T lymphocytes |
Function | To present endogenous antigen to cytotoxic T lymphocytes | To present endogenous antigen to helper T lymphocytes |
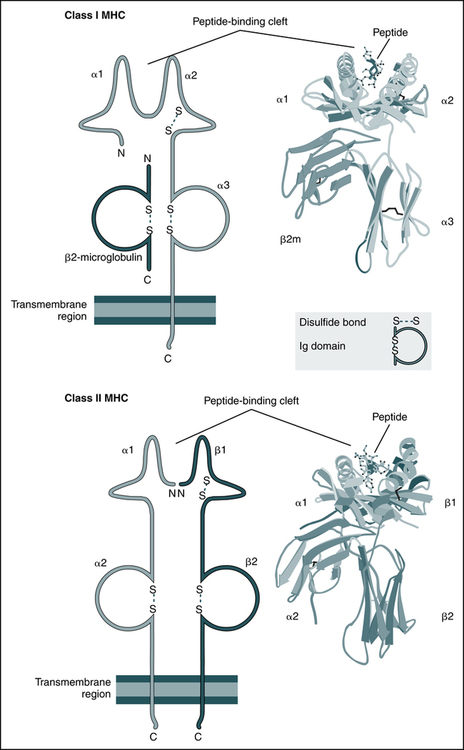
The schematic diagrams (left) and models (right) of the crystal structures of class I and class II MHC molecules illustrate the domains of the molecules and the fundamental similarities between them. Both types of MHC molecules contain peptide-binding clefts and invariant portions that bind CD8 (the α3 domain of class I) or CD4 (the β2 domain of class II). β2m, β2-Microglobulin. (From Abbas AK, Lichtman AH: Basic immunology: functions and disorders of the immune system, updated edition, ed 3, Philadelphia, 2011, Saunders; crystal structures courtesy Dr. P. Bjorkman, California Institute of Technology, Pasadena, Calif.)
Autoantigens
The evolution of a recognition system that can recognize and destroy nonself material must also have safeguards to prevent damage to self antigens. The body’s immune system usually exercises tolerance to self antigens but, in some situations, antibodies may be produced in response to normal self antigens. This failure to recognize self antigens can result in autoantibodies directed at hormones, such as thyroglobulin (see Chapter 28).
Blood Group Antigens
Blood group substances are widely distributed throughout the tissues, blood cells, and body fluids. When foreign RBC antigens are introduced to a host, a transfusion reaction or hemolytic disease of the fetus and newborn can result (see Chapter 26). In addition, certain antigens, especially those of the Rh system, are integral structural components of the erythrocyte (RBC) membrane. If these antigens are missing, the erythrocyte membrane is defective and results in hemolytic anemia. When antigens do not form part of the essential membrane structure (e.g., A, B, and H antigens), the absence of antigen has no effect on membrane integrity.
General Characteristics of Antibodies
Antibodies are specific proteins referred to as immunoglobulins. Many antibodies can be isolated in the gamma globulin fraction of protein by electrophoresis separation (Fig. 2-3). The term immunoglobulin (Ig) has replaced gamma globulin because not all antibodies have gamma electrophoretic mobility. Antibodies can be found in blood plasma and in many body fluids (e.g., tears, saliva, colostrum).
Immunoglobulin (Ig) Classes
Five distinct classes of immunoglobulin molecules are recognized in most higher mammals—IgM, IgG, IgA, IgD, and IgE. These Ig classes differ from each other in characteristics such as MW and sedimentation coefficients (Table 2-2).
Table 2-2
Characteristics of Immunoglobulin Classes
IgM | IgG | IgA | IgE | IgD | |
Molecular weight (daltons, Da) | 900,000 | 160,000 | 360,000 | 200,000 | 160,000 |
Sedimentation coefficient (Σ) | 19 | 7 | 11 | 8 | 7 |
Carbohydrate (%) | 12 | 8 | 7 | 12 | 12 |
Subclasses | — | IgG1-4 | α1, α2 | — | — |
Serum concentration, adults (mg/mL) | 1.5 | 13.5 | 3.5 | 0.05 | Trace |
Serum half-life (days)∗ | 5 | 23 | 6 | 2.5 | 3 |
∗Half life (days) = the amount of time to reach ½ activity concentration. Serum values are average concentrations in normal, healthy individuals.
Adapted from Peakman M, Vergani D: Basic and clinical immunology, St Louis, 2009, Elsevier, p 41.
Immunoglobulin M
• Infectious diseases, such as subacute bacterial endocarditis, infectious mononucleosis, leprosy, trypanosomiasis, malaria, and actinomycosis
• Collagen disorders, such as scleroderma
• Hematologic disorders, such as polyclonal gammopathies, monocytic leukemia, and monoclonal gammopathies (e.g., Waldenström’s macroglobulinemia)
Immunoglobulin G
Normal human adult serum values of IgG are 800 to 1800 mg/dL (90 to 210 IU/mL). In infants 3 to 4 months old, the IgG level is approximately 350 to 400 mg/dL (40 to 45 IU/mL), gradually increasing to 700 to 800 mg/dL (80 to 90 IU/mL) by the end of the first year of life (Fig. 2-4). The average adult level is achieved before age 16 years. Other body fluids containing IgG include cord blood (800 to 1800 mg/dL) and CSF (2 to 4 mg/dL).
Immunoglobulin A
• Infectious diseases, such as tuberculosis and actinomycosis
• Collagen disorders, such as rheumatoid arthritis
• Hematologic disorders, such as polyclonal gammopathies, monocytic leukemia, and monoclonal gammopathy (e.g., IgA myeloma)
• Liver disease, such as Laennec’s cirrhosis and chronic active hepatitis
Antibody Structure
Typical Immunoglobulin Molecule
The basic unit of an antibody structure is the homology unit, or domain. A typical molecule has 12 domains, arranged in two heavy (H) and two light (L) chains, linked through cysteine residues by disulfide bonds so that the domains lie in pairs (Fig. 2-5). The antigen-binding portion of the molecule (N-terminal end) shows such heterogeneity that it is known as the variable (V) region; the remainder is composed of relatively constant amino acid sequences, the constant (C) region. Short segments of about 10 amino acid residues within the variable regions of antibodies (or T cell receptor [TCR] proteins) form loop structures called complementary-determining regions (CDRs). Three hypervariable loops, also called CDRs, are present in each antibody H chain and L chain. Most of the variability among different antibodies or TCRs is located within these loops.
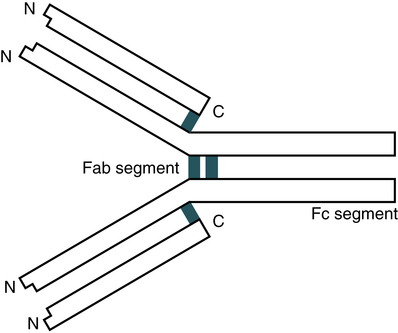