Chapter 80 Antiarrhythmic Drugs
Reorientation of drug therapy has also been necessary in the wake of the knowledge that certain antiarrhythmic agents, while suppressing supraventricular and ventricular arrhythmias, may increase mortality by their associated proarrhythmic effects. This was demonstrated in the Cardiac Arrhythmia Suppression Trial.1 The findings have important clinical implications with respect to the choice of antiarrhythmic agents in the short-term, long-term, and prophylactic control of arrhythmias. The issue is critical in the choice of intravenous therapy of ventricular arrhythmias not only in hospitalized patients but also in those developing out-of-hospital VT or VF, especially in the choice of agents as an integral part of the current Advanced Cardiovascular Life Support (ACLS) guidelines.2
Classification of Antiarrhythmic Drugs Revisited
Interest in the mechanisms of action of pharmacologic agents has provided the basis for their classification since the 1970s.3–9 The original intent was to classify the mechanisms of action of antiarrhythmic drugs and not the drugs themselves. Such an approach permitted grouping of drugs by their dominant action with the inevitable realization that many antiarrhythmic drugs in the clinic exerted single actions (e.g., β-blockers) or a varying spectrum of actions (sotalol and amiodarone) with their defined overall clinical effects both beneficial as well as deleterious.
The attempts at classification of the manner in which antiarrhythmic agents might work beneficially in the control of disorders of rhythm; this follows closely in the wake of a series of experimental studies of a number of structurally disparate compounds having certain discrete electrophysiological actions.3–5,8,9 These compounds were local anesthetics or sodium channel blockers, β-adrenoceptor blockers, drugs that were also found to have anti-fibrillatory actions in the context of their property to selectively prolong cardiac repolarization, and those that were found to selectively block calcium channels at the membrane level. The premise of the initial classification was that each of the mechanisms described exerted, although in differing ways, an anti-fibrillatory effect in a standardized experimental model of VF.5 The evolution of the steps in the classification of antiarrhythmic mechanisms of drugs that are now widely used stems from the original framework, which has subsequently been modified slightly, the most recent being by Nattel and Singh.3–5,8,10 The final step completed in 1979 is shown in Figure 80-1.11 The need for the classification of antiarrhythmic drug mechanisms was conceived almost simultaneously and independently on both sides of the Atlantic. It will be noted that the Hoffman and Bigger attempt at classifying antiarrhythmic drugs placed compounds such as quinidine and procainamide, which had been known to slow conduction as well as to prolong repolarization in one category (type 1), and lidocaine and diphenylhydantoin (DPH), which also inhibited conduction but shortened repolarization into another category (type 2).12 Because propranolol in high doses exhibited potent conduction blocking properties, it was included among type 1 antiarrhythmic compounds.
On the basis of the findings in the experimental laboratory, it was suggested that type 1 compounds terminated or prevented arrhythmias by converting uni-directional block into a bi-directional one in an arrhythmia circuit, whereas the type 2 agents acted by eliminating uni-directional block by increasing conduction velocity. Subsequently, as an integral part of a series of studies on which the conventional antiarrhythmic classification has been based, it was found that this observation might have occurred as a consequence of the use of potassium ion (K+) concentration of 2.7 mEq/L in the perfusion media.9 The retesting of the effects of lidocaine and DPH in media containing physiological levels of potassium ions clearly established that both the compounds had their predominant actions as inhibitors of sodium (Na) channel–mediated conduction. It also became evident that in clinically significant concentrations, propranolol exerted no local anesthetic actions.8
In contrast, in the comprehensive classification scheme suggested by Singh, and Singh and Vaughan Williams, all drugs that exerted local anesthetic actions in the nerve as well as in the myocardial membrane (including lidocaine, quinidine, procainamide, disopyramide, and DPH among numerous others) were thought to act via class I actions; and propranolol and other β-adrenoceptor–blocking compounds that exhibited sympatholytic actions were characterized as acting via class II antiarrhythmic actions.3,5,7
When it was found that compounds such as sotalol (a β-blocker) and amiodarone, which prolonged repolarization, exerted unequivocal anti-fibrillatory actions not accounted for by class I or class II actions, the possibility of a class III action was suggested.3 Subsequently, on the basis of the electropharmacologic effects of the drug verapamil, a fourth class of antiarrhythmic actions was added by Singh and Vaughan Williams in 1972.7 Hauswirth and Singh subdivided class I compounds into those that suppressed sodium channels in all cardiac fast-channel tissues, as typified by quinidine and procainamide (class IA agents), and those that inhibited sodium channels only in diseased or depolarized tissues (class IB: lidocaine and DPH).11 Another difference between these two subclasses was that in the case of the 1A agents, repolarization was also prolonged, whereas in the case of 1B agents, repolarization was abbreviated. Harrison completed the subclassification by assigning the potent class I agents flecainide and encainide into a group designated as class IC.13 Experimental studies by Campbell validated the separation of subclasses of sodium channel blockers on the biophysical basis; he found that class IA agents had sodium channel–blocking kinetics between those of class IB agents (very fast) and IC compounds (very slow).14
This classification of antiarrhythmic drugs is still widely used in clinical practice. It has been the basis for the development of newer antiarrhythmic compounds. It was recognized early that a direct extrapolation of the experimental data to the clinical setting in this regard might not be readily possible. However, it was considered that for advances in understanding drug action, it was desirable to explore the effects of various classes of antiarrhythmic drugs in myocardial cells and membranes in terms of ion channels and related parameters, on the one hand, and relate them to their clinical effects, on the other hand.8,11 This approach was first suggested in detail by Hauswirth and Singh in 1979, and it re-emerged subsequently in the form of the Sicilian Gambit in 1991, incorporating an increasing understanding of membrane currents with the introduction of the patch clamp technique.11,15
Amiodarone and sotalol—two unique compounds—the dominant electrophysiological property of which is the prolongation of repolarization, formed the basis for the class III action.3,4,7,16–18 Amiodarone and sotalol have provided the background for the synthesis and characterization of simpler compounds (such as dofetilide and azimilide) as well as the impetus to develop other agents with similar properties but safer electropharmacologic profiles. The main properties of the major agents are discussed in this chapter relative to their roles in the control of supraventricular and ventricular tachyarrhythmias. Recently approved compounds for AF treatment, such as dronedarone and vernakalant, are also discussed. Certain electrophysiological properties of antiarrhythmic compounds are of much importance in the clinical area. They will be emphasized at the outset.
Heart Rate Dependency of the Action of Antiarrhythmic Agents
The electrophysiological property that also appears to be of major clinical interest is the rate-dependent effect of the compounds on the action potential duration (APD) and refractoriness.19 Rate dependency refers to a different effect at varying heart rates, with reverse-rate dependence (less effect at higher rates) being of great clinical importance. The differences in this parameter among the various agents may be of therapeutic relevance. This issue is of particular relevance in the case of their role in the control of AF. The drugs that exert the classic reverse-rate dependency (dofetilide, quinidine, and d,l-sotalol) appear to exhibit a similar ceiling of efficacy for maintaining the stability of sinus rhythm and a similar propensity for inducing torsades de pointes (TdP). Their effect on prolonging the APD and the effective refractory period (ERP) in the atrial muscle decline as the stimulation frequency increases. All such compounds are powerful blockers of the delayed rectifier current (IKr), and they are likely to be more effective in terminating AFL than AF when acutely administered. They are moderately effective in preventing recurrences of AF and AFL in the paroxysmal and persistent forms of these arrhythmias.
In contrast, compounds such as azimilide and possibly ambasilide, which may also inhibit the slow component of the delayed rectifier current (IKs), exhibit a different pattern of rate dependency of action with respect to the APD and associated refractoriness. Under the action of the drugs, a parallel increase occurs in the APD and the ERP, as shown in Figure 80-2. Whether this is clinically significant may require a direct comparison of these compounds with those of other so-called class III compounds such as dofetilide. In the case of azimilide, the available data suggest a low incidence of TdP, a neutral effect on mortality in high-risk patients with previous myocardial infarction (MI) and at least moderate efficacy in maintaining the stability of sinus rhythm in patients with AF.20,21 Large pivotal placebo-controlled trials with respect to azimilide are in progress.
Of particular interest, the action of amiodarone and that of dronedarone, with respect to rate-dependent effects, are similar, as they are associated with a parallel shift (i.e., ERP increases in a parallel fashion over a wide range of frequencies), as noted in the case of azimilide and ambasilide.10,22 In the case of amiodarone evidence for the effectiveness of the drug for maintaining the stability of sinus rhythm is growing. Its potency (see later) appears the highest among all class III antiarrhythmic compounds, and it is associated with the lowest incidence of TdP in the context of the longest Q-T interval that amiodarone may produce during the course of long-term treatment. The precise reason for this combination of drug effects remains unclear, but the observations are clearly of theoretical importance for the purposes of developing compounds in the future. Perhaps of great importance also is the observation that in the case of flecainide (presumably also propafenone) is the nature of their effects on the ERP and the APD as a function in atrial tissue. Wang and coworkers found that in a variety of mammalian atria, flecainide (and presumably propafenone acts in a similar manner) had the property of prolonging the APD and the ERP as a function of rate.23 For example, greater effects were seen as the stimulation rates were increased (Figure 80-3) (see the discussion on atrial-selective drugs below). The available data suggest that the rate-related effects of antiarrhythmic drugs may vary in differing cardiac tissues, which is possibly a reflection of the differential action of drugs on the ion channels that they may inhibit to varying extents.
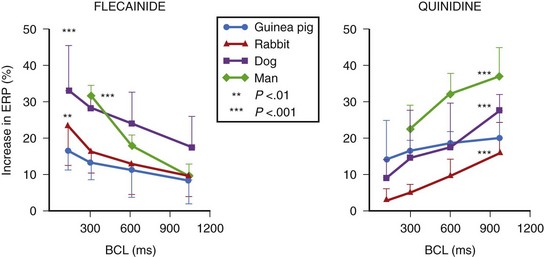
FIGURE 80-3 Rate-dependent effects of flecainide and quinidine on effective refractory period (ERP) in mammalian atria. The data contrast the forward use dependence of flecainide with the reverse use dependence of quinidine (see Figure 80-2).
(From Wang Z, Pelletier LC, Talajic M, Nattel S: Effects of flecainide and quinidine on human atrial potentials: Role of rate dependence and comparison with guinea pig, rabbit and dog tissues, Circulation 82:274–283, 1990.)
Class I Antiarrhythmic Compounds
Conversely, lidocaine and its oral congeners, mexiletine and tocainide, and DPH (class IB agents), in fact, shorten repolarization and hence refractoriness, in addition to slowing conduction. The actions of class I agents are usually more intense in diseased tissues that are partially depolarized, such as in myocardial ischemia. Here, their proarrhythmic effects in settings of ischemia or left ventricular dysfunction are often much greater and may prove fatal—as in the case of flecainide, encainide, and propafenone (class IC compounds), which are contraindicated in patients with significant ventricular dysfunction. As a class of antiarrhythmic drugs, the use of sodium channel blockers is declining because none of the agents has the potential to increase survival by controlling cardiac arrhythmias. If they are administered to patients with potentially serious cardiac disease, the risk of mortality may increase.24 Their main usefulness is in the conversion of AF to sinus rhythm and in maintaining the stability of sinus rhythm in patients in whom the arrhythmia occurs with normal or near-normal ventricular function. Relevant aspects of individual agents are presented below.
Quinidine
Quinidine has the dual electrophysiological properties of prolonging repolarization and slowing conduction by blocking inward sodium current, thereby slowing conduction and by blocking a variety of outward potassium currents; the effects of the drug on myocardial ion channels are compared with those of other antiarrhythmic drugs in Table 80-1. The drug is available in injectable and oral forms, but the injectable formulation is now rarely used. Quinidine may increase heart rate and facilitate AV nodal conduction by its vagolytic actions and increase ventricular response in AF. The major electrophysiological effect of clinical utility relative to the drug’s antiarrhythmic actions is the prolongation of the APD and the ERP (a class III action); the effects on both are attenuated as the heart rate increases. The drug has been used in patients with impaired ventricular function, but it may depress myocardial contractility. The elimination half life of quinidine is 8 to 9 hours, its metabolism being largely by hepatic hydroxylation. The usual oral dose of the drug is 1.2 to 1.6 g/day in 8 to 12 hourly divided doses relative to the preparation in use.
Table 80-1 Effects of IKr Inhibition on Effectiveness in Maintaining Sinus Rhythm in AF Relative to the Development of TdP and Total Mortality with and Without Blocking Other Ionic Currents
The most common adverse effects of quinidine include diarrhea, nausea, and vomiting, which occur in one third of all patients. Quinidine can also cause cinchonism (headache, dizziness, and tinnitus), and quinidine can cause syncope, a syndrome characterized by lightheadedness and fainting. The major shortcomings of quinidine are severe diarrhea, frequently caused by the drug, and TdP, which develops in 2% to 5% in association with a prolonged Q-T interval. Rarely, TdP may be fatal. In a detailed meta-analysis of the trials in AF involving quinidine, an increase in mortality has been reported.25 For these reasons, when considered in conjunction with the limited ceiling of effectiveness of the drug in ventricular arrhythmias as well as in supraventricular arrhythmias, the clinical utility of quinidine is limited. Its continued use can be justified only marginally.
Disopyramide
As in the case of quinidine and procainamide, disopyramide as a class IA antiarrhythmic agent has limited clinical use. Its electropharmacologic profile is similar to that of quinidine, but it has a more potent anticholinergic action and it produces a lesser degree of gastrointestinal disturbance. The elimination half life of the drug is about 8 hours, but slow-release formulations have been introduced. The effects of the drug in arrhythmia control have not been extensively studied except for the suppression of premature ventricular contractions (PVCs). Disopyramide has been used largely in the oral form at a dose of 100 to 200 mg q6h, but it is now rarely used for the control of ventricular tachyarrhythmias. Like quinidine, it does prolong the Q-T interval and may produce a variable incidence of TdP; of particular importance, in patients with impaired ventricular function and in those with heart failure, it is likely to severely aggravate cardiac decompensation by its potent negative inotropic actions. However, such a property may be of therapeutic value in the syndrome of idiopathic hypertrophic cardiomyopathy (HCM), a setting in which the drug produces a greater negative inotropic effect than do β-blockers with which disopyramide can be combined. Although not approved in the United States, disopyramide may have a significant anti-fibrillatory effect in AF in two settings where its role in controlling this arrhythmia may be unique—in patients with HCM who develop AF and in patients whose AF is entirely caused by excessive vagal tone.26 In the latter setting, the drug’s anticholinergic actions may be a specific therapy, which might be strikingly effective. It should be emphasized, however, that the drug’s anticholinergic actions may lead to serious adverse reactions in the form of urinary retention, worsening of glaucoma or myasthenia gravis, and severe constipation.
Lidocaine, Mexiletine, and Tocainide
The properties and the roles of intravenous lidocaine and its two orally active congeners, mexiletine and tocainide, as class IB compounds, will be discussed only briefly because their roles have declined considerably in recent years and they are likely to be only of historic interest. Despite the suppression of ventricular arrhythmias that class IB agents produce, data on their adverse effects on mortality continue to accrue.27,29 Lidocaine, a class I antiarrhythmic, is available as an injection. Lidocaine action is characterized by a fast, on-off block of sodium ion channels in both the activated and inactivated states, with a preference for inactivated state sodium channels. It also shortens the duration of the APD (hence the Q-T interval), and refractoriness, and has been shown in the experimental setting to elevate the ventricular defibrillation threshold. Thus, the drug and its oral congeners (also available as intravenous formulations) may act largely by slowing conduction rather than prolonging refractoriness. However, their actions in terminating and preventing arrhythmias may also stem from the conversion of unidirectional block to a bidirectional block in the arrhythmia re-entrant circuit although the proof for this in the clinical setting is lacking. Lidocaine is metabolized rapidly in the liver, and it is administered generally as an initial bolus of 100 to 200 mg, followed by 2 to 4 mg/min for varying durations of time (usually 24 to 36 hours) aiming at serum levels of 1.4 to 5 µg/mL.
Lidocaine gained prominence as a prophylactic agent in the early days of the inception of coronary care units and subsequently as the first-line agent in the control of VT/VF in all acute care units, including the emergency department, and in the resuscitation of those developing out-of-hospital cardiac arrest. The use of lidocaine and, indeed, other antiarrhythmic drugs in these settings was not based on data from controlled clinical trials. It is of interest to note that in a direct blinded comparison, intravenous lidocaine converted 18% of VT to sinus rhythm compared with 69% conversion with intravenous sotalol.30 The Cardiac Arrhythmias Suppression Trial on the drugs encainide, flecainide, and moricizine indicated a dichotomy between arrhythmia suppression and mortality.1 Abundant data now suggest that intravenous lidocaine and mexiletine are similarly ineffective in the setting of postmyocardial infarction and raise the issue that they might also be similar in the control of destabilizing VT or VF as well as in the survivors of out-of-hospital cardiac arrest.27–29 It is not surprising that in line with the data, the most recent AHA/ACC/ACLS (American Heart Association/American College of Cardiology/Advanced Cardiac Life Support) Guidelines have relegated the use of lidocaine as being “indeterminate.”2 This issue will be discussed in depth later in this chapter when the effects of intravenous lidocaine and intravenous amiodarone will be discussed relative to the broader implications of the choice antiarrhythmic agents in the control of life-threatening ventricular arrhythmias in various clinical settings.
Class 1c Agents
This class of antiarrhythmic agents developed on the basis of the belief that the suppression of ambient arrhythmias—whether PVCs, sustained VT, or nonsustained VT—by antiarrhythmic drugs should result in prevention of arrhythmic death and in the prolongation of survival. The fact that such a hypothesis was not vindicated has had a number of therapeutic consequences: (1) near-complete or complete suppression of PVCs in the patient with previous MI is associated with an increased mortality rate in those with cardiac disease and indicates that this class of drugs cannot be used with impunity even for the control of symptoms caused by arrhythmias; (2) increased observed mortality induced by class IC agents may be a property of class I agents in general and is also found in the case of lidocaine; and (3) data do not exclude the possibility for using such agents for controlling arrhythmia symptoms or for restoring and maintaining sinus rhythm in AF or AFL in patients without structural heart disease. This is especially so in the case of flecainide and propafenone. Moricizine is often included in the category of class IC agents, but its properties are difficult to classify, and it may not have any advantages over flecainide or propafenone. These agents slow conduction velocity profoundly but have little, if any, effect on refractoriness in ventricular tissues; and theoretically, they eliminate re-entry by slowing conduction to a point where the impulse is extinguished and cannot propagate further. This may be the basis of their effectiveness in markedly reducing ventricular ectopy. Their actions in atrial tissues differ markedly compared with those in the ventricle. They increase the ERP in the atria as a function of increases in heart rate, an effect that is likely to be the basis of their effectiveness in restoring and maintaining sinus rhythm.23 This is their major clinical utility as antiarrhythmic drugs.
Flecainide
Flecainide is a powerful blocker of sodium channels in virtually all cardiac tissues, but it does not significantly inhibit the pacemaker current or the calcium channels. It has effects on K+ channels but without a major effect on repolarization, except in the atria, where repolarization is prolonged and the effective period is lengthened. The drug has a significant negative inotropic effect especially at higher doses. The plasma half-life of the drug is 13 to 19 hours; two thirds of it is metabolized in the liver. The usual dose of flecainide is 100 to 300 mg, given twice daily. The intravenous formulation is available but is not used routinely. One clinical utility of flecainide is in the control of intractable symptoms caused by PVCs in patients with no significant cardiac disease. Perhaps its greatest value is in the restoration and prevention of recurrences of AF but only in patients without structural heart disease because of its serious proarrhythmic reactions in patients with cardiac disease.31 In the prophylactic control of AF or AFL, the drug should be combined with an AV nodal blocking drug (β-blockers or calcium channel blockers) to avoid the development of facilitated conduction across the AV node as the atrial rate slows under the action of the drug. In recent years, single oral doses (200 to 300 mg) of the drug have been successfully used for the acute termination of paroxysms of AF (“pill in the pocket” approach).
Propafenone
Propafenone is also a powerful class IC agent with an electrophysiological activity profile similar to that of flecainide; it also has a mild degree of β-blocking action, which does not appear to be clinically relevant.32 Its actions in the atria may also be similar to those of flecainide, and the drug does not prolong repolarization in ventricular tissues. The drug is rapidly absorbed with the bioavailability of 50%, its elimination half-life being 2 to 10 hours in normal subjects and 12 to 32 hours in poor metabolizers. Orally, propafenone is administered as 150 to 300 mg three times daily, and a longer acting formulation has been introduced. The drug can also be administered intravenously and as single oral doses (300 or 600 mg) for the acute conversion of paroxysms of atrial AF. As in the case of flecainide, propafenone should not be used in patients with significant cardiac disease, and its two main indications are for the suppression of resistant symptomatic PVCs and for the restoration and maintenance of sinus rhythm in the case of AF in patients with structurally normal hearts. Its efficacy rivals that of flecainide, although direct comparisons have not been made. As in the case of flecainide, propafenone should be combined with an AV nodal blocking drug to reduce the possibility of accelerated conduction with the slowing of atrial rate induced by the drug.
β-Adrenergic Blockers as Antiarrhythmic and Anti-fibrillatory Compounds
β-Blockers as a class of drugs exert distinctive antiarrhythmic and anti-fibrillatory effects because of their properties of consistently alleviating symptoms and prolonging survival in a wide subset of patients. The electrophysiological and anti-fibrillatory effects are most striking in the clinical context of most intense sympathetic stimulation. They prevent the development of VF in a variety of experimental animal models. The beneficial effects in this setting could not be accounted for by any known electrophysiological mechanisms. However, in the clinic, the antiarrhythmic potential of β-blockade was greatly overshadowed by its anti-ischemic actions for which this class of drugs was synthesized. Thus, the recognition of the fact that blunting the effects of catecholamines on the heart might be a potent anti-fibrillatory mechanism was slow in coming. It has been known for many years that increased activity of the sympathetic nervous system might induce cardiovascular morbidity and mortality through a variety of mechanisms. For example, given the appropriate pathologic substrate, increased sympathetic activity may be associated with sudden arrhythmic death.33–35 This is now known to be particularly striking in the case of patients developing MI with or without heart failure.
The intrinsic effects of β-blockers may be modified to varying extents by the associated pharmacologic properties that individual agents may have. For example, in the case of propranolol at high concentrations the sodium channel is significantly inhibited. Some agents (e.g., acebutolol, atenolol, bisoprolol, carvedilol, and metoprolol) are relatively cardioselective for blocking β1-adrenoceptors, and others are nonselective (propranolol, nadolol, timolol, and sotalol) with respect to β1– and β2-adrenoceptors. However, evidence that these varying degrees of selectivity of action significantly alter the antiarrhythmic and anti-fibrillatory actions of β-blockers in patients is scarce. However, little doubt exists that the presence of marked agonist actions (e.g., in pindolol) is associated with often significant increases rather than decreases in heart rate that largely offset the antiarrhythmic and anti-fibrillatory actions of a given agent.36
Electropharmacologic Properties and Anti-fibrillatory Actions
The precise effects of β-antagonists on ionic currents in differing myocardial cells in terms of depolarization and repolarization are difficult to characterize.37 It should be recognized that for a given agent within the broad class of these compounds, the effects may stem from their intrinsic properties of blocking β-receptors as well as from their associated pharmacologic actions. The dominant effect, however, is from β-receptor blockade, which has minimal effects on calcium channels (ICa-L) or various potassium channels (IK). It is known that sympathomimetic amines exert a stimulant effect on the pacemaker current (If), which is markedly inhibited by β-blockers. Indeed, the blocking effect of the pacemaker current by β-blockers is the most readily defined pharmacodynamic property of this class of drugs and one that correlates well with the beneficial effects on mortality in patients with cardiac disease. The effects of β-blockade on the nodal tissues are, therefore, significant with the slowing of the heart rate by effects in the sinoatrial (SA) node and by prolonging refractoriness in the AV node, which may be of clinical utility in terminating SVTs and slowing the ventricular response in the setting of AFL and AF.
Antiarrhythmic Actions of β-Blockers
Perhaps, the antiarrhythmic and anti-fibrillatory actions of β-blockers are best characterized in terms of their property of attenuating the deleterious effects of excess catecholamines. The electrophysiological consequences of sympathetic hyperactivity have been extensively documented in numerous experimental and clinical studies.33–35 In the experimental setting, they have included (1) shortening of the ventricular APD, and hence the ERP; (2) augmenting ventricular conduction; (3) increasing ventricular automaticity; (4) reducing vagal tone; (5) decreasing VF threshold; and (6) the reversal or attenuation of the effects of antiarrhythmic drugs being administered in the expectation of preventing arrhythmic deaths. Conversely, it is known that the depletion of the adrenergic transmitters to the heart increases VF threshold; and in experimental models in which VF could be induced reproducibly, the arrhythmia is preventable by sympathetic blockade.33 Aspects of the anti-fibrillatory effects of β-adrenergic blocking drugs are shown in Figure 80-4.36 In fact, the appreciation of such an anti-fibrillatory effect was the basis for classifying it as a class II antiarrhythmic action.3–8
β-Blockade
Impact on Sudden Cardiac Death and Total Mortality in Survivors of Myocardial Infarction and in Patients with Heart Failure
β-Adrenergic blocking drugs are effective in reducing mortality in many subsets of patients with manifest arrhythmias and in those at high risk of dying from arrhythmic deaths.37–42 For example, they reduce death rates in patients with congenital long Q-T syndrome (LQTS), in survivors of cardiac arrest, and in selected cases of VT, although the data supporting these conclusions have not always been from controlled clinical trials.39–43 However, they are in line with the compelling data from randomized, placebo-controlled β-blocking trials, in which consistent and significant decreases in mortality have occurred, especially in survivors of acute MI (AMI) and in those with congestive heart failure.44–51 These trials have usually been of adequate sample size and of acceptable protocol design with features that include double-blind comparison in placebo-controlled studies. They have shown that these agents, as a class, not only bring about a beneficial change in total mortality but also on sudden, presumably arrhythmic death (Table 80-2). The overall clinical utility and versatility of this class of antiadrenergic compounds are well documented as indicated in Table 80-3.36
Table 80-2 Principal Uses of β-Blockers to Reduce Life-Threatening Ventricular Arrhythmias and Control Postoperative Atrial Fibrillation After Cardiac Surgery
INDICATIONS | COMMENTS |
---|---|
Cardiac arrest survivors | No controlled clinical trials but uncontrolled data compelling |
Adjunctive therapy to ICDs | |
Controlled data still to be obtained, but support for VT/VF strong from large uncontrolled database | |
Congenital long QT syndrome | Compelling but uncontrolled data used a single drug modality, now being replaced with ICDs, with which they often need to be combined |
MI survivors | Most consistent antiarrhythmic agents increase survival in this setting, reduce total mortality, sudden death, and re-infarction rates |
Overwhelmingly positive data from controlled clinical trials | |
Congestive cardiac failure | Reduce total mortality and sudden death in CHF of ischemic and nonischemic origins: role well established |
Prevention of AF | Prophylactic administration reduces the incidence of AF in postoperative cardiac patient undergoing cardiac surgery |
AF, Atrial fibrillation; ICDs, implantable cardioverter-defibrillators; MI, myocardial infarction; VT/VF, ventricular tachycardia/ventricular fibrillation.
Table 80-3 Actions of Intravenous Amiodarone Compared with Long-Term Amiodarone
actions | INTRAVENOUS AMIODARONE | long-term AMIODARONE |
---|---|---|
Repolarization (Q-T interval) prolongation (atria and ventricles) | + | ++++ |
Conduction velocity (atria and ventricles reduced) | ++ | ++ (as a function of rate) |
Sinus rate reduced | + | +++ |
AV nodal conduction slowed | + | ++ |
AV nodal refractoriness increased | ++ | +++ |
Atrial refractoriness increased | + | +++ |
Ventricular refractoriness increased | + | +++ |
Noncompetitive α- and β-blocking activities | + | ++ |
AV, Atrioventricular.
β-Blockers, as a class, consistently reduce the incidence of SCD and total mortality in the survivors of AMI and in patients with cardiac failure of ischemic and nonischemic origins. In the case of patients with MI, beneficial effects on SCD and total mortality have been documented at the time of diagnosis out of the hospital, during hospital stay, and following discharge from the hospital. In the latter case, SCD and total mortality were reduced from 18% to 39% during the first year. It is presumed that the benefit in survival is from the prevention of VF. It is noteworthy that with β-blockers, mortality is reduced over the first 10 days when the drugs are given in an initial intravenous dose followed by oral therapy as well as when they are given in conjunction with thrombolytic therapy during the early stages of AMI. Two other features of the response to β-blockers in this setting should be emphasized. First, unlike trials such as CAST, β-blocker trials have not been arrhythmia suppression trials, but the degree of benefit on survival correlated linearly with the degree of heart rate reduction, in the case of MI as well as with cardiac failure.1 Second, evidence from two post-MI trials showed that total mortality reduction was greater when amiodarone was administered in combination with β-blockers versus when it was used alone in the drug treatment limb.52–54
As summarized recently, β-blockers, given in controlled and graduated dose regimens, have been shown to variably but significantly reduce mortality in subsets of patients with advanced congestive cardiac failure.5,55–61 The outcomes of three recent trials with three different β-blockers should be emphasized (Table 80-4).56,57,60
In the trial involving the use of metoprolol in 3991 patients with largely New York Heart Association class II to IV heart failure (LVEF <40%), randomized to long-acting metoprolol (n = 1990) in graduated doses of up to 200 mg/day or placebo (n = 2001), the primary endpoint was all-cause mortality, analyzed by intention to treat. The mean follow-up was 1 year. During the period of follow-up, 147 deaths (7.2%) occurred on metoprolol versus 217 deaths (11.0%) on placebo (P < .00009). A statistically significant reduction in SCD on metoprolol was seen compared with placebo.56
Similar data have been reported from a double-blind, multi-center study on the drug bisoprolol in 2647 patients randomized to a β-blocker (n = 1327) or placebo (n = 1320). The patients were all in classes III and IV with left ventricular ejection fraction (LVEF) of 35% or lower and all were taking diuretics and angiotensin-converting enzyme (ACE) inhibitors as in the case of Metoprolol Controlled-Release Randomised Intervention Trial in Heart Failure (MERIT-HF). All-cause mortality was 11.8% (156 deaths) versus 17.3% (17.3%). This difference was significant (P < .0001). The drug was effective in reducing SCD significantly. Cardiovascular deaths on bisoprolol were significantly fewer, fewer patients were admitted to the hospital, and the difference for the combined endpoints was also significant. The numbers of permanent treatment withdrawals were similar for bisoprolol and placebo. In the case of carvedilol, a number of relatively smaller individual trials have been conducted, but the cumulative data have been sufficiently compelling for making it the first β-blocker to be approved for use in congestive heart failure in the United States.60 The composite data from all the trials of β-blockers performed to date support the routine use of this mode of prophylactic therapy in all patients in whom β-blockers are not contraindicated.
Drugs Acting by Prolonging Repolarization
Of the currently available antiarrhythmic compounds, β-blockers, sotalol, amiodarone, ibutilide, and dofetilide are likely to remain the prototypes of the drugs of the future. Amiodarone and sotalol are of the greatest interest.62–67 They prolong repolarization and refractoriness in atria and ventricles while blocking sympathetic stimulation, although by differing mechanisms. Their propensities to decrease heart rate and to modulate the AV node function, and thereby slow ventricular response in AF and AFL, have additional value for the control of many arrhythmias. Thus, they have the greatest clinical utility for controlling ventricular as well as supraventricular arrhythmias. Their respective roles are further bolstered by controlled trials showing superiority over class I agents in patients with VT or VF.68,69 The perception that in spite being powerful sympathetic antagonists, sotalol and amiodarone acted dominantly by prolonging the APD led to the search for pure compounds devoid of other associated properties having simpler adverse effect profiles.70–73 Intravenous amiodarone has recently been studied extensively in clinical trials in destabilizing VT or VF in the hospital and in shock-resistant VF following out-of-hospital cardiac arrest.2,74–78
Current data suggest that compounds that induce an isolated or “pure” prolongation of the APD accompanied by a proportional lengthening of myocardial refractoriness may be anti-fibrillatory.37 Under certain circumstances, they have advantages over complex compounds such as sotalol or amiodarone. This has been the case with dofetilide, ibutilide, and azimilide.79 The properties of tedisamil, which is also under development, are somewhat more complex.37 Such compounds may be the result of the block of a single repolarizing ionic current (e.g., dofetilide) or multiple currents (ibutilide, tedisamil, or azimilide). Thus, for the present, it would appear that two subclasses of such agents might be appropriate to develop—those that are either safer and more effective chemical derivatives of sotalol and amiodarone or those that are relatively pure prolongers of repolarization and refractoriness. All such agents may need to produce a homogeneous lengthening of repolarization by selectively inhibiting multiple or single ionic channels in the myocardium with a minimal proclivity to cause TdP.
Amiodarone as a Versatile and Complex Class III Agent
Amiodarone was synthesized as an antianginal drug in 1962, but attention was not drawn to the compound for its unique properties until 1970 when Singh and Vaughan Williams showed the properties of the drug to prolong cardiac repolarization, with anti-fibrillatory properties and the potential for slowing heart rate because of its nonspecific antiadrenergic actions.4 Since these early studies, it has become increasingly evident that amiodarone is an exceedingly complex antiarrhythmic compound (Figure 80-5).
The electrophysiological effects of amiodarone administered acutely and after a period of long-term drug therapy differ markedly (see Table 80-3), both associated with distinct and potent antiarrhythmic actions.36 The electropharmacologic effects of the compound are multi-faceted.80 The most striking long-term property is the ability of the drug to increase the APD in atrial and ventricular tissues following long-term drug administration. Little or no effects occur in Purkinje fibers and M cells, a phenomenon that leads to the reduction in QT dispersion.81–83 The drug is also unique in that its effect on repolarization is not influenced by heart rate.84,85 In isolated myocardial fibers, it eliminates the tendency for the development of early afterdepolarization (EAD) produced in Purkinje fibers and in M cells. The ionic channels that amiodarone blocks after long-term drug administration are not fully defined, but it has been shown to block Ito and IK as well as INa and Ica.64 It abolishes EADs induced in isolated cardiac tissue.84 In humans, despite producing marked slowing of the heart rate and considerable increases in the Q-T or QTc interval, the drug has a very low propensity for producing TdP.84 Amiodarone rarely exhibits proarrhythmic actions typical of class I agents, although it is a fairly potent sodium channel blocker, especially at high heart rates. The salient pharmacodynamic features of amiodarone are summarized in Figure 80-5.
Amiodarone has a broad-spectrum antiarrhythmic effect with a varied adverse effect profile, which is generally dose and duration dependent. Included in Table 80-5 are the main potential clinical indications that have been accepted. Intravenous amiodarone is effective in the control of hemodynamically significant and refractory VT or VF (to lidocaine and procainamide), with a potency at least as high or higher than that of intravenous bretylium.74,75 The drug exerts a powerful suppressant effect on PVCs and nonsustained ventricular tachycardia (NSVT) and provides control in 60% to 80% of recurrent VT or VF when conventional drugs have failed during continuous oral therapy.86 In only a small number of patients does the drug prevent inducibility of VT or VF, there being little or no systematic relationship between the prevention of inducibility of VT or VF and the long-term clinical outcome.87
Table 80-5 Major Uses of Amiodarone in the Control of Ventricular and Supraventricular Arrhythmias
INDICATIONS | COMMENTS |
---|---|
Cardiac arrest survivors | Superior to class I agents (guided therapy); tolerated in VT/VF and in all levels of LVEF and CHF; low incidence of torsades de pointes Increasingly perceived as the most potent antiarrhythmic agent, especially in the setting of low LVEF but being increasingly replaced with ICDs for primary therapy in many subsets of patients with VT/VF May increase VT defibrillation threshold; clinical significance unclear |
Adjunctive therapy to ICDs | Controlled data still to be obtained but support for VT/VF is strong from large uncontrolled database; preferred agent in patients with significantly impaired LVEF and CHF |
Conversion of VT to SR by IV regimen | Precise efficacy not known |
Intravenous amiodarone in destabilizing refractory VT/VF | Superior to lidocaine and procainamide; efficacy equal to that of bretylium but with less hypotension; increases survival to hospitalization in patients with out-of-hospital cardiac arrest (ARREST and ALIVE trials) First-line antiarrhythmic agent in cardiac arrest resuscitation |
Post-MI survivors | Numerous uncontrolled positive mortality studies; two recent placebo-controlled studies—EMIAT and CAMIAT—significant reduction in arrhythmic deaths, trend toward total mortality reduction in one of the studies, augmented effect, if combined with β-blockers Meta-analysis of all data consistent with reduction in total mortality and in sudden death while on drug |
Congestive cardiac | Two controlled trials, one blinded placebo-controlled (CHF STAT) and one blinded (GESICA) indicate a spectrum of failure effects from reduction in total mortality (GESICA) and neutral (CHF STAT) with a trend in selective favorable effects in nonischemic cardiomyopathy Amiodarone increased LVEF |
ATRIAL FIBRILLATION | |
Acute conversion | Variable efficacy but systematic placebo-controlled study against a comparator agent not available |
Maintenance of SR | Up to 60% or greater at 12 months after restoration of SR; maintains ventricular on relapse to atrial fibrillation on oral drug (controlled trials in progress) |
Effect on AF prevention in postoperative cardiac surgery | Preoperative administration of oral drug reduces incidence of postoperative AF; IV drug given postoperatively may shorten hospital stay by reducing AF. |
AF, Atrial fibrillation; CHF, congestive heart failure; ICD, implantable cardioverter-defibrillator; IV, intravenous; LVEF, left ventricular ejection fraction; MI, myocardial infarction; SR, sinus rhythm; VT/VF, ventricular tachycardia and ventricular fibrillation; ARREST, Amiodarone in Out-of-Hospital Resuscitation of Refractory Sustained Ventricular Tachyarrhythmias trial; ALIVE, Azimilide Post-Infarct Survival Evaluation trial; EMIAT, European Myocardial Infarct Amiodarone Trial; CAMIAT, Canadian Amiodarone Myocardial Infarction Arrhythmia Trial; CHF STAT, Congestive Heart Failure Survival Trial of Antiarrhythmic Therapy; GESICA, Grupo de Estudio de la Sobrevida en la Insuficienca Cardiaca en Argentina.
The properties of amiodarone during long-term administration permit predictable control of recurrent paroxysmal SVTs, slowing of the ventricular response in AF and AFL, and maintaining the stability of sinus rhythm after chemical or electrical conversion.88 Amiodarone is the most potent drug for maintaining the stability of sinus rhythm in patients converted from AF. The drug is currently being compared with placebo and sotalol in a number of major multi-center studies.
Amiodarone is effective in converting AF to sinus rhythm both in valve disease as well as in patients with nonrheumatic AF.88,89 With respect to AF occurring after cardiac surgery, the drug has been studied in three different trials.90–92 Hohnloser and colleagues studied amiodarone in a randomized, double-blind, placebo-controlled study in 77 patients after coronary artery bypass surgery.90 They gave a 300-mg bolus of intravenous amiodarone followed by 1200 mg daily every 24 hours for 2 days and 900 mg daily for the next 2 days. At the end of the study period, the overall incidence of AF in the placebo group (n = 38) was 21%; in the amiodarone group (n = 39) it was 5% (P < .05).