Chapter 104 Aneurysm and arteriovenous fistula of the liver and pancreatic vasculature
Overview
Traumatic injuries to the hepatic pedicle generally occur in conjunction with injuries to the liver or pancreas. Hemorrhage or hematoma at the porta hepatis can be indicative of injury to the hepatic artery, portal vein, or both. In blunt trauma, injury is often secondary to compression or shearing forces and disruption at the points of maximal fixation, where the porta hepatis is relatively fixed to the retroperitoneum. An isolated pedicle injury may occur with penetrating trauma, which may involve intrahepatic or extrahepatic vessels with disruption of any structure in the trajectory; see Chapters 102 and 103 for further discussion on traumatic injuries.
Vascular control can be attempted using the Pringle maneuver (1908) or by clamping the porta hepatis; this controls arterial and portal vein hemorrhage from the liver. Continuing hemorrhage is due to retrograde bleeding from the vena cava or hepatic veins. Kocherization of the duodenum and head of the pancreas by dividing the peritoneum along the lateral border of the duodenum allows for better exposure (see Chapter 63A). This maneuver is especially useful for distal pedicle injuries or those to the retropancreatic portion of the portal vein.
In cases of severe injury, hepatic arterial ligation can be used in the operating room or with the aid of angiography; this should be considered if the patient has a noticeable decrease in bleeding after the Pringle maneuver is performed. Complete selective hepatic arterial ligation is used in approximately 1% of patients (David et al, 2000). Disruption of the right or left hepatic artery may be treated by ligation, providing the ipsilateral portal vein is intact. Simple lacerations of the common hepatic artery may be repaired primarily, but blind placement of sutures should be avoided because of the proximity of the common bile duct and potential for iatrogenic injury. With vascular injuries to the pedicle, common bile duct injury should be suspected, and a cholangiogram should be performed. If right or common hepatic artery ligation is performed, or if injury to the common bile duct is evident, a cholecystectomy should be performed.
Injuries to the portal vein are difficult to manage because of profuse bleeding and the relative inaccessibility of the portal vein owing to its posterior position in the porta hepatis. These injuries are associated with a 50% to 70% mortality rate and carry a high risk of other vascular injuries (Asensio et al, 2007; Buckman et al, 2001; Coimbra et al, 2004; Fraga et al, 2009; Pearl et al, 2004). Shock or systolic blood pressure below 90 mm Hg is a significant predictor of survival, and 90% of injuries to the portal vein are caused by penetrating trauma; the most common location of injury is within the hepatoduodenal ligament. The three classifications of portal vein injuries are type A, less than 50% of vein circumference is disrupted; type B, disruption is greater than 50%; type C, complete transection is evident (Coimbra et al, 2004).
Primary venorrhaphy is the preferred approach for repair of portal vein injuries in the stable patient (Fraga et al, 2009; Pearl et al, 2004). The hemodynamic status of the patient generally determines the complexity of the repair. Other alternatives are end-to-end anastomosis, portocaval shunt, graft interpostition, vein patch graft, and portal vein ligation (Coimbra et al, 2004; Fraga et al, 2009; Pearl et al, 2004; Stone et al, 1982). Portal vein ligation can be performed only if the hepatic artery is intact, and it is an appropriate damage control option in an unstable and coagulopathic patient. Portal vein ligation has been reported with a 50% to 80% survival rate and low incidences of subsequent portal hypertension (Coimbra et al, 2004; Stone et al, 1982). Following ligation of the portal vein or superior mesenteric vein (SMV), bowel will become edematous with areas of ischemia. Most surgeons would recommend temporary abdominal closure followed by a second-look laparotomy in 48 to 72 hours. Injury to both the hepatic artery and portal vein are rare and technically difficult to manage, and at least one of these structures must be repaired to avoid hepatic necrosis.
SMV injuries are uncommon but devastating; this is due to the difficulty in obtaining exposure and getting control of hemorrhage quickly. Primary venorrhaphy is the preferred repair, when such is possible; although ligation of the SMV has been performed with variable success, it should be reserved for use in the hemodynamically unstable patient (Asensio et al, 2000, 2007). It should also be noted that superior mesenteric venous injuries are often associated with portal vein and other vascular injuries (Asensio et al, 2007).
Hepatic Artery Aneurysms and Pseudoanerysms
Visceral artery aneurysms are rare, with an average incidence of approximately 0.1% to 2% (Hossain et al, 2001; Panayiotopoulos et al, 1996). Splenic artery aneurysms account for approximately 60% of all visceral aneurysms, followed by hepatic artery aneurysms, which constitute 20% (Abbas et al, 2003). Hepatic artery aneurysms were initially described by Wilson in 1809 (O’Connor et al, 1995). The first successful surgical repair was performed by Kehr in 1903, in which he ligated the feeding vessel proximal to the hepatic artery (Carr et al, 1996; Guida & Moore, 1966). Within the past 2 decades, endovascular therapy has been utilized with increasing frequency, and O’Connor and colleagues reported successful endovascular stent exclusion of a hepatic artery aneurysm in 1995.
Hepatic artery aneurysms are more common in men, with a 2 : 1 prevalence (Abbas et al, 2003; Panayiotopoulos et al, 1996). The mean age of presentation is generally around 60 years of age, but hepatic artery aneurysms have been reported in all age groups (Abbas et al, 2003). The etiology of nontraumatic hepatic artery aneurysms remains unclear. True aneurysms undergo dilation and thinning of the vessel wall, although the blood is still contained within the vessel wall (Ikeda et al, 2008). A common histopathologic feature is medial degeneration of the arterial wall, which may be congenital but is further stressed by various factors (Abbas et al, 2003; Panayiotopoulos et al, 1996). Atherosclerosis is present in approximately 30% of aneurysms, but it is debatable whether it is a causative event or a secondary phenomenon (Messina & Shanley, 1997).
Several other conditions have been associated with hepatic artery aneurysms; these include arterial fibrodysplasia, vasculitis, polyarteritis nodosa, systemic lupus erythematous, tuberculosis, Marfan syndrome, Ehler-Danlos syndrome, arteritis, and hyperflow conditions (Abbas et al, 2003; Ikeda et al, 2008; Panayiotopoulos et al, 1996; Messina & Shanley, 1997). In the early twentieth century, most true hepatic artery aneurysms were mycotic, with bacterial endocarditis being the most common cause. With the advent of antibiotics, this form is generally only found in intravenous drug users (Shanley et al, 1996a).
Approximately 50% of all hepatic artery aneurysms are false aneurysms, or pseudoaneurysms, caused by a ruptured arterial wall with subsequent periarterial hematoma formation. These are generally the result of trauma, iatrogenic injury, inflammation, or infection (Carr et al, 1996; Guida & Moore, 1966). Pseudoaneurysms that result from iatrogenic injury to the hepatic arterial system are usually secondary to biliary, hepatic, or pancreatic interventions and to the laparoscopic or open treatment of abdominal and retroperitoneal pathologies (Bulut et al, 2002; Duce et al, 2002; Grego et al, 2003; Ikeda et al, 2008; Larson et al, 2002; Tessier et al, 2003; Tulsyan et al, 2007; see Chapters 28, 33, 34, and 54). Aneurysms resulting from laparoscopic cholecystectomy frequently affect the right hepatic artery but may be confined to the cystic artery remnant (Tessier et al, 2003). Pancreatitis and other inflammatory diseases can damage the arterial wall and lead to pseudoaneurysm formation (Mallick & Winslet, 2004). Additionally, hepatic artery pseudoaneurysm after resection of a hilar cholangiocarcinoma has been reported (Briceno et al, 2008).
Most hepatic artery aneurysms are solitary (80%), either saccular or fusiform, with a maximum diameter ranging from 1 to 14.5 cm (Abbas et al, 2003; Shanley et al, 1996). Other concomitant visceral artery aneurysms are present 31% of the time, and nonvisceral artery aneurysms are evident in 42% (Abbas et al, 2003). The majority, 66% to 80%, occur extrahepatically; however, the incidence of intrahepatic aneurysms may be increasing, compared with extrahepatic aneurysms (Abbas et al, 2003; Messina & Shanley, 1997). This change in presentation is likely the result of increasing use of percutaneous and laparoscopic interventions and also of improved imaging modalities utilized in the trauma setting (Kasirajan et al, 2001).
In one review of reported cases from 1985 to 1995, the right hepatic artery was found to be most commonly affected (47%), followed by the common hepatic artery (22%), the proper hepatic artery (16%), the left hepatic artery (13%), and rarely the cystic artery (1%) (Shanley et al, 1996). The natural history of these lesions is that of progressive growth, and it is probable that increasing size is accompanied by an increasing rate of rupture, in spite of a paucity of supporting evidence (Abbas et al, 2003). Nonatherosclerotic aneurysms carry a higher risk of rupture than atherosclerotic aneurysms (Abbas et al, 2003).
The lifetime risk of rupture for hepatic artery aneurysm ranges from 14% to 80% (Abbas et al, 2003; Carr et al, 2001; Dolapci et al, 2003; Ikeda et al, 2008; Lagana et al, 2006; Panayiotopoulos et al, 1996), and the mortality rate for ruptured hepatic artery aneurysms is 3% to 40% (Abbas et al, 2003; Ikeda et al, 2008; Panayiotopoulos et al, 1996; Shanley et al, 1996; Tessier et al, 2003). Rupture can occur into the peritoneal cavity (43%), biliary tree (41%), gastrointestinal (GI) tract (11%), or portal vein (5%) (Carr et al, 2001). Abdominal pain is often present, usually in the epigastrium with radiation to the back or right shoulder. Approximately 60% of patients with rupture come to medical attention in hypovolemic shock.
In 1871, Quincke described jaundice, hemobilia (see Chapter 105), and biliary colic in a patient with a hepatic artery aneurysm. These symptoms have become known as the classic triad, but in actuality, they are present in fewer than 40% of cases (Messina & Shanley, 1997). These symptoms are caused by erosion into the biliary tree, which causes intraductal clot or external compression of the bile duct (Messina & Shanley, 1997); rupture into the bile duct causes hemobilia. Occasionally, with a large hepatic artery aneurysm, a mass may be palpated in the upper abdomen, often with an associated bruit or thrill; however, pulsatile masses and bruits are uncommon in intact aneurysms. Expanding aneurysms can cause upper abdominal discomfort with pain radiating into the back, and pseudoaneurysms that result from placement of transhepatic biliary drainage catheters often manifest with a sentinel hemorrhage and blood in the collection bag.
With recent advances in cross-sectional imaging and arteriography, true hepatic artery aneurysms are being detected earlier in their course. Asymptomatic aneurysms (30% to 60%) may be discovered incidentally during radiologic investigation performed for other indications (O’Connor et al, 1995; Panayiotopoulos et al, 1996; Tulsyan et al, 2007). On plain radiographs, a hepatic artery aneurysm may appear as an eggshell-like calcification in the right upper quadrant (O’Driscoll et al, 1999). If this diagnosis is suspected, further investigation with contrast-enhanced computed tomography (CT) scan or magnetic resonance angiography is warranted to delineate the extent of the lesion and define its relationship to surrounding structures. Angiography (see Chapter 19) can be used to characterize hepatic artery blood flow prior to operative treatment (Messina & Shanley, 1997), but ultrasound is rarely used to diagnose hepatic artery aneurysms. A hepatic artery aneurysm may be discovered during abdominal surgery for another indication and will appear as a calcified nodule on the hepatoduodenal ligament.
Improvements in the quality and availability of cross-sectional imaging (see Chapters 16 and 17) have led to an increasing number of aneurysms being detected prior to rupture. Although it is unclear whether the actual incidence of true hepatic artery aneurysms is increasing, incidental discovery during imaging performed for unrelated reasons has increased. For example, the routine use of CT scan in trauma patients has led to increased detection of false aneurysms of the intrahepatic arterial branches (Messina & Shanley, 1997). Also, the increased use of diagnostic and therapeutic biliary interventions have contributed to the increasing incidence of false aneurysms (Messina & Shanley, 1997).
Recent studies demonstrate that a selective approach can be used to manage asymptomatic hepatic artery aneurysms. Currently, no consensus exists regarding size criteria for intervention, but many advocate that small (<2 cm) lesions can be safely observed (Abbas et al, 2003; Ikeda et al, 2008); however, surgical repair, ligation, or embolization should be considered for aneurysms larger than 2 cm in a patient who is a reasonable operative risk with a life expectancy greater than 2 years. In patients with marginal or poor health and aneurysms that measure 2 to 5 cm, careful observation may be warranted (Abbas et al, 2003). The presence of multiple aneurysms is a risk factor for rupture and warrants intervention. Likewise, it is generally recommended that all pseudoaneurysms should be treated, given the high risk of rupture that they pose.
Although some centers allow for a degree of permissive hypotension, the management of ruptured hepatic artery aneurysms consists of volume resuscitation and correction of coagulopathy followed by definitive treatment involving surgical repair, embolization, occlusion, or endovascular stenting (Carr et al, 2001). Occasionally, angiographic intervention may be used as an adjunct prior to repair. In the elective setting, the choice of open surgical repair versus endovascular treatment depends on many factors, including the size and anatomy of the aneurysm, the shape of the sac and its accessibility to endovascular device placement or embolization, the need to restore arterial continuity, and the general health of the patient. A past failure of endovascular intervention does not necessarily preclude further attempts.
Open repair of hepatic artery aneurysms depends on the anatomy and location of the aneurysm, collateral blood flow, operative risk, and clinical status. In the elective setting, the anatomy of the aneurysm, delineation of arterial circulation, and identification of collateral vessels in the port hepatic are ideally determined preoperatively by angiography. In general, aneurysms of the common hepatic artery may be ligated, with or without resection of the aneurysm sac, as long as the portal vein is patent. Reconstruction is usually unnecessary secondary to collateral circulation via the gastroduodenal and right gastric arteries. Failure to confirm adequate collateral vessel circulation can lead to hepatic necrosis (Rokke et al, 1997).
If the patient has underlying hepatic parenchymal disease or is in a state of hemorrhagic shock, reconstruction is recommended, because the liver will be more sensitive to ischemia (Messina & Shanley, 1997; Shanley et al, 1996). Arterial reconstruction is recommended for aneurysms of the proper hepatic artery and its extrahepatic branches, because ligation may interfere with collateral circulation and may induce ischemic necrosis. Autogenous vein grafts, synthetic grafts, aneurysmorraphy with vein patch closure, and aortohepatic bypass have been utilized to restore blood flow. Aortohepatic bypass with saphenous vein or polytetrafluoroethylene (PTFE) graft that originates at the supraceliac aorta is generally preferred when bypass is required.
Intrahepatic aneurysms usually require ligation of the branch hepatic arteries and may require liver resection. Simple ligation of proximal vessels can help control bleeding intrahepatic aneurysms and may be a safer alternative to resection in critically ill patients, despite the risk of necrosis. In all hepatic aneurysms, vascular control can be gained from within the aneurysm, if surrounding inflammation prevents adequate exposure and dissection of adjacent arteries (Messina & Shanley, 1997). Complications after surgical repair are generally related to ischemic necrosis of the liver, abscess formation, and sepsis (Abbas et al, 2003).
Endovascular management of hepatic artery aneurysms has become increasingly popular over the past decade and is particularly useful for treating pseudoaneurysms that arise from iatrogenic injuries (Figs. 104.1 and 104.2). These procedures offer an alternative to conventional open surgical intervention with the benefit of lower morbidity and mortality (Ikeda et al, 2008; Larson et al, 2002; Saltzberg et al, 2005; Tulsyan et al, 2007). Recent studies found rates of technical success from 75% to 98% (Kasirajan et al, 2001; Mallick & Winslet, 2004; Sachdev et al, 2006).
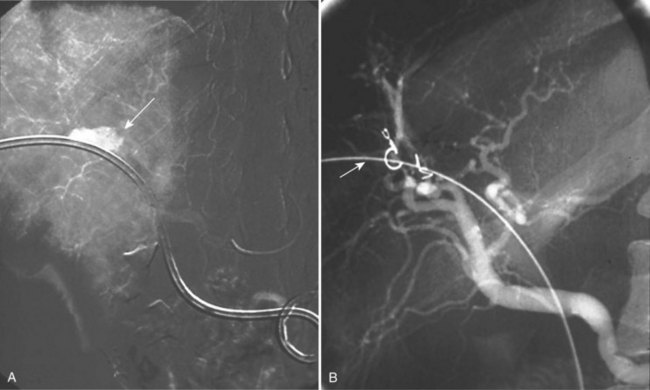
FIGURE 104.1 Hepatic pseudoaneurysm. A, After percutaneous biliary stent insertion (arrow). B, Managed by embolization (arrow).
Various minimally invasive techniques are available to treat aneurysms and pseudoaneurysms. Selective catheterization of the involved vessel and embolization with coils or polymerizing agents has been used for ablation of hepatic artery aneurysms, and percutaneous embolization is being used with increasing frequency in cases of emergent aneurysm rupture (Tulsyan et al, 2007). Endovascular stent grafts allow for preservation of end-organ flow and exclusion of the aneurysm. The arterial anatomy and the location of the aneurysm have a significant impact on the technical ability to place a stent graft (Larson et al, 2002); sufficient normal caliber artery must be available on either side of the aneurysm to allow for a proper stent graft seal and aneurysm exclusion, although further studies are needed to elucidate the long-term durability of stents.
The tortuosity and redundancy of the visceral vessels can increase the difficulty of endovascular interventions. Migration of embolic material and stent graft occlusion are recognized complications of transcatheter treatment. Additional complications include endoleak, failure to completely occlude the aneurysm, hepatic ischemia, and recanalization (Abbas et al, 2003; Kasirajan et al, 2001).
Hepatic Arteriovenous Fistulae and Shunts
Congenital Hepatic Vascular Shunts
The paired umbilical veins bring oxygenated blood to the embryo and course on either side of the liver. When they contact the hepatic sinusoids, the right umbilical vein and portions of the left umbilical vein become obliterated; the persistent left umbilical vein carries blood from the placenta to the fetus. A large channel, the ductus venosus, connects the left umbilical vein to the inferior vena cava to allow oxygenated blood to bypass sinusoid circulation (Gallego et al, 2004). Any deviation from the normal vascular system’s embryologic development can lead to abnormal fistulae and shunting (Fig. 104.3).
Hemangiomas
Some congenital hepatic neoplasms can cause vascular shunting; these include hemangiomas, hepatoblastomas, angiosarcomas, hamartomas, and metastatic neuroblastomas (Burrows et al, 2001; Gallego et al, 2004; Ingram et al, 2000). Infantile hemangiomas are a group of benign vascular tumors with a high rate of endothelial cell proliferation that results in a large mass and dilation of vascular channels, which are often accompanied by arteriovenous (AV) shunting. Following rapid growth, these tumors typically undergo a period of involution and increasing fibrosis (Boon et al, 1996). They are more common in females and may be solitary or multiple. Many small, asymptomatic hemangiomas remain undetected or are discovered on imaging indicated for another reason. Symptomatic presentation may include high-output congestive heart failure, hepatomegaly, anemia, thrombocytopenia, respiratory distress, hemorrhage, jaundice, and hypothyroidism secondary to overproduction of type III iodothyronine deiodinase (Boon et al, 1996; Burrows et al, 2001; Christison-Lagay et al, 2007; Ho et al, 2005; Huang et al, 2000; Konrad et al, 2003; Prokurat et al, 2002; see also Kasabach-Merritt syndromein Chapter 80A). Association with cutaneous hemangiomas and hemangiomatosis of the central nervous system, GI tract, and respiratory tract have been observed (Christison-Lagay et al, 2007). All patients with significant multifocal or diffuse disease should be screened with thyroid function tests and echocardiogram (Dickie et al, 2009).
Magnetic resonance imaging (MRI) is the diagnostic modality of choice for hemangiomas; lesions appear as hypointense relative to liver on T1-weighted images and hyperintense on T2-weighted images (Christison-Lagay et al, 2007; Gallego et al, 2004; see Chapters 17 and 79A). A gadolinium sequence typically demonstrates centripetal enhancement, and multiple flow voids can be present adjacent to or within the lesions (Gallego et al, 2004; Fig. 104.4). Selective arteriography can be used to determine the extent of the lesion and to evaluate the large vessels feeding the tumor, but this is rarely necessary (Stocker, 2001).
Hepatic hemangiomas should be individually assessed based on initial presentation, size, and disease progression (Dickie et al, 2009). Asymptomatic patients without evidence of cardiac failure or significant shunting can be monitored with ultrasonography to assess for change (Christison-Lagay et al, 2007; Isaacs, 2007), and solitary tumors can often be successfully treated by resection (von Schweinitz, 2003). Large, multiple, and diffuse lesions are associated with significant morbidity and mortality; for these, treatment options include medical management, surgery, embolization, radiation, or transplantation (Dickie et al, 2009).
The mortality of large multiple hemangiomas treated with supportive observation has been reported to range from 30% to 80% (Boon et al, 1996; Gallego et al, 2004;). Patients with significant shunting are initially treated with corticosteroids or interferon (IFN)-α with close observation by ultrasonography for spontaneous regression (Deb et al, 2002; von Schweinitz, 2003). Pharmacotherapy failure is an indication for angiographic embolization, but the presence of multiple collaterals can make embolization difficult. When embolizing tumors with a large portal venous component, the portal vein should be done prior to the hepatic arterial component to prevent hepatic necrosis (Burrows et al, 2001). In patients with large hemodynamically significant shunts and cardiac failure, consider early angiographic intervention or ligation. Embolization can be used for interim control of congestive heart failure during pharmacotherapy or prior to operative intervention. Patients with diffuse intrahepatic hemangiomas often respond poorly to pharmacotherapy or other interventions and may require transplantation (see Chapter 97A; Christison-Lagay et al, 2007).
Hepatic Arteriovenous Malformations
True congenital arteriovenous malformations (AVMs) are malformations of the vascular channels and are classified by the predominant endothelium lining the channel and also by flow characteristics (Lima et al, 2005). Blood is shunted through direct arteriovenous connections that have no growth potential and that do not regress spontaneously. They are generally localized to one lobe of the liver (Boon et al, 1996; Gallego et al, 2004; Prokurat et al, 2002) and are usually present in neonates with congestive heart failure, portal hypertension, hepatomegaly, and anemia (Boon et al, 1996). Cardiac failure is noted in 50% to 60% of affected newborns with an associated 50% to 90% mortality rate (Boon et al, 1996; Luks et al, 1991). AVMs can also come to medical attention in late childhood in the clinical setting of hereditary telangiectasia with heart failure, portal hypertension, and hepatic ischemia (Boon et al, 1996; Burrows et al, 2001).
Duplex ultrasound (see Chapter 13) may show a nest of tortuous, dilated vessels with increased venous pulsatility and high peak Doppler shifts in both arteries and veins (Gallego et al, 2004). Contrast-enhanced CT scan shows homogeneous enhancement in the arterial or early portal venous phase with rapid washout of contrast material. MRI shows intrahepatic AVMs as large flow voids and localized masses that are hyperintense on T1-weighted images (Boon et al, 1996). MRI is a useful tool for differentiating AVMs from infantile hemangiomas, because AVMs will show an absence of delayed uptake of contrast material around the hypertrophic vessels (Gallego et al, 2004). Arteriography shows anomalous blood supply and arteriovenous shunting within the vasculature and can help delineate anatomy and resectability criteria.
Many different therapies have been used in the treatment of hemangiomas, including corticosteroids, radiotherapy, embolization, hepatic artery ligation, and hepatic lobectomy (Boon et al, 1996; Gallego et al, 2004; Lima et al, 2005). Super-selective catheterization and embolization (see Chapter 28) with total occlusion of the feeding arteries close to the AVM must be achieved for effective treatment (Lima et al, 2005). Some studies advocate embolization with subsequent hepatic resection (Boon et al, 1996), but complications include incomplete occlusion, rapid development of collateral vessels, and necrosis. Liver transplantation is indicated for very large or diffuse lesions.
Congenital Arterioportal Fistulae
Arterioportal fistulae are characterized by anomalous communication between the arteries and the portal venous system. Congenital arterioportal fistulae are discovered during infancy by portal hypertension, as compared with congestive heart failure with AVMs. Rarely, these fistulae are associated with hereditary telangiectasia, Ehler-Danlos syndrome, and biliary atresia (Burrows et al, 2001; Gallego et al, 2004). Age of presentation varies, but infants with congenital arterioportal fistulae generally come to medical attention within the first year of life. Hepatofugal flow in the portal vein causes arterialization of the portal vein and resultant portal hypertension, which can cause subsequent splenomegaly, gastroesophageal varices, ascites, diarrhea, and intestinal malabsorption (Gallego et al, 2004).
Doppler ultrasound shows arterialization of the portal vein, turbulent flow at the site of the fistula, increased peak velocities, and heterogeneous hepatic parenchyma secondary to vibration artifact (Gallego et al, 2004; Marrone et al, 2006). An enlarged hepatic artery and dilated portal vein segment at the fistula site may also be visible (Gallego et al, 2004). Contrast enhancement of the portal vein on arterial phase images and perfusion anomalies of adjacent hepatic parenchyma are demonstrated by CT scan and MRI (Fig. 104.5; Gallego et al, 2002).
Arteriography with embolization is the preferred therapeutic option, and it should be performed soon after diagnosis to minimize hepatoportal sclerosis and portal radicle fibrosis secondary to progressive portal hypertension (Altuntas et al, 1998). If angiography is unsuccessful, surgical ligation of the arterial feeding vessels may be performed with or without liver resection. Resection of the fistula proper is not necessarily required, although fistulae may recur through arterial collateralization, and patients must be followed closely after intervention. In infants with biliary atresia, liver viability usually depends on arterial inflow, and embolization may precipitate liver ischemia. Liver transplantation is preferred in this subset of patients (Choi et al, 2002).
Acquired Arterioportal Fistulae
The most common causes of acquired arterioportal fistulae are cirrhosis, trauma, iatrogenic trauma stemming from hepatic or biliary intervention, hepatic neoplasms, inflammation, and gastrectomy (Gallego et al, 2004; Marrone et al, 2006; Tomczak et al, 1997). Acquired arterioportal fistulae often present with bleeding from an upper GI source secondary to mucosal congestion, and upper endoscopy shows friable and edematous mucosa. The diagnosis is often missed because patients generally enter a quiescent phase, as collateral circulation forms over the next 6 months to 5 years. As with congenital fistulae, portal hypertension develops and causes GI bleeding and varix formation, splenomegaly, ascites, and intestinal dysfunction (see Chapters 70A and 71; Blumgart & Fong, 2000).
When blood flow through the portal vein is decreased, the hepatic arterial flow increases, causing an arterioportal shunt through multiple anatomic routes (Choi et al, 2002; Park et al, 1990). In localized portal vein obstruction—such as from tumor thrombus or external compression—blood flows from the hepatic artery through the communicating vaso vasorum into distal unobstructed portal vein. In hypervascular tumors, arterial blood may flow directly through the tumor into the portal vein. In patients with increased hepatic vein resistance secondary to Budd-Chiari syndrome or cirrhosis, arterial blood fills the portal vein by retrograde flow through the sinusoids (see Fig. 104.5). In cases of complete obstruction of the portal vein, a peribiliary vascular plexus develops along the intrahepatic and extrahepatic biliary tree.
Contrast enhancement of the portal vein on arterial phase images and perfusion anomalies of adjacent hepatic parenchyma are demonstrated by CT scan and MRI (Gallego et al, 2002). As with congenital arterioportal shunts, treatment options include embolization, surgical correction, and transplantation. Fistulae may recur through arterial collateralization, and patients need to be followed closely after intervention.
Portosystemic Venous Shunts
Portosystemic shunts can be intrahepatic or extrahepatic; congenital shunts are the apparent result of disruption of the complex embryologic process of vitelline, umbilical, and hepatoportal venous involution. In 1793, Abernethy first reported an extrahepatic portosystemic shunt. Two types of extrahepatic portosystemic shunts are found: in type I, the portal vein is congenitally absent, and portal blood flow is completely diverted into the vena cava; in type II, a side-to-side extrahepatic communication exists between the vena cava and portal vein, through which some of the portal flow is diverted to the vena cava (Gallego et al, 2004). These shunts have been observed alone or in combination with other congenital malformations, including biliary atresia (see Chapter 40), genitourinary malformations, mental retardation, and as a part of Goldenhar syndrome (Gallego et al, 2004). Hepatic tumors may also be associated with systemic diversion of portal blood flow.
Congenital intrahepatic portosystemic shunts are abnormal connections between branches of the portal vein and hepatic veins thought to be due to persistent communication between the vitelline veins of the omphalomesenteric system and the sinus venosus (Lane et al, 2000). Infantile hepatic hemangiomas and cutaneous hemangiomas have been associated with these shunts (Gitzelmann et al, 1997). Intrahepatic shunts are classified into four types: 1) a single, large tube that connects the right portal vein to the inferior vena cava, 2) one or more communications present in a single hepatic segment, 3) portosystemic shunt through an aneurysm, and 4) multiple communications between portal and hepatic veins (Park et al, 1990).
Doppler ultrasound may demonstrate abnormal communicating structures between the portal venous branches and hepatic veins and may also reveal an absence of portal vein at the liver hilum. A pulsatile triphasic or biphasic spectral pattern in the portal or splenic veins suggests the presence of a portosystemic shunt (Gallego et al, 2004), the ratio of which can be determined by portal scintigraphy using iodine 123 iodoamphetamine. The isotope is administered rectally, is absorbed into the inferior mesenteric vein, and travels to the liver. When a shunt is present, the isotope can be detected in the liver and lungs simultaneously, and the ratio is determined by dividing the lung counts by the liver counts (Gallego et al, 2004).
Portosystemic venous shunting causes elevated levels of galactose, bile acid, glucose, and ammonia (Gallego et al, 2004). The clinical manifestations may include cataract formation (hypergalactosemia), encephalopathy, liver dysfunction secondary to lack of hepatocyte nutrition, and GI dysfunction. Adults are more susceptible to hepatic encephalopathy than young children. Reduced portal inflow causes liver dysfunction, fatty degeneration, and atrophy as a result of lack of nutrition, but liver function can be improved with restoration of portal blood flow.
Intrahepatic shunts generally close within the first 2 years of life, thus observation is sufficient in asymptomatic infants. With mild metabolic abnormalities, a protein-free diet supplemented with branched-chain amino acids, and lactulose is recommended. In all patients, shunt ratios greater than 60% require correction by embolization or surgical interruption (Gallego et al, 2004). If these interventions fail, hepatic transplantation is often necessary.
Gastroduodenal and Pancreatoduodenal Artery Aneurysms
Since 1895, when Ferguson first described a superior pancreatoduodenal artery (PDA) aneurysm, few studies have addressed the management and natural history of these lesions (Moore et al, 2004). True aneurysms of the PDA and the gastroduodenal artery (GDA) are rare and account for 2% and 1.5% of all splanchnic artery aneurysms, respectively (Shanley et al, 1996). Pseudoaneurysms are more common and are generally seen in the setting of inflammation, specifically pancreatitis (see Chapter 54). It is important to distinguish between true and false aneurysms, because the implications for diagnosis and management will be different.
A review of all reported PDA and GDA aneurysms between the years 1946 and 2001 identified approximately 146 cases (Moore et al, 2004). The mean age at presentation was 58 years (range, 20 to 72 years), with an equal distribution across ethnic groups and an equal male/female gender distribution; however, past studies have demonstrated a male predominance, which might be explained by a lack of distinction between true and false aneurysms (Moore et al, 2004; Shanley et al, 1996).
Approximately 50% of true PDA aneurysms are associated with celiac stenosis or occlusion (Moore et al, 2004), and aneurysms have also been described with common hepatic artery stenosis or occlusion (Bracale et al, 1996). Sutton first described the association of PDA aneurysms with celiac artery occlusion or stenosis in 1973 (Sutton & Lawton, 1973). Celiac artery entrapment by the median arcuate ligament can cause increased blood flow through collateral vessels and may be an etiologic factor (de Perrot et al, 1999b). Hyperdynamic flow and retrograde flow through the pancreatic collateral vessels leads to arterial hypertension and subsequent weakening of the arterial wall (Kalva et al, 2007). Other possible etiologies include degenerative disease of the arterial wall, fibromuscular dysplasia, Takayasu disease, and polyarteritis nodosa. In addition, the presence of a second aneurysm in a remote location—such as in the cerebral vasculature, found in 18% of GDA aneurysms and 24% of PDA aneurysms—suggests a systemic factor contributes to aneurysm formation (Moore et al, 2004). Medial arterial wall degeneration and atherosclerosis are present on pathologic examination.
The majority of patients with GDA or PDA aneurysms are symptomatic at the time of presentation. Epigastric pain with radiation to the back is common in the majority of symptomatic patients, and about 6% are seen initially for a palpable abdominal mass (Moore et al, 2004). Nonruptured aneurysms may also manifest with GI bleeding, occasional jaundice, and nonspecific GI symptoms (Shanley et al, 1996). Approximately 38% to 62% of PDA aneurysms and 35% to 56% of GDA aneurysms are brought to medical attention by rupture, which is associated with a mortality rate that ranges from 21% to 50% (Chiesa et al, 2005; Ikeda et al, 2008; Moore et al, 2004; Saltzberg et al, 2005; Sessa et al, 2004). About 20% of PDA aneurysms have the potential to rupture into the bowel and cause hematemesis, melena, and other signs of GI hemorrhage. Approximately 50% of GDA aneurysms will erode into the duodenum and cause GI bleeding. Aneurysms may also erode into adjacent vessels, organs, the bile duct (hemobilia; see Chapter 105), and the pancreatic duct (Ikeda et al, 2008). Aneurysms rupture into the intestinal tract and peritoneal cavity with equal frequency.
Ruptured aneurysms are treated with volume resuscitation, CT scan and angiography, and endovascular treatment or laparotomy, depending on the hemodynamic status of the patient. Nonruptured aneurysms should be treated aggressively, because they pose a high risk of subsequent rupture, and the risk is not necessarily related to the size of the aneurysm (Bageacu et al, 2006; Carr et al, 2001; Messina & Shanley, 1997; Moore et al, 2004; Takao et al, 2010).
In general, PDA aneurysms are more difficult to manage operatively than GDA aneurysms, because they are located deep within the parenchyma of the pancreas, and exposure may be difficult. Intraoperative duplex scanning or arteriography may aid in diagnosis and delineation of these aneurysms (Moore et al, 2004). Hemostasis may be difficult to obtain secondary to the extensive collateralization of the peripancreatic vessels and anatomic distortion from a retroperitoneal hematoma, and pancreatoduodenectomy has occasionally been required (de Perrot et al, 1999). Suture ligation of afferent and efferent vessels from within the aneurysm sac may be useful for aneurysms embedded in the pancreas. GDA aneurysms are slightly more accessible after duodenal mobilization, although adherence to bowel and surrounding structures can increase the difficulty of the operation.
Endovascular treatment has been increasingly utilized in the management of visceral aneurysms over the past decade. Peripancreatic aneurysms are often difficult to identify and isolate operatively, and catheter-based techniques are emerging as the treatment of choice. Various methods have been employed for embolization: coils, gelatin, foam, cyanoacrylate glue, ethanol sclerosant, and balloons (Coll et al, 1998). Recently, more selective embolization has become possible with the advent of smaller catheters and wire-based techniques that decrease risk of visceral ischemia (Moore et al, 2004). Selective embolization uses selective catheterization of the superior mesenteric artery followed by subselective catheterization of the branches feeding the aneurysm sac. Ideally, both the inflow and outflow of the aneurysm should be embolized to prevent reperfusion. If the aneurysm sac cannot be occluded for technical reasons, the parent artery can be occluded with coils (Kalva et al, 2007). Percutaneous embolization under CT guidance has recently been described (Williams et al, 2006).
Most studies advocate celiac revascularization, in addition to aneurysm ligation or embolization, when the celiac axis is stenotic or occluded (Bageacu et al, 2006; Moore et al, 2004). Celiac revascularization can be accomplished with either an open bypass or transluminal endovascular intervention. Revascularization may be performed through angioplasty and stenting in cases of atherosclerotic narrowing or by surgically dividing the median arcuate ligament in patients with median arcuate ligament syndrome (Tien et al, 2004). In addition, a bypass graft between the celiac axis or hepatic artery and superior mesenteric artery can be performed (Kalva et al, 2007). In nonruptured true aneurysms, it may be safer to restore celiac axis patency prior to aneurysm occlusion (de Perrot et al, 1999; Moore et al, 2004). Mesenteric ischemia and death following aneurysm occlusion in the absence of a patent celiac axis has been reported.
Pseudoaneurysms
Most pseudoaneurysms that affect the peripancreatic and pancreatic vasculature are complications of pancreatitis (Fig. 104.6; see Chapter 54), but these may also result from treatment of pancreatic tumors (see Chapter 62A, Chapter 62B, Chapter 63A, Chapter 63B ). Pseudoaneurysms complicate 3% to 10% of acute pancreatitis cases and affect 10% to 17% of patients with chronic pancreatitis (de Perrot et al, 1999; Mallick & Winslet, 2004; Woods et al, 1995). The temporal relationship of pseudoaneurysm formation to pancreatitis is highly variable, and it is complicated by the fact that the presenting features of abdominal pain—tachycardia and hypotension—are also features of pancreatitis. A pseudoaneursym associated with chronic pancreatitis is often identified at the time of an acute exacerbation, and it remains unclear whether the pseudoaneurysm caused the exacerbation or was the result of a new episode of inflammation.
The likelihood of pancreatic pseudocyst appears to increase with the severity of the inflammation in acute pancreatitis (Flati et al, 2003). An expanding pseuodocyst may erode into an adjacent vessel and form a pseudoaneurysmal pseudocyst as a result of constant compression, ischemia, and elastolytic action (Flati et al, 2003; Shanley et al, 1996). Additionally, severe inflammation, infection, release of pancreatic enzymes, and necrosis may cause pseudoaneurysm formation and vessel erosion, which can precipitate subsequent rupture with resultant massive bleeding into the GI tract, retroperitoneum, and peritoneal cavity (Flati et al, 2003; Mallick & Winslet, 2004; Woods et al, 1995). Manipulation of friable tissue during necrosectomy may precipitate vascular damage as well. Periarterial inflammation, vascular necrosis, trauma, infection, vascular intervention, biliary intervention, and autoimmune disorders have also been identified as causative agents of pseudoaneurysms.
The splenic artery is the most frequently involved (40%), followed by the GDA (30%) (Mallick & Winslet, 2004). The size of the pseudoaneurysm is not predictive of rupture, which may occur into the abdomen, retroperitoneum, adjacent hollow organ, or blood vessel. The mortality associated with a ruptured pseudoaneurysm ranges from 7.5% to 80% (Burli et al, 2008; Flati et al, 2003), and symptoms include exacerbation of abdominal pain, hypotension, tachycardia, hematemesis, melena, or bleeding from surgical drains in patients who have recently undergone a pancreatic procedure.
The management of ruptured pseudoaneurysms of the peripancreatic vasculature and GDA is similar to that described for true aneurysms with resuscitation, CT scan, angiography, endovascular therapy, or open surgical therapy (Fig. 104.7). Immediate surgery is indicated if the patient is hemodynamically unstable. Conservative management is associated with a higher overall mortality rate (21% to 54%) than intervention (18% to 34%), therefore definitive treatment is recommended (Flati et al, 2003; Moore et al, 2004). Patients with angiographic evidence of bleeding can be treated with endovascular embolization or percutaneous thrombin injection into the aneurysm sac. Because of the extensive collateral circulation, it is imperative to occlude the artery proximal and distal to the lesion and to interrupt any collateral supply (Burli et al, 2008).
Pancreatic Arteriovenous Malformations
AVM of the pancreas is a rare anomaly, first reported by Halpern in 1968 and described as a vascular mass lesion that arises via an aberrant bypass anastomosis of the arterial and venous systems in the pancreas (Halpern et al, 1968a, 1968b). Congenital pancreatic AVMs are more common (approximately 90%) and are likely a remnant of the fetal vascular network. One third of these cases are associated with Osler-Weber-Rendu syndrome (Chang et al, 2004), and acquired malformations are generally the result of trauma, tumor, infection, or inflammation, especially pancreatitis. Pancreatic AVMs have been reported in pancreatic allografts following transplantation (Barth et al, 2008).
AVMs typically come to medical attention in the fifth decade of life, but ages at presentation range from infancy to the seventh decade. Some studies note a male predominance (Takemoto et al, 2007). The most commonly involved vessels are the splenic artery (42%), GDA (22%), and small pancreatic arteries (22%; Rezende et al, 2003). Most patients are diagnosed based on presentation with complicating symptoms such as GI bleeding, portal hypertension, or pancreatitis. GI bleeding is present in 50% of patients and may be caused by rupture into the pancreatic duct, rupture of superficial vessels into the duodenum, duodenal erosion, hemobilia as a result of erosion into the bile duct (see Chapter 105), or bleeding AVMs at other sites within the GI tract (Aida et al, 2002). Pancreatic AVMs can cause portal hypertension, leading to esophageal varices, GI bleeding, and other related sequelae. Early diagnosis and intervention is warranted to prevent complications.
Angiography demonstrates an abnormal communication between the arterial and venous system with dilated feeding vessels, transient pancreatic staining, and early filling of the portal vein. Doppler ultrasound shows a mosaic pattern and abnormal blood flow and a pulsatile waveform in the portal vein (Koito et al, 2001). CT scan features include conglomeration of strong nodular stains and early enhancement of portal venous system in the arterial phase (Ogawa et al, 2009). MRI shows a circular lesion with signal void in T1- and T2-weighted images, consistent with rapid blood flow (Ogawa et al, 2009).
Angiographic embolization is the first-line treatment for pancreatic AVMs. The recurrence rate is 30% to 40% secondary to the development of multiple collateral vessels (Rezende et al, 2003). For large lesions confined to the head of the pancreas, surgical resection by pancreatoduodenectomy and total pancreatectomy has been performed. Some studies advocate embolization prior to operative intervention to potentially decrease intraoperative bleeding and postoperative morbidity and mortality (Iwashita et al, 2002; Rezende et al, 2003). Lesions localized to the tail of the pancreas can be treated by distal pancreatectomy.
Abbas MA, et al. Hepatic artery aneurysm: factors that predict complications. J Vasc Surg. 2003;38:41-45.
Aida K, et al. Duodenal ulcer and pancreatitis associated with pancreatic arteriovenous malformation. Eur J Gastroenterol Hepatol. 2002;14:551-554.
Altuntas B, et al. Severe portal hypertension due to congenital hepatoportal arteriovenous fistula associated with intrahepatic portal vein aneurysm. J Clin Ultrasound. 1998;26:357-360.
Asensio JA, et al. Operative management and outcome of 302 abdominal vascular injuries. Am J Surg. 2000;180:528-533.
Asensio JA, et al. Superior mesenteric venous injuries: to ligate or to repair remains the question. J Trauma. 2007;62:668-675.
Bageacu S, et al. True aneurysms of the pancreaticoduodenal artery: successful non-operative management. Surgery. 2006;139:608-616.
Barth MM, et al. Transarterial and transvenous embolotherapy of arteriovenous fistulas in the transplanted pancreas. J Vasc Interv Radiol. 2008;19:1231-1235.
Blumgart LH, Fong Y. Surgery of The Liver and Biliary Tract, 3rd ed. New York: WB Saunders; 2000.
Boon LM, et al. Hepatic vascular anomalies in infancy: a twenty-seven-year experience. J Pediatr. 1996;129:346-354.
Bracale G, et al. Inferior pancreaticoduodenal artery aneurysm associated with common hepatic artery occlusion. J Cardiovasc Surg (Torino). 1996;37:579-581.
Briceno J, et al. Late hepatic artery pseudoaneurysm: a rare complication after resection of hilar cholangiocarcinoma. World J Gastroenterol. 2008;14:5920-5923.
Buckman RF, et al. Portal vein injuries. Surg Clin North Am. 2001;81:1449-1462.
Bulut T, et al. False aneurysm of the hepatic artery after laparoscopic cholecystectomy. Acta Chir Belg. 2002;102:459-463.
Burli P, et al. Imaging appearances and endovascular management of uncommon pseudoaneurysms. Clin Radiol. 2008;63:1254-1264.
Burrows PE, Dubois J, Kassarjian A. Pediatric hepatic vascular anomalies. Pediatr Radiol. 2001;31:533-545.
Carr SC, et al. Current management of visceral artery aneurysms. Surgery. 1996;120:627-633.
Carr SC, et al. Visceral artery aneurysm rupture. J Vasc Surg. 2001;33:806-811.
Chang S, et al. Arteriovenous malformation of the pancreas in a patient with gastrointestinal bleeding: helical CT findings. Abdom Imaging. 2004;29:259-262.
Chiesa R, et al. Visceral artery aneurysms. Ann Vasc Surg. 2005;19:42-48.
Choi BI, et al. Hepatic arterioportal shunts: dynamic CT and MR features. Korean J Radiol. 2002;3:1-15.
Christison-Lagay ER, et al. Hepatic hemangiomas: subtype classification and development of a clinical practice algorithm and registry. J Pediatr Surg. 2007;42:62-67.
Coimbra R, et al. Outcome from traumatic injury of the portal and superior mesenteric veins. Vasc Endovascular Surg. 2004;38:249-255.
Coll DP, et al. Aneurysms of the pancreaticoduodenal arteries: a change in management. Ann Vasc Surg. 1998;12:286-291.
David RJ, et al. Evolution in the management of hepatic trauma: a 25-year perspective. Ann Surg. 2000;232:324-330.
Deb G, et al. Hemangioendothelioma: successful therapy with interferon-alpha: a study in association with the Italian Pediatric Haematology/Oncology Society (AIEOP). Med Pediatr Oncol. 2002;38:118-119.
de Perrot M, et al. Management of bleeding pseudoaneurysms in patients with pancreatitis. Br J Surg. 1999;86:29-32.
de Perrot M, et al. Management of true aneurysms of the pancreaticoduodenal arteries. Ann Surg. 1999;229:416-420.
Dickie B, et al. Spectrum of hepatic hemangiomas: management and outcome. J Pediatr Surg. 2009;44:125-133.
Dolapci M, Ersoz S, Kama NA. Hepatic artery aneurysm. Ann Vasc Surg. 2003;17:214-216.
Duce MN, et al. Spontaneous thrombosis of a post-traumatic hepatic artery pseudoaneurysm with arterio-biliary fistula in a child: helical CT diagnosis. Pediatr Radiol. 2002;32:95.
Flati G, et al. Potentially fatal bleeding in acute pancreatitis: pathophysiology, prevention, and treatment. Pancreas. 2003;26:8-14.
Fraga GP, et al. A 20-year experience with portal and superior mesenteric venous injuries: has anything changed? Eur J Vasc Endovasc Surg. 2009;37:87-91.
Gallego C, et al. Congenital and acquired anomalies of the portal venous system. Radiographics. 2002;22:141-159.
Gallego C, et al. Congenital hepatic shunts. Radiographics. 2004;24:755-772.
Gitzelmann R, Forster I, Willi UV. Hypergalactosaemia in a newborn: self-limiting intrahepatic portosystemic venous shunt. Eur J Pediatr. 1997;156:719-722.
Grego FG, et al. Visceral artery aneurysms: a single center experience. Cardiovasc Surg. 2003;11:19-25.
Guida PM, Moore SW. Aneurysm of the hepatic artery: report of five cases with a brief review of the previously reported cases. Surgery. 1966;60:299-310.
Halpern M, Turner AF, Citron BP. Angiodysplasias of the abdominal viscera associated with hereditary hemorrhagic telangiectasia. Am J Roentgenol Radium Ther Nucl Med. 1968;102:783-789.
Halpern M, Turner AF, Citron BP. Hereditary hemorrhagic telangiectasia: an angiographic study of abdominal visceral angiodysplasias associated with gastrointestinal hemorrhage. Radiology. 1968;90:1143-1149.
Ho J, et al. New insight into the pathophysiology of severe hypothyroidism in an infant with multiple hepatic hemangiomas. J Pediatr Endocrinol Metab. 2005;18:511-514.
Hossain A, et al. Visceral artery aneurysms: experience in a tertiary-care center. Am Surg. 2001;67:432-437.
Huang SA, et al. Severe hypothyroidism caused by type 3 iodothyronine deiodinase in infantile hemangiomas. N Engl J Med. 2000;343:185-189.
Ikeda O, et al. Nonoperative management of unruptured visceral artery aneurysms: treatment by transcatheter coil embolization. J Vasc Surg. 2008;47:1212-1219.
Ingram JD, et al. Hepatoblastoma in a neonate: a hypervascular presentation mimicking hemangioendothelioma. Pediatr Radiol. 2000;30:794-797.
Isaacs HJr. Fetal and neonatal hepatic tumors. J Pediatr Surg. 2007;42:1797-1803.
Iwashita Y, et al. Pancreatic arteriovenous malformation treated by transcatheter embolization. Hepatogastroenterology. 2002;49:1722-1723.
Kalva SP, et al. Inferior pancreaticoduodenal artery aneurysms in association with celiac axis stenosis or occlusion. Eur J Vasc Endovasc Surg. 2007;33:670-675.
Kasirajan K, et al. Endovascular management of visceral artery aneurysm. J Endovasc Ther. 2001;8:150-155.
Koito K, et al. Congenital arteriovenous malformation of the pancreas: its diagnostic features on images. Pancreas. 2001;22:267-273.
Konrad D, Ellis G, Perlman K. Spontaneous regression of severe acquired infantile hypothyroidism associated with multiple liver hemangiomas. Pediatrics. 2003;112:1424-1426.
Lagana D, et al. Multimodal approach to endovascular treatment of visceral artery aneurysms and pseudoaneurysms. Eur J Radiol. 2006;59:104-111.
Lane MJ, Jeffrey RBJr, Katz DS. Spontaneous intrahepatic vascular shunts. AJR Am J Roentgenol. 2000;174:125-131.
Larson RA, Solomon J, Carpenter JP. Stent graft repair of visceral artery aneurysms. J Vasc Surg. 2002;36:1260-1263.
Lima M, et al. Congenital symptomatic intrahepatic arteriovenous fistulas in newborns: management of 2 cases with prenatal diagnosis. J Pediatr Surg. 2005;40:e1-e5.
Luks FI, et al. Benign liver tumors in children: a 25-year experience. J Pediatr Surg. 1991;26:1326-1330.
Mallick IH, Winslet MC. Vascular complications of pancreatitis. JOP. 2004;5:328-337.
Marrone G, et al. Percutaneous transarterial embolization of extrahepatic arteroportal fistula. World J Gastroenterol. 2006;12:5562-5564.
Messina LM, Shanley CJ. Visceral artery aneurysms. Surg Clin North Am. 1997;77:425-442.
Moore E, et al. Surgical management of peripancreatic arterial aneurysms. J Vasc Surg. 2004;40:247-253.
O’Connor PJ, et al. The radiological treatment of hepatic artery aneurysms. Clin Radiol. 1995;50:792-796.
O’Driscoll D, Olliff SP, Olliff JF. Hepatic artery aneurysm. Br J Radiol. 1999;72:1018-1025.
Ogawa H, et al. Arteriovenous malformation of the pancreas: assessment of clinical and multislice CT features. Abdom Imaging. 2009;34:743-752.
Panayiotopoulos YP, Assadourian R, Taylor PR. Aneurysms of the visceral and renal arteries. Ann R Coll Surg Engl. 1996;78:412-419.
Park JH, et al. Intrahepatic portosystemic venous shunt. AJR Am J Roentgenol. 1990;155:527-528.
Pearl J, et al. Traumatic injuries to the portal vein: case study. J Trauma. 2004;56:779-782.
Pringle JH. V. Notes on the arrest of hepatic hemorrhage due to trauma. Ann Surg. 1908;48:541-549.
Prokurat A, et al. Hemangioma of the liver in children: proliferating vascular tumor or congenital vascular malformation? Med Pediatr Oncol. 2002;39:524-529.
Rezende MB, et al. Pancreatic arteriovenous malformation. Dig Surg. 2003;20:65-69.
Rokke O, et al. Successful management of eleven splanchnic artery aneurysms. Eur J Surg. 1997;163:411-417.
Sachdev U, et al. Management of aneurysms involving branches of the celiac and superior mesenteric arteries: a comparison of surgical and endovascular therapy. J Vasc Surg. 2006;44:718-724.
Saltzberg SS, et al. Is endovascular therapy the preferred treatment for all visceral artery aneurysms? Ann Vasc Surg. 2005;19:507-515.
Sessa C, et al. Treatment of visceral artery aneurysms: description of a retrospective series of 42 aneurysms in 34 patients. Ann Vasc Surg. 2004;18:695-703.
Shanley CJ, Shah NL, Messina LM. Common splanchnic artery aneurysms: splenic, hepatic, and celiac. Ann Vasc Surg. 1996;10:315-322.
Shanley CJ, Shah NL, Messina LM. Uncommon splanchnic artery aneurysms: pancreaticoduodenal, gastroduodenal, superior mesenteric, inferior mesenteric, and colic. Ann Vasc Surg. 1996;10:506-515.
Stocker JT. Hepatic tumors in children. Clin Liver Dis. 2001;5:259-281. viii-ix
Stone HH, Fabian TC, Turkleson ML. Wounds of the portal venous system. World J Surg. 1982;6:335-341.
Sutton D, Lawton G. Coeliac stenosis or occlusion with aneurysm of the collateral supply. Clin Radiol. 1973;24:49-53.
Takao H, Nojo T, Ohtomo K. True pancreaticoduodenal artery aneurysms: a decision analysis. Eur J Radiol. 2010;75:110-113.
Takemoto I, et al. Pancreatic arteriovenous malformation combined with portal thrombosis. Intern Med. 2007;46:233-236.
Tessier DJ, et al. Iatrogenic hepatic artery pseudoaneurysms: an uncommon complication after hepatic, biliary, and pancreatic procedures. Ann Vasc Surg. 2003;17:663-669.
Tien YW, Kao HL, Wang HP. Celiac artery stenting: a new strategy for patients with pancreaticoduodenal artery aneurysm associated with stenosis of the celiac artery. J Gastroenterol. 2004;39:81-85.
Tomczak R, et al. Abdominal arteriovenous and arterio-portal fistulas: etiology, diagnosis, therapeutic possibilities [in German]. Z Gastroenterol. 1997;35:555-562.
Tulsyan N, et al. The endovascular management of visceral artery aneurysms and pseudoaneurysms. J Vasc Surg. 2007;45:276-283.
von Schweinitz D. Neonatal liver tumours. Semin Neonatol. 2003;8:403-410.
Williams M, et al. CT-guided percutaneous thrombin injection for treatment of an inferior pancreaticoduodenal artery pseudoaneurysm. Cardiovasc Intervent Radiol. 2006;29:669-671.
Woods MS, et al. Successful treatment of bleeding pseudoaneurysms of chronic pancreatitis. Pancreas. 1995;10:22-30.