CHAPTER 27 Anesthesia for Ophthalmic Surgery
Anesthesia for ophthalmologic procedures in children requires an understanding of several physiologic and pharmacologic concepts that are unique to this population. The majority of ophthalmic procedures are brief and noninvasive, but the spectrum extends to more invasive procedures in patients with significant comorbid disease. Caring for otherwise healthy children undergoing nasolacrimal duct probing or strabismus surgery may be relatively straightforward, but the pediatric anesthesiologist is also required to care for vulnerable infants born prematurely or with congenital disorders and associated pathology of the eye. In 2005 there were 2.2 million procedures performed on patients younger than 15 years of age; 12,000 of these were operations on the eye (Defrances and Hall, 2007). Most of these procedures are performed in the ambulatory setting. In an analysis of ophthalmic procedures performed in surgery centers, the reasons for cancellation of surgery within 24 hours were explored. The pediatric group (0 to 9 years of age) had the highest rate of cancellation when compared with those in other age groups. The majority of cancellations, however, were because of unpreventable causes such as patient illness (Henderson et al., 2005). The information contained in this chapter will give anesthesiologists the information needed to plan for and safely perform anesthesia for ophthalmic procedures.
Anesthesiologists with a particular interest in ophthalmologic anesthesia can find valuable resources through the Ophthalmic Anesthesia Society (http://www.eyeanesthesia.org) and the British Ophthalmic Anaesthesia Society (http://www.boas.org). A glossary of terms is given in Box 27-1.
Box 27-1 Glossary of Ophthalmologic Terms
From Dorland’s illustrated medical dictionary, Philadelphia, 2000, Saunders.
Anatomy and Physiology
Knowledge of the anatomy and physiology of the eye is paramount to understanding the array of ophthalmic procedures performed, the influence that anesthesia may have on normal and abnormal ocular physiology, and the systemic effects that surgical manipulation of the eye may have on the patient (McGoldrick, 1992a).
Anatomy Overview
The orbit is formed by a complex arrangement of seven cranial bones: frontal, zygomatic, sphenoid, maxilla, palatine, lacrimal, and ethmoid (Fig. 27-1). The optic foramen transmits the optic nerve, the ophthalmic artery and vein, and the sympathetic contributions from the carotid plexus. The superior orbital fissure transmits branches from four other cranial nerves (oculomotor, trigeminal, trochlear, and abducens) and the superior and inferior ophthalmic veins. The infraorbital fissure (representing the weakest aspect of the orbit) transmits the infraorbital and zygomatic nerves. The infraorbital foramen (located below the orbital rim) transmits the infraorbital nerve, artery, and vein.
The aqueous humor occupies the anterior and posterior chambers of the eye and is responsible for providing nutrients to the avascular lens and the endothelial aspect of the cornea (Fig. 27-2). The volume of aqueous humor (0.3 mL in the adult) is primarily responsible for intraocular pressure (IOP) regulation. The vitreous humor, created embryologically between 1 and 4 months’ gestation, is a hydrophilic gel that accounts for 80% of the volume of the globe. The vitrous humor is 99% water, although in the presence of hyaluronic acid (a mucopolysaccharide), its viscosity is twice that of water. The volume of the vitreous humor is more constant than that of the aqueous humor, although it may be slightly influenced by hydration status and osmotically active medications.
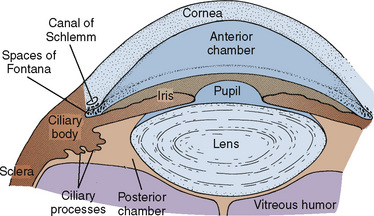
FIGURE 27-2 Anatomy of the anterior eye.
(From McGoldrick KE: Anesthesia for ophthalmic surgery. In Motoyama ES, Davis PJ, editors: Smith’s anesthesia for infants and children, ed 6, Philadelphia, 1996, Mosby.)
The optic nerve (cranial nerve II) is the nerve of vision and may be thought of as a diverticulum of the forebrain. The oculomotor nerve (cranial nerve III) provides motor innervation to four of the six extraocular muscles and the levator palpebrae superioris, as well as parasympathetic innervation to the pupillary sphincter (miosis) and ciliary muscles (accommodation). The two other extraocular muscles are innervated by the trochlear and abducens nerves. The ophthalmic division of the trigeminal nerve (cranial nerve V) transmits all of the nonvisual sensory innervation from the eye and orbit and provides sympathetic innervation to the pupillary dilators (mydriasis). The temporal and zygomatic branches of the facial nerve (cranial nerve VII) innervate the orbicularis oculi (Ellis and Feldman, 1997).
Blood is supplied to the eye and orbit through branches of the internal and external carotid arteries. The first branch of the intracranial carotid artery, the ophthalmic artery, divides into the central retinal artery, as well as the long and short posterior ciliary arteries, to nourish the retina. The long and short posterior ciliary arteries converge to supply the choriocapillaris, the capillary layer within the choroid that supplies 60% to 80% of the oxygen to the retina. The anterior portion of the optic nerve is perfused by the posterior ciliary arteries. It is this network of arteries that is subject to significant individual variation, predisposing some patients to anterior ischemic optic neuropathy after periods of hypotension. The posterior optic nerve is perfused by pial vessels branching from the ophthalmic artery. Superior and inferior ophthalmic veins drain the orbit, and the central retinal vein provides ocular drainage. All venous drainage is subsequently transmitted to the cavernous sinus (Williams, 2002).
Physiology Overview
Normal IOP varies between 10 and 20 mm Hg and may differ by as much as 5 mm Hg between the two eyes. Normal pressures are somewhat lower in the newborn (average, 9.5 mm Hg) but approximate adult pressures by 5 years of age (Pensiero et al., 1992). A pressure above 25 mm Hg at any age is considered abnormal (Johnson and Forrest, 1994). Transient changes in IOP are well tolerated in the intact eye, although chronic elevations may be detrimental to normal retinal perfusion and vision.
With mydriasis, Fontana’s spaces narrow, resistance to outflow is increased, and IOP rises. Mydriasis is a threat in both closed-angle and open-angle glaucoma.* Hence, a miotic agent such as pilocarpine hydrochloride is often efficacious when applied conjunctivally before surgery in patients with glaucoma.
The arterial circulation of the eye is autoregulated. Only marked deviations in systemic arterial pressures affect IOP. Elevated venous pressures, on the other hand, can dramatically increase IOP, primarily by augmenting the choroidal blood volume and tension of the orbit. Coughing, vomiting, and Valsalva maneuvers may increase IOP to 40 mm Hg or more. Respiratory acidosis increases IOP, whereas metabolic acidosis has the opposite effect. Conversely, respiratory alkalosis decreases IOP, whereas metabolic alkalosis increases IOP (Calobrisi and Lebowitz, 1990). Hypoxia is capable of increasing IOP by dilating intraocular vessels, whereas hyperoxia appears to decrease IOP (Johnson and Forrest, 1994).
The OCR, through its vagal efferent pathway, may manifest as sinus bradycardia, ectopy, and sinus arrest. Death secondary to the OCR in otherwise healthy children has been described (Lang and Van der Wal, 1994; Smith, 1994). A more thorough description of this reflex, prophylaxis, and therapy are provided later in the discussion on intraoperative complications.
The ORR has also been recognized for nearly 100 years but is less often appreciated with the use of controlled ventilation. Through a postulated connection between the trigeminal nerve, the pneumotaxic center of the pons, and the medullary respiratory centers, pressure on the extraocular muscles may result in tachypnea or respiratory arrest (Johnson and Forrest, 1994). This reflex is not inhibited by the use of atropine or glycopyrrolate. A review of the ORR and its potential for causing hypercapnia and hypoxia (potentially aggravating the OCR) has heightened awareness of the reflex and led some investigators to recommend controlled ventilation during strabismus surgery (Blanc et al., 1988).
The OER is admittedly more theoretic than the other two reflexes but would explain the high incidence of nausea and emesis after strabismus surgery. An association between the OCR and the OER has been demonstrated such that patients who exhibit the OCR intraoperatively are 2.6 times more likely to experience postoperative vomiting than those without OCR manifestations (Allen et al., 1998). Anticholinergic therapy does not decrease the incidence of postoperative nausea and vomiting (PONV). Appropriate prophylaxis and treatment of PONV have been studied extensively and are thoroughly reviewed later in this chapter.
General Considerations In Caring for the Ophthalmologic Patient
Associated Congenital and Metabolic Conditions
Ophthalmologic disorders may be inherited as isolated defects in autosomal recessive, autosomal dominant, and X-linked recessive fashion. An additional large number of metabolic defects, congenital syndromes, and chromosomal abnormalities are also associated with ocular pathology. The anesthesiologist caring for the ophthalmologic patient must be aware of these associations. An overview of the commonly encountered syndromes and disorders along with their ocular manifestations and potential anesthetic implications is provided in Table 27-1 (McGoldrick, 1992d; Butera et al., 2000; Baum and O’Flaherty, 2006).
TABLE 27-1 Congenital Syndromes and Chromosomal Abnormalities with Associated Ocular Manifestations
Disorder or Syndrome | Ocular Manifestations | Anesthetic Implications |
Acute intermittent porphyria | Cataracts, retinal degeneration, optic atrophy | Various medications, including barbiturates and etomidate, may trigger attacks. |
Apert’s syndrome | Glaucoma, cataracts, strabismus, hypertelorism, proptosis | Possibly difficult intubation, possible choanal stenosis, cervical spine fusion, CHD (10% incidence) |
Cri du chat syndrome | Strabismus | Micrognathia and possibly difficult intubation, hypotonia, prone to hypothermia, CHD (33% incidence) |
Crouzon’s disease | Glaucoma, cataracts, strabismus, hypertelorism, proptosis | Possibly difficult intubation, possible elevated intracranial pressure |
Cystinosis | Corneal clouding, retinal degeneration | Chronic renal failure, possible diabetes mellitus, esophageal varices, recurrent epistaxis, hyperthermia |
Down syndrome | Cataracts, strabismus | Trisomy 21, airway obstruction, atlantoaxial instability, CHD (50% incidence), may be more sensitive to atropine |
Ehlers-Danlos syndrome | Retinal detachment, blue sclera, ectopia lentis, keratoconus | Laryngeal trauma possible with intubation, careful positioning, avoid arterial and central venous lines |
Goldenhar’s syndrome | Glaucoma, cataracts, strabismus, lacrimal drainage defects | Hemifacial microsomia and possible cervical spine abnormalities, possible difficult mask and intubation, rare CHD, and hydrocephalus |
Hallerman-Streiff syndrome | Congenital cataracts, coloboma, microphthalmia, glaucoma | Major craniofacial abnormalities with likely difficult intubation, upper airway obstruction, chronic lung disease |
Homocystinuria | Ectopia lentis, pupillary block glaucoma, retinal detachment, central retinal artery occlusion, strabismus optic atrophy, | Marfanoid habitus with kyphoscoliosis and sternal deformity, prone to thromboembolic complications and hypoglycemia |
Hunter’s syndrome | Retinal degeneration, optic atrophy | Often difficult intubation, copious secretions, macroglossia, stiff temporomandibular joint, limited neck mobility, possible ischemic or valvular heart disease |
Hurler’s syndrome | Corneal clouding, retinal degeneration, optic atrophy | Often difficult intubation and difficult mask, possible cervical spine instability, possible ischemic or valvular heart disease |
Jeune syndrome | Retinal degeneration | Limited thoracic excursion, pulmonary hypoplasia, possible renal and hepatic insufficiency |
Lowe’s syndrome | Cataracts, glaucoma (hydrophthalmia) | Renal failure, renal tubular acidosis |
Marfan syndrome | Ectopia lentis, glaucoma, retinal detachment, cataracts, strabismus | Aortic or pulmonary artery dilation, aortic and mitral valve disease, pectus excavatum, risk for pneumothorax |
Moebius sequence | Strabismus, ptosis, congenital nerve VI and VII palsy | Possibly difficult intubation, micrognathia, copious secretions, possible cervical spine anomalies |
Myotonia congenita | Cataracts, blepharospasm | Prone to myotonic contractions, sustained contraction with succinylcholine |
Myotonic dystrophy | Cataracts, ptosis, strabismus | Prone to myotonic contractions, succinylcholine-associated contractions and hyperkalemia, cardiac conduction abnormalities, sensitive to central nervous system depressants |
Rubella syndrome | Cataracts, microphthalmos, glaucoma, optic atrophy | Neonatal pneumonia, anemia, and thrombocytopenia; CHD, hypopituitarism, diabetes mellitus |
Sickle cell disease | Retinal detachment, vitreous hemorrhage, retinitis proliferans | Tendency for sickling occurs with high hemoglobin S concentrations, hypoxemia, cold, stasis, dehydration, and infection |
Smith-Lemli-Opitz syndrome | Congenital cataracts | Possibly difficult intubation, micrognathia, pulmonary hypoplasia, CHD, gastroesophageal reflux, seizure disorders |
Stickler syndrome | Vitreous degeneration, retinal detachments, cataracts, strabismus | Possibly difficult intubation, micrognathia, mitral valve prolapse, marfanoid habitus, scoliosis, kyphosis |
Sturge-Weber syndrome | Choroidal hemangioma, glaucoma, ectopia lentis | Angiomas of the airway, CHD and high output failure, seizure disorders, hyperkalemic response to succinylcholine in those with hemiplegia |
Treacher Collins syndrome | Lid defects, microphthalmia | Often difficult intubation, mandibular hypoplasia, CHD |
Turner’s syndrome | Ptosis, strabismus, cataracts, corneal scars, blue sclera | Possibly difficult intubation and intravenous access, CHD |
von Hippel–Lindau syndrome | Retinal hemangioma | Possible increased intracranial pressure, possible pheochromocytoma, cerebellar tumors may also produce episodic hypertension |
von Recklinghausen’s disease | Ptosis, proptosis, optic glioma and meningioma, optic atrophy, glaucoma, Lisch nodules | Possibly difficult mask ventilation and intubation, possible airway tumors, restrictive lung disease, renovascular hypertension, possible pheochromocytoma, sensitive to neuromuscular blockers |
Zellweger syndrome | Glaucoma, cataracts, optic atrophy, optic nerve hypoplasia | Micrognathia, possible CHD, renal and adrenal insufficiency |
CHD, Congenital heart disease.
Ophthalmologic Medications and Their Systemic Effects
There are a variety of medications used by pediatric ophthalmologists in the outpatient and perioperative settings that may have important anesthetic ramifications. As with all medications, the ophthalmic agents have both desirable and undesirable effects that may be more pronounced and ominous in the pediatric patient by virtue of greater systemic absorption or higher dosing relative to body weight and pharmacologic compartment. The anesthesiologist must be familiar with every medication used in the perioperative period and pay particular attention to the total dose administered and potential for deleterious effects. An overview of the ophthalmic medications is provided (Table 27-2).
Topical ophthalmologic agents have greater use than systemic agents in the pediatric and adult populations, primarily because most of the side effects that would be consequential to systemic administration are diminished. Nevertheless, the excess from ocular application invariably enters the lacrimal system, reaching the nasopharyngeal mucosa where systemic absorption is greatly enhanced compared with that at the conjunctival sac. Whereas a single drop from a commercial eye dropper may have a volume ranging between 50 and 75 μL, maximal ocular bioavailability is reached by instillation of only 20 μL (McGoldrick, 1992b). It has been recommended that digital pressure over the lacrimal duct for 5 minutes after instillation may reduce systemic absorption by 67% (Zimmerman et al., 1984). Keeping the eye gently closed for 5 minutes may afford similar benefit, yet both techniques are understandably difficult in the conscious and fretful child.
Cycloplegic and Mydriatic Agents
Cyclopentolate, a commonly used cycloplegic agent, has a peak effect within 20 to 45 minutes and residual effects that persist for as long as 36 hours (Cooper et al., 2000). Mild gastrointestinal discomfort and feeding intolerance are the most commonly encountered side effects, although more severe atropine-like toxicity with symptoms ranging from vomiting, ileus, hyperthermia, delirium, and grand mal seizures (Kennerdell and Wucher, 1972; Bauer et al., 1973) has also been reported.
Atropine and homatropine are extremely potent anti-accommodative agents that are rarely used for pediatric patients in the perioperative setting. These agents are more commonly used for intraocular inflammation and amblyopia therapy; they may also be used for prolonged mydriasis after cataract extraction to prevent the formation of synechiae. Common side effects include thirst, tachycardia, and hyperthermia, although more severe symptoms may result after overzealous administration (McGoldrick, 1992b).
Ophthalmic phenylephrine (available in 2.5% and 10% concentrations) is commonly used for mydriasis and vasoconstriction during various procedures. Maximal effects are usually observed within 15 minutes, and residual effects may persist for 4 hours after administration. The generally accepted dosing limit for pediatric patients is one drop of the 2.5% solution in each eye per hour (Borromeo-McGrail et al., 1973). One drop (50 µL) of the 2.5% solution contains approximately 1.25 mg of phenylephrine. The potential for severe hypertension, pulmonary edema, cardiac arrhythmia, cardiac arrest, and subarachnoid hemorrhage with topical phenylephrine is well appreciated by surgeons and anesthesiologists alike. With careful application of the 2.5% solution, systemic effects are typically mild, well tolerated, and generally observed within 1 to 20 minutes after application (Fraunfelder et al., 2002). Although one study demonstrated no significant difference in the mydriatic effects of cyclopentolate vs. phenylephrine (both administered in combination with tropicamide), many ophthalmologists still rely on the medication either primarily or when additional dilation is needed after the administration of other preparations (Rosales et al., 1981).
Glaucoma Pharmacologic Therapy
Pilocarpine is a parasympathomimetic agent that produces miosis and a fall in IOP that is thought to result from an increase in aqueous humor outflow. It is rarely used for temporary treatment before surgery in children but should be discontinued on the evening before surgery for adequate assessment of pressure (Khaw et al., 2000). At recommended dosages, side effects are thought to be rare but may include gastrointestinal disturbances and diaphoresis. More severe cardiovascular effects (e.g., hypotension, bradycardia, and atrioventricular block) are occasionally observed in the geriatric patient (Everitt and Avorn, 1990).
Topical epinephrine and its prodrug, dipivefrin, are sympathomimetic agents historically used in the treatment of glaucoma. Topical epinephrine is occasionally used by ophthalmologists in the intraoperative setting and is known to potentiate dysrhythmias in the myocardium sensitized by the volatile agents. Of all the potent inhalation agents, halothane clearly has the greatest dysrhythmogenic potential, although one study has demonstrated that the pediatric heart may be more resistant to the interactions between halothane and exogenous epinephrine (Karl et al., 1983; Ueda et al., 1983). At equipotent concentrations, isoflurane has three times less dysrhythmogenic potential than halothane (Marshall and Longnecker, 2001). Desflurane and sevoflurane are thought to be similar to isoflurane in this regard (Moore et al., 1993; Navarro et al., 1994).
The β-blocking agents timolol, levobunolol, and betaxolol act by decreasing the production of aqueous humor and are occasionally used postoperatively in children. The agents should not be used in the neonatal and infant populations in light of several reports of apnea with the use of timolol (Olson et al., 1979; Bailey, 1984). In older children and adults, the use of betaxolol, which is selective for the β1-receptors, is associated with fewer complications involving the pulmonary system, although dyspnea and bronchospasm have been reported (Everitt and Avorn, 1990). Lethargy, bradycardia, and heart block are possible with all of the topical β-blocking agents (Gross and Pineyro, 1997).
Apraclonidine and brimonidine are topical α2-agonists that decrease sympathetic tone and subsequently reduce aqueous humor production. Brimonidine, unlike apraclonidine, is capable of crossing the blood-brain barrier and should be used with great caution in young children. Bradycardia, hypotension, hypothermia, hypotonia, and apnea have all been reported with the use of brimonidine (Enyedi and Freedman, 2001).
Newer topical agents, including the prostaglandin analogues (latanoprost, bimatoprost, and travoprost) and the topical carbonic anhydrase inhibitors (dorzolamide and brinzolamide), are generally very safe in the pediatric population but are believed to be less effective than they are in adults (Beck, 2001). The topical carbonic anhydrase inhibitors, like systemic acetazolamide, are sulfonamide derivatives that should be avoided in the patient with sulfa sensitivity.
Miscellaneous Ophthalmologic Agents
Topical anesthetics, including cocaine, tetracaine, and proparacaine, are occasionally used by ophthalmologists in the perioperative setting. Cocaine is rarely used, but it is unique among the local anesthetics because of its vasoconstrictive properties. The potential for serious cardiovascular and central nervous system effects should be recognized by both the surgeon and anesthesiologist. The accepted maximum dose is 3 mg/kg, 1.5 mg/kg in the presence of volatile anesthetics. One drop of the 4% formulation contains approximately 1.5 mg of cocaine (McGoldrick, 1992b). The drug should not be used in patients with cardiovascular disease or in the presence of additional adrenergic-modifying medications such as monoamine oxidase inhibitors or tricyclic antidepressants.
Intraocular gases, including sulfur hexafluoride, perfluoropropane, and carbon octofluorine, are poorly diffusible inert gases that may be injected during certain vitreoretinal procedures. When nitrous oxide is present during injection, the nitrous oxide equilibrates with these new gas spaces to increase the volume and pressure of the intraocular injection, potentially compromising retinal perfusion. Animal studies, case reports, and mathematic models have demonstrated the necessity of discontinuing nitrous oxide no less than 15 minutes before intraocular gas injection and avoiding subsequent use of nitrous oxide for at least 4 weeks after the use of sulfur hexafluoride and 6 weeks after the use of perfluoropropane or carbon octofluorine (Wolf et al., 1983; McGoldrick, 1992b; Seaberg et al., 2002).
Effects of Various Anesthetic Agents on Intraocular Pressure
The central nervous system depressants (benzodiazepines, barbiturates, and opioids) commonly used by the anesthesiologist decrease IOP in both normal and glaucomatous eyes. The agents commonly used for preoperative anxiolysis in the pediatric population are associated with minor decreases in IOP that should not affect diagnostic measurements and likewise should not be relied on to attenuate the increase in IOP attributable to the use of succinylcholine and laryngoscopy. Effects specific to the use of oral or rectal midazolam in the pediatric population have not been delineated, although two studies of the use of intravenous (IV) midazolam in adults demonstrate minimal effects on IOP (Virkkila et al., 1992; Carter et al., 1999).
With the possible exception of ketamine, all of the IV induction agents are associated with a significant decrease in IOP. Thiopental and propofol reduced IOP by 40% and 53%, respectively, in one study, although both agents are unable to completely attenuate increases that are secondary to succinylcholine and laryngoscopy (Mirakhur and Shepherd, 1985; Mirakhur et al., 1987). Etomidate diminished IOP more profoundly than thiopental in one adult study (Calla et al., 1987). Etomidate-related myoclonus could be hazardous to the patient with traumatic injury and bothersome to the ophthalmologist. Early studies of the effects of ketamine uniformly demonstrated an increase in IOP, but subsequent studies in adults and children have demonstrated either insignificant changes or minor decreases in IOP (Peuler et al., 1975; Ausinsch et al., 1976). There is no clear consensus regarding the effects of ketamine on IOP, although its association with blepharospasm and nystagmus makes other induction agents more useful for ophthalmologic surgery.
All of the volatile anesthetics are associated with a dose-dependent decrease in IOP. Various postulated mechanisms include a reduction in aqueous humor production with a concomitant increase in outflow, relaxation of the supporting musculature, and depression of the central nervous system control center for IOP (McGoldrick, 1992c). As was previously demonstrated with halothane, reliable measurements of IOP may be made for approximately 10 minutes after mask induction with sevoflurane (Watcha et al., 1990; Yoshitake et al., 1993).
The deleterious effects of succinylcholine on IOP and the various methods of attenuating these effects have been evaluated by numerous investigators for several decades. The augmentation of IOP is thought to be mediated not only by tonic contractions of the extraocular muscles but also by dilation of the choroidal vasculature and relaxation of the orbital smooth muscle (Calobrisi and Lebowitz, 1990). In a study of patients undergoing elective enucleation, it was noted that the change in IOP after succinylcholine administration was the same in the normal eye as in the eye where the extraocular muscles were detached; therefore, it does not appear that extraocular muscle contraction significantly contributes to the increase in IOP after succinylcholine administration (Kelly et al., 1993). In adult patients with normal IOP, succinylcholine at doses between 1.5 and 2 mg/kg increased pressures by no more than 9 mm Hg, with peak effects demonstrated within 3 minutes after administration (Pandey et al., 1972). In patients who were not intubated, IOP was restored to baseline within 6 minutes, although other studies have demonstrated mild elevations that may persist for 30 minutes after succinylcholine administration. Whereas these effects of succinylcholine are significant in comparison with the effects of the nondepolarizing agents, they are clearly insignificant in comparison with the increase in IOP that is possible with laryngoscopy, coughing, and retching.
Numerous methods of blunting the rise in IOP secondary to succinylcholine and laryngoscopy have been evaluated, although none has demonstrated consistent or reliable efficacy. The results of early studies of pretreating patients with small doses of the nondepolarizing agents were promising but later refuted (Miller et al., 1968; Meyers et al., 1978). In two adult studies, the use of alfentanil was demonstrated to significantly attenuate the response to succinylcholine and intubation (Polarz et al., 1992; Eti et al., 2000). Another study comparing the effects of fentanyl and alfentanil demonstrated that although both agents were effective in attenuating the response to succinylcholine, fentanyl did not significantly attenuate the increase in IOP secondary to laryngoscopy (Sweeney et al., 1989). Early studies concerning the benefit of lidocaine before succinylcholine were discouraging, but lidocaine had favorable effects on IOP during laryngoscopy and intubation in subsequent investigations (Smith et al., 1979; Mahajan et al., 1987; Warner et al., 1989). The opioids and lidocaine may also facilitate gentle extubations after intraocular procedures and in patients with elevated IOP.
More contemporary methods of controlling IOP with the use of succinylcholine and laryngoscopy have been promising. Premedication with sublingual nifedipine and oral clonidine has demonstrated efficacy in the elderly population (Ghignone et al., 1988; Indu et al., 1989; Polarz et al., 1993). Intramuscular (IM) dexmedetomidine also effectively reduced IOP during regional anesthetic procedures in adults (Virkkila et al., 1994). None of these methods has been evaluated in the pediatric population.
More information regarding airway management and the effects on IOP are discussed in Chapter 30, Anesthesia for the Pediatric Trauma Patient. In addition, Vachon and others (2003) review the use of succinylcholine and the open globe.
General Anesthetic Considerations
Premedication
The value of premedication is well appreciated by all physicians providing anesthetic care to children. Premedication is useful to ease separation from parents and to provide for a smooth induction. Children between the ages of 1 and 6 years commonly benefit from premedication. Older children, especially those subject to repeated procedures, may also benefit from premedication or having a parent present during induction of anesthesia (Kain et al., 2006). Parental presence has also been shown to enhance the effects of oral midazolam on induction and emergence behavior (Arai et al., 2007). Oral midazolam (0.25 to 0.5 mg/kg) is commonly used and is generally effective within 10 to 20 minutes after administration (Coté et al., 2002). Nasal midazolam (0.2 mg/kg) may be useful in the patient refusing oral administration, but the acidity of the formulation is associated with a 71% incidence of burning and crying on administration (Karl et al., 1993). Oral clonidine (2 to 4 mcg/kg) also provides adequate anxiolysis within 30 minutes and has been demonstrated to decrease the incidence of PONV after strabismus surgery in two investigations (Mikawa et al., 1995; Handa and Fujii, 2001). In one study, oral clonidine was more effective than oral midazolam in multiple aspects of premedication, including acceptance by patients, more effective sedation, and better recovery from anesthesia (Almenrader et al., 2007). Neither midazolam nor clonidine consistently decreases the incidence of emergence delirium (Fazi et al., 2001; Valley et al., 2003). At recommended doses, neither of the agents should prolong the time required for discharge from the postoperative recovery unit.
General Anesthesia
Anesthesia can be maintained with any of the volatile agents, but the incidence of emergence delirium may be higher with the newer, less-soluble agents (Welborn et al., 1996; Lapin et al., 1999). Nitrous oxide is avoided if inert gas is to be injected into the eye. Nitrous oxide has been associated with an increased incidence of PONV in some adult studies, although similar effects cannot be demonstrated in the pediatric population (Hartung, 1996; Tramer et al., 1996, 1997). One study demonstrated no correlation between the use of nitrous oxide and the incidence of PONV in pediatric patients with strabismus (Kuhn et al., 1999). Maintenance with propofol may also be used, but three studies have demonstrated a higher incidence of dysrhythmias attributable to the OCR with propofol anesthesia (Watcha et al., 1991; Larsson et al., 1992; Tramer et al., 1998). Neuromuscular blockade is often indicated for intraocular procedures to ensure that the field remains motionless and that coughing or bucking does not result in damage to the eye and untoward increases in IOP.
A smooth emergence from anesthesia is desired after ophthalmologic procedures. This may be facilitated by deep extubation in the lateral position. Any of the inhaled agents may be safely used for deep extubation, although slightly more airway complications may occur with the use of desflurane (Valley et al., 2003). Small doses of IV lidocaine (0.5 to 1 mg/kg) may be administered before extubation if the depth of anesthesia is unclear.
Local And Regional Anesthesia
The primary methods of administering local anesthetics for ocular procedures include topical application, infiltration, and regional blockade. Conjunctival injection is often used to provide anesthesia for the treatment of retinopathy of prematurity (ROP). Topical application or infiltration may be useful in the cooperative older child to perform otherwise uncomfortable examinations and simple procedures such as foreign body removal and laceration repair. Regional techniques, including retrobulbar, peribulbar, and sub-Tenon’s blocks, are useful in adults as the primary anesthetic for a variety of ophthalmologic procedures, but they are less likely to be used as the sole anesthetic in children younger than 18 years of age (Johnson and Forrest, 1994). The benefits of regional techniques as adjuncts to general anesthesia in children are controversial.
The retrobulbar or intraconal block was first described in 1884. The block involves injection of local anesthetic into the posterior cone of the extraocular muscles and is effective in producing anesthesia and akinesia by blocking the ciliary ganglion and the oculomotor and abducens nerves. Hyaluronidase is a commonly used adjuvant that decreases the time for onset. The retrobulbar technique is not often used in the pediatric population. Complications of the retrobulbar block include stimulation of the OCR, retrobulbar hemorrhage, penetration of the optic nerve, intravascular injection, and brainstem anesthesia (McGoldrick, 1992e). One study evaluating the efficacy of retrobulbar blocks in combination with general anesthesia for pediatric strabismus surgery demonstrated no significant benefit, although the sample size was relatively small (Ates et al., 1998). An additional study of 45 patients showed a significant decrease in incidence and severity of OCR with retrobulbar block, and a decrease in PONV with retrobulbar block or topical local anesthesia (Gupta et al., 2007).
The peribulbar or periconal block has been used increasingly since it was first described in 1986. Because the cone of the extraocular muscles is not entered, the potential for intraocular and intradural injection is minimized, and the risk of retrobulbar hemorrhage and direct nerve injury is virtually eliminated (McGoldrick, 1992e). Disadvantages of the peribulbar block include a slightly higher failure rate (10% incidence) and an increased forward pressure on the globe secondary to the larger volumes of local anesthetic required (Zahl, 1992). Two pediatric studies that compared peribulbar blocks with IV meperidine for vitreoretinal and strabismus surgery demonstrated superior analgesia and significantly less PONV for up to 24 hours after surgery (Deb et al., 2001; Subramaniam et al., 2003). Parental satisfaction was also greater for those patients receiving adjunctive regional blocks.
The sub-Tenon’s block was first reported in adults in 1992 as a blunt-needle alternative to the sharp needle peribulbar or retrobulbar blocks (Stevens, 1992). Tenon’s capsule is a fascial sheath that envelops the eyeball and separates it from the orbital fat. The inner surface is separated from the outer surface of the sclera by a potential space called the episcleral or sub-Tenon’s space (Fig. 27-3). Typically, a blunt, curved cannula is inserted in the inferonasal quadrant under the Tenon’s capsule and 3 to 5 mL of local anesthesia are deposited in adults. In pediatric patients the volume is considerably less. The advantages include relatively painless insertion of the block and the ability to use a blunt needle with less opportunity to inject into unwanted areas of the eye. This block is associated with a higher incidence of chemosis and subconjunctival hemorrhage (Guise, 2003).
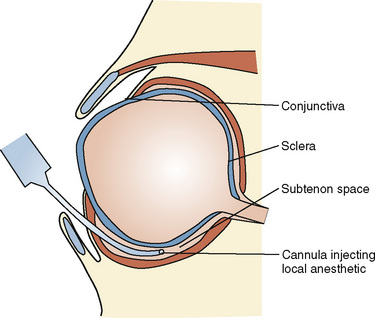
FIGURE 27-3 Anatomy of the sub-Tenon’s space
(From Ghai B et al.: Subtenon block compared to intravenous fentanyl for perioperative analgesia in pediatric cataract surgery, Anesth Analg 108:1132, 2009.)
The sub-Tenon’s block in conjunction with general anesthesia has been reported to decrease PONV, improve postoperative pain control, and reduce intraoperative analgesia requirements in patients having strabismus surgery (Steib et al., 2005). Ghai et al. (2009) in a prospective, randomized, blinded study of pediatric cataract patients compared sub-Tenon’s block (0.06 to 0.08 mL/kg 2% lidocaine with 0.5% bupivacaine [50:50 mixture]) to IV fentanyl (1 mcg/kg) and found significantly less pain and lower incidence of oculocardiac reflex. There was no statistically significant reduction in PONV, but parent satisfaction scores were higher with the sub-Tenon’s block. Additionally, it has been proposed as a technique in conjunction with sedation to perform laser surgery for ROP in neonates at the bedside (Parulekar et al., 2008).
Intraoperative and Postoperative Complications
Oculocardiac Reflex
The OCR was first described by two independent observers in 1908. The OCR is reported to occur at an incidence as high as 70% to 79% during pediatric ophthalmologic surgery (particularly strabismus surgery) without the use of prophylaxis (Ruta et al., 1996; Allen et al., 1998). The reflex is most often elicited by traction on the extraocular muscles but is also elicited by pressure on the eye and after intraorbital or retrobulbar injections. The reflex has been observed in the child who is congenitally anophthalmic and in the patient with an empty orbit after enucleation (Kerr and Vance, 1983; Ward and Bass, 2001). The anesthesiologist should be aware of the OCR and its potential consequences.
The OCR is mediated by the afferent ophthalmic division of the trigeminal nerve and the efferent vagal nerve (Fig. 27-4). The most common manifestation of the reflex is sinus bradycardia, but more ominous manifestations, including atrioventricular block, ventricular bigeminy, ventricular tachycardia, and asystole, have been described. Most studies define significant OCR-related bradycardia as a 10% to 20% decrease in the resting heart rate that is sustained for 5 seconds or longer. With sustained traction on one of the extraocular muscles, a counterregulatory adrenergic phase and restoration of heart rate occurs, which may be followed by a further increase in heart rate once traction is released (Braun et al., 1993). Most often, the initial bradycardia is associated with a variable degree of hypotension, although bradycardic hypertensive responses are also possible (Hahnenkamp et al., 2000).
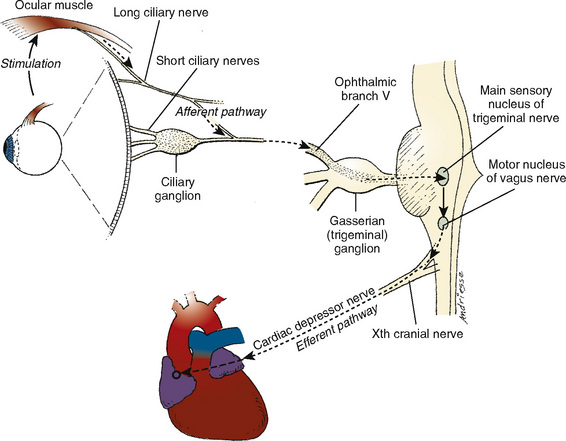
FIGURE 27-4 Anatomy and physiology of the oculocardiac reflex.
(From Vassallo SA, Ferrari LR: Anesthesia for ophthalmology. In Coté CJ et al., editors: A practice of anesthesia for infants and children, ed 2, Philadelphia, 1993, Saunders.)
When the reflex occurs intraoperatively, release of the stimulus is usually effective in ablating dysrhythmias within 10 to 20 seconds. If bradycardia persists or is worrisome, the patient may be given atropine (10 to 20 mcg/kg, IV) or glycopyrrolate (10 mcg/kg, IV). The initial effects of atropine should be evident within 20 seconds, and the maximal response is observed after 80 seconds (Braun et al., 1993). In the event that IV access is not available or is unreliable, one study has determined that intraglossal administration is superior to the IM route and in fact may be superior to IV administration (Arnold et al., 2002). It is generally safe to proceed with surgery once normal sinus rhythm at a rate within 10% of baseline is restored.
The value of prophylaxis has been debated for many years. There clearly is no method of completely eliminating the occurrence of the OCR. The consensus is that most pediatric patients (but not adults) undergoing strabismus surgery should be treated with IV atropine at a dose of 20 mcg/kg before manipulation (Steward, 1983). IV glycopyrrolate at a dose of 10 mcg/kg also has demonstrated efficacy with slightly less pronounced tachycardia. One randomized study of 120 children demonstrated a reduction in the incidence of OCR to 5% with glycopyrrolate and 2% with atropine given immediately after induction (Chisakuta and Mirakhur, 1995). Preoperative treatment by oral or IM routes is generally not warranted and is probably less effective (Mirakhur et al., 1982; Mirakhur, 1991). Opponents to prophylaxis with atropine maintain that possible dysrhythmias secondary to atropine itself (e.g., ventricular tachycardia, ventricular fibrillation, and left bundle branch block) may be more ominous and difficult to treat than the dysrhythmias associated with the OCR (Massumi et al., 1972; McGoldrick, 1992f). These effects are much more common in the adult patient. In otherwise healthy children, the tachycardic effects of atropine or glycopyrrolate should be safe and well tolerated.
Retrobulbar blocks are effective in preventing the OCR (Taylor et al., 1963; Braun et al., 1993; Deb et al., 2001); regional blocks are not commonly used during pediatric procedures and have triggered the reflex during placement. Topical lidocaine, applied directly to the medial rectus at 1 mg/kg, decreased the incidence of the OCR to 19% (vs. 79% in the control population); this technique has not been investigated since the initial study of 36 pediatric patients (Ruta et al., 1996).
The reflex may occur during regional and general anesthesia, and the depth of anesthesia has been thought to be irrelevant. However, there is one study using bispectral index (BIS) to estimate the depth of anesthesia that suggests a BIS of 40 to 50 seems to adequately prevent the OCR, compared with a BIS greater than 60 (Yi and Jee, 2008). Hypercarbia and hypoxemia, which may be more likely in the spontaneously breathing patient, are associated with a greater incidence of the bradycardic response. At least six studies have demonstrated that the choice of maintenance agents may influence the incidence or severity of the OCR. In one study of 39 pediatric patients who were mechanically ventilated through laryngeal mask airways, propofol anesthesia was associated with the greatest decrease in heart rate during a standardized traction of an extraocular muscle. Ketamine was associated with the least decrease in heart rate, whereas halothane and sevoflurane were associated with intermediate effects (Hahnenkamp et al., 2000). In another study of spontaneously breathing normocarbic patients who were not pretreated with atropine, sevoflurane was associated with a 38% incidence of OCR, whereas halothane was associated with an incidence of 79% (Allison et al., 2000). There is no discernable difference between the OCR provoked with desflurane vs. sevoflurane (Oh et al., 2007). Remifentanil appears to have an effect on the incidence of OCR. During strabismus surgery on children between 1 and 9 years of age without anticholinergic prophylaxis, those with maintenance of anesthesia using remifentanil had twice the incidence of OCR compared with those in the group receiving sevoflurane (Chung et al., 2008).
Postoperative Pain
For patients requiring minimal analgesia, rectal acetaminophen (30 to 40 mg/kg) may be administered after induction and followed with subsequent doses (20 mg/kg) at 6-hour intervals (Birmingham et al., 2001). Up to 120 minutes may be required to achieve peak serum levels. IV ketorolac (0.5 mg/kg) yields consistent analgesia for those procedures resulting in moderate postoperative pain (Dsida et al., 2002). Two studies of ketorolac administered for strabismus surgery have demonstrated analgesia equivalent to that provided by fentanyl (1 mcg/kg) or morphine (0.1 mg/kg) with a substantial reduction in PONV (Munro et al., 1994; Mendel et al., 1995). Another more recent study investigated the effect of topical tetracaine on postoperative pain control and emergence delirium. Although they were unable to make conclusions about emergence agitation, they did demonstrate that tetracaine drops improved pain control in the early postoperative period (Anninger et al., 2007).
Postoperative Nausea and Vomiting
Nausea and vomiting after ophthalmologic procedures are so common that they often serve as a model for the evaluation of various prophylactic techniques. Because nausea is subjective and difficult to measure in children, most studies look only at postoperative vomiting (POV). Several large scale studies report POV in children to occur at an incidence ranging from 13% to 42% after general anesthesia for all procedures (Rose and Watcha, 1999). The incidence of POV in untreated children after strabismus surgery ranges from 40% to 88% (Lerman, 1995). Older children and those receiving opioid analgesics are at greater risk. Whereas prophylaxis vs. symptomatic treatment is debated for low-risk procedures in children, it is generally accepted that prophylaxis should be provided for children undergoing strabismus surgery and all intraocular procedures regardless of demographics and the anesthetic technique employed. Retching and vomiting may be particularly detrimental after surgical or traumatic trespass of the globe. A summary of prophylactic measures is provided in Box 27-2.
One simple measure that does not involve the administration of any drug prophylaxis is the use of IV fluid therapy. In a comparison of pediatric patients undergoing strabismus surgery, the amount of fluid resuscitation was shown to make a difference in the incidence of PONV. POV was reported in 54% of the control group, who received 10 mL/kg per hour of lactated Ringer’s solution, vs. only 22% of the “superhydration group,” who received 30 mL/kg per hour of lactated Ringer’s solution (Goodarzi et al., 2005).
The efficacy of droperidol, a butyrophenone, has been demonstrated in numerous studies over the past 20 years. Low doses (20 mcg/kg) given after induction are as effective as higher doses (75 mcg/kg) and are less likely to prolong recovery time (Brown, et al., 1991). Lower doses (15 mcg/kg) in combination with ondansetron (100 mcg/kg, IV) are more effective than either drug given individually (Shende et al., 2001). The Food and Drug Administration (FDA) issued a black box warning in 2001 regarding the potential for fatal dysrhythmias associated with prolonged QTc intervals in rare instances when patients were given droperidol (Gan et al., 2002). No significant complications have been reported in children with the use of low-dose droperidol. However, considering a recent study showing doses of 25 mcg/kg of droperidol and 150 mcg/kg of ondansetron administered at induction of anesthesia to be equally effective in reducing the incidence and severity of POV, the pediatric anesthesiologist may find it easier to use alternative drugs than to comply with the rigorous monitoring recommendations made by the FDA (Bharti and Shende, 2003).
Ondansetron is a serotonin 5-HT3–receptor antagonist commonly used for prophylaxis and rescue therapy at doses ranging from 50 to 200 mcg/kg, IV. The half-life in children is slightly shorter than it is in adults (2.5 vs. 3.8 hours), and side effects are unusual in all populations (Culy et al., 2001). Administration either before or after surgical manipulation is acceptable with equivalent benefit (Madan et al., 2000). It has been demonstrated that doses of 75 mcg/kg are as effective as higher doses in the immediate postoperative period (Sadhasivam et al., 2000). Two studies have demonstrated that higher doses (150 to 200 mcg/kg) are required for significant benefit carried through the initial 24-hour postoperative period (Rose et al., 1994; Bowhay et al., 2001).
Other 5-HT3–receptor antagonists have been developed since 1991, primarily in an effort to provide a longer duration of action. Granisetron (20 to 40 mcg/kg, IV or orally [PO]) and dolasetron (12.5 mg or 350 mcg/kg, IV) have demonstrated efficacy in the pediatric strabismus population (Munro et al., 1999; Wagner et al., 2003). Tropisetron (200 mcg/kg up to 2 mg) has also been used in pediatric patients. As costs fall on these newer agents, their use may become more common.
Studies of dexamethasone as an antiemetic have been favorable, primarily in light of the agent’s low risk, low cost, long duration of action (up to 48 hours), and potential for augmenting postoperative analgesia (Splinter and Rhine, 1998). Many studies demonstrate its efficacy both alone and in conjunction with other antiemetics. In a dose response study, 250 mcg/kg, IV was found to be as effective as higher doses (Madan et al., 2005). The greatest reduction in POV after surgery for strabismus was reported in a study using low-dose dexamethasone (150 mcg/kg, maximum 8 mg) in combination with ondansetron (50 mcg/kg) after induction (Splinter, 2001). These results were upheld in a more recent study using 0.15 mg/kg of ondansetron and 0.2 mg/kg dexamethasone, resulting in only a 10% incidence of POV (Bhardwaj et al., 2004). One study comparing high-dose dexamethasone at 1 mg/kg (maximum of 25 mg) to ondansetron at 100 mcg/kg (maximum 4 mg) demonstrated a similar incidence of PONV during the initial 6 hours but significantly less during the subsequent 18 postoperative hours in those treated with dexamethasone (Subramaniam et al., 2001). The severity of POV and the number of patients requiring rescue medication were also significantly less in the dexamethasone group. Facial flushing is possible after dexamethasone, but there is no evidence in the literature for more detrimental side effects (e.g., hyperglycemia, delayed wound healing, or adrenal suppression) after single dose therapy (Subramaniam et al., 2001; Madan 2005).
Metoclopramide is a dopaminergic antagonist that provides central antiemetic action as well as increased gastric emptying and increased tone at the lower esophageal sphincter. There is inconsistent support in the literature for the use of metoclopramide for POV prophylaxis. Two randomized studies have demonstrated moderate benefit with metoclopramide at a dose of 250 mcg/kg given after induction, although two more recent studies failed to demonstrate any significant benefit (Broadman et al., 1990; Lin et al., 1992; Shende and Haldar, 1998; Kathirvel et al., 1999). A recent consensus panel failed to find evidence to recommend metoclopramide as a routine antiemetic (Gan, 2007).
Four early studies comparing propofol with halothane demonstrated a significant attenuation of POV after strabismus surgery when propofol was used (Watcha et al., 1991; Larsson et al., 1992; Reimer et al., 1993; Weir et al., 1993). The benefit was demonstrated primarily during the immediate postoperative period. A more recent study comparing propofol with isoflurane failed to demonstrate any significant benefit with propofol (Hamunen et al., 1997).
Specific Pathology and Surgical and Anesthetic Management
Examination under Anesthesia
For healthy children, mask induction and maintenance of spontaneous ventilation provide adequate conditions for the ophthalmologist and affording accurate control of anesthetic depth and a smooth emergence from anesthesia. Accurate measurements of IOP can be made for the initial 10 minutes after induction and maintenance with both sevoflurane and halothane (Watcha et al., 1990; Yoshitake et al., 1993). More lengthy examinations, including photographic retinal mapping and assessment of visual evoked potentials, may be performed with either propofol sedation or spontaneous ventilation with inhaled anesthetic agents and a laryngeal mask airway.
Retinopathy of Prematurity
Conditions associated with ROP include low birth weight, prematurity, oxygen exposure, mechanical ventilation, blood transfusions, intraventricular hemorrhage, sepsis, and vitamin E deficiency. ROP occurs in approximately 70% of infants who weigh less than 1000 g at birth. Fortunately, 80% to 90% of these infants have spontaneous regression of their retinal changes (Simons and Flynn, 1999; Moore, 2000). The greatest risk of oxidative damage to the developing retina appears to be in the first few weeks of life (Gaynon, 2006). It is clear that supplemental oxygen and relative hyperoxia are not the only factors responsible for development of the disease. Nevertheless, the immature retina is indisputably more susceptible to damage from the higher ex utero concentrations of oxygen and subsequent elevated levels of vascular endothelial growth factor (VEGF).
The immature retina responds to elevated oxygen tension (or another insult) by arrest of normal vasculogenesis and later by neovascularization and fibrous-tissue formation in the retina and vitreous humor. Retinal tears and detachment may occur secondary to contraction of the vitreous humor. Vasculogenesis takes place between 16 and 44 weeks’ postconception. In the normal developing retina, there is no clear border dividing the vascular and avascular tissue. This border becomes more prominent in those patients with ROP and forms the basis for staging the disease. The International Classification of Retinopathy of Prematurity (ICROP) was established in 1984 to uniformly describe the anatomic zone involved and the severity or stage of disease (Committee for the Classification of Retinopathy of Prematurity, 1984). This was revisited in 2005 with some modifications, including a description of a more virulent form of retinopathy in the tiniest babies (International Committee for the Classification of Retinopathy of Prematurity, 2005). Definitions for the various stages are provided in Box 27-3 and can be seen Figure 27-5. Threshold ROP, defined by specific ICROP criteria, is the stage of progression that is amenable to treatment.
Box 27-3 International Classification of Retinopathy of Prematurity Stages
From Committee for the Classification of Retinopathy of Prematurity: An international classification of retinopathy of prematurity, Pediatrics, 74:127, 1984.
Investigations have demonstrated the benefit of extremely limited oxygen supplementation in premature infants without ROP and the potential benefit of more liberal oxygen therapy in older infants with established prethreshold disease (Sinha and Tin, 2003). At one institution, rigorous guidelines restricting the use of oxygen greatly diminished the occurrence of advanced ROP and abolished the need for laser therapy over a 5-year period (Chow et al., 2003). The STOP-ROP multicenter controlled trial revealed that patients with established prethreshold disease are not harmed by oxygen supplementation and that a subset of these patients may benefit from higher arterial saturations (STOP-ROP Multicenter Study Group, 2000).
It is prudent to the anesthesiologist to limit oxygen supplementation in those infants without a diagnosis of ROP during the period of retinal vascularization. Older infants (older than 44 weeks’ postconceptional age) and those with established prethreshold disease are probably less vulnerable to higher oxygen tensions commonly provided during transports and general anesthesia. Anesthesia provided for nonophthalmologic procedures was once believed to increase the risk of ROP, but controlling for other risk factors has revealed that such a population is at no greater risk (Flynn, 1984).
Infants with threshold ROP are treated by cryotherapy or laser photocoagulation, depending on specific clinical findings. Cryotherapy involves placement of a probe chilled with nitrous oxide on the outer surface of the globe. Cryonecrosis of the underlying retinal tissues results in a decrease in the incidence of retinal detachment. Laser procedures (diode and argon lasers) are the preferred modality of therapy and are easier for the infant and the therapist than the cryotherapy technique. In many hospitals, these procedures are performed at the bedside in the neonatal intensive care unit. Both procedures may be performed with atropine, analgesics including opioids and ketamine, and local anesthesia that includes the sub-Tenon’s block (Lyon et al., 2008). Infants who are not intubated for the procedure may experience respiratory or hemodynamic instability, leading some neonatologists to electively intubate these infants for the procedure (Sullivan TJ et al., 1995). Cardiorespiratory disturbances may occur in 5% to 9% of all cases (Brown et al., 1990; Haigh et al., 1997). Some ophthalmologists prefer the benefits of general anesthesia in an operating-room setting. In a recent survey of pediatric ophthalmologists, 50% preferred general anesthesia with a controlled airway, whereas the rest used IV or oral sedation with local anesthesia (Chen et al., 2007). Variation in practice is because of the ophthalmologists’ beliefs and experiences, and also because of resource allocation, such as the availabilty of pediatric anesthesia. Once ROP progresses to stage 4 or 5, scleral buckle or vitectomy are performed to attempt to prevent blindness. These surgeries are almost always performed with general endotracheal anesthesia.
Strabismus
Strabismus has a prevalence of 3% to 5% in the pediatric population (Vivian, 2000). The disorder is most often idiopathic but may be associated with poor vision, cataracts, trauma, neuromuscular disorders, or one of several congenital syndromes (see Table 27-1). Surgical correction involves isolation of one or more of the extraocular muscles with subsequent recession (transection and reinsertion) or resection (shortening) of the muscle. Contemporary techniques involving botulinum toxin injection and postoperative adjustable sutures are not commonly used in the pediatric population. Amblyopia develops in approximately 50% of all patients with congenital esotropia and should be treated with occlusion therapy before surgical correction for strabismus (Guthrie and Wright, 2001).
General anesthesia may be provided by a flexible laryngeal mask airway, although endotracheal intubation with controlled ventilation may lessen the risk of hypercarbia and hypoxemia, which are known to increase the incidence and severity of the OCR (Blanc et al., 1988). Many ophthalmologists request the use of paralytic agents for performance of the forced duction test to more clearly differentiate paretic and restrictive disorders before surgical correction. This possibility should be discussed with the surgeon before formulating the anesthetic plan. Prolonged contractions of the extraocular muscles associated with the use of succinylcholine interfere with interpretation of the forced duction test for at least 15 minutes after administration (France et al., 1980). Its use is relatively contraindicated.
Masseter muscle rigidity after succinylcholine administration is four times more common in children anesthetized for strabismus surgery than in the general pediatric surgical population (Carroll, 1987). Because of the association of masseter muscle rigidity with malignant hyperthermia and an increased incidence of malignant hyperthermia in patients undergoing strabismus surgery, these patients are thought to be at a greater risk for the development of malignant hyperthermia (Strazis and Fox, 1993) (see Chapter 37, Malignant Hyperthermia).
Prophylaxis for both the OCR and PONV are critical for the patient undergoing strabismus repair. Postoperative pain is generally mild and of conjunctival origin. Acetaminophen, IV ketorolac, or both are usually effective. Topical analgesics (ketorolac, diclofenac, and benoxinate [oxybuprocaine]) have been evaluated in the strabismus population, and clear advantages over systemic agents and uniform efficacy have not been demonstrated (Morton et al., 1997; Bridge et al., 2000).
Lacrimal Apparatus Dysfunction
Congenital nasolacrimal duct obstruction is present in 60% to 70% of all infants at birth; spontaneous resolution is observed in 96% of these children by 1 year of age (Freitag and Woog, 2000). Congenital obstructions are most often isolated findings, but they may be associated with a variety of syndromes and craniofacial defects (Table 27-1). Acquired nasolacrimal duct obstruction is uncommonly encountered in the pediatric population but may be secondary to trauma, granulomatous disease, and systemic neoplasms (leukemia and lymphoma). The anatomy of the nasolacrimal duct system is illustrated in Figure 27-6.
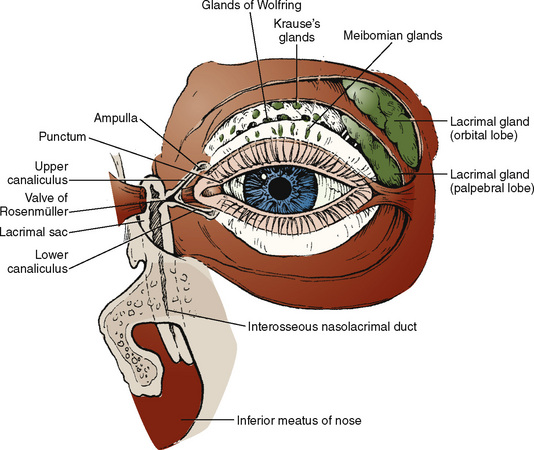
FIGURE 27-6 Anatomy of the lacrimal duct apparatus.
(From Nowinski TS: Anatomy and physiology of the lacrimal system. In Bosniak S, editor: Principles and practice of ophthalmic plastic and reconstructive surgery, vol 2, Philadelphia, 1996, Saunders.)
The initial surgical management of congenital obstruction usually requires only simple probing to establish patency. A small Bowman probe is inserted through one or both of the puncti, through the lacrimal sac, and subsequently into the nasolacrimal duct to pierce the valve of Hasner beneath the inferior turbinate (Freitag and Woog, 2000). The procedure is often atraumatic, requires no more than 5 to 10 minutes, and has been performed successfully in the office setting in children younger than 6 months of age. Usually the procedure is performed with general mask anesthesia. As with similar noninvasive procedures, IV access is not absolutely required for patients who are easily ventilated with a mask. Patients with significant comorbidities may be more safely cared for with IV access (Eippert et al., 1998). Although transient bacteremia has been demonstrated after nasolacrimal duct probing, the benefit of antibiotics to prevent endocarditis is questionable (Wilson et al., 2007).
The lacrimal system may be irrigated with saline or fluorescein after the procedure to demonstrate patency. Preventing excessive pooling, possible laryngospasm, and aspiration may require only head-down positioning and careful suctioning through the ipsilateral naris or oropharynx. Some anesthesiologists believe that patients are better managed with intubation to provide optimal protection of the airway (Johnson and Forrest, 1994). Analgesic requirements after nasolacrimal probing and irrigation are negligible.
Secondary surgical management of nasolacrimal obstruction may include infracture of the inferior turbinate, placement of silicone tubing that remains in place for 3 to 12 months, and dacryocystorhinostomy (Freitag and Woog, 2000). Dacryocystorhinostomy is a procedure used to bypass the nasolacrimal duct by creating an anastomosis between the lacrimal sac and the nasal mucosa beneath the middle turbinate. A small incision is made just medial to the medial canthus and overlying the lacrimal sac. These more complex procedures may require up to 2 hours to perform and may be complicated by appreciable blood loss. They require general anesthesia with endotracheal intubation. Topical vasoconstrictors may be used to minimize bleeding at the highly vascular nasal mucosa, and pharyngeal packing is usually beneficial. Acetaminophen, ketorolac, or small doses of opioids should provide adequate postoperative analgesia.
Cataracts
The prevalence of pediatric cataracts is between 1.2 and 6:10,000 live births. Bilateral cataracts are most commonly associated with systemic disease (see Table 27-1), whereas unilateral cataracts are more often idiopathic (Russell-Eggitt, 2000; Fallaha and Lambert, 2001). Surgery for bilateral congenital cataracts must be performed within the first several weeks of life to allow for normal retinal development. Unilateral congenital disease requires surgical attention within the first 4 months of life to prohibit the development of irreversible amblyopia.
Lens extraction is performed after maximal mydriasis with one or more of the topical agents. Ultrasonic phacoemulsification (commonly used in adults) is not often used in the pediatric population because of the pliable nature of the immature lens. Vitrectomy instrumentation is the preferred method of extraction in young children; the entire procedure is typically performed with only one intraocular instrument. Capsular opacification occurs often in children, and a capsulectomy is usually performed at the time of primary surgery. Intraocular lens implants are commonly provided to children older than 6 months with unilateral disease and children older than 1 year with bilateral disease (Fallaha and Lambert, 2001).
Anesthesia for lens extraction should provide complete akinesia and meticulous control of IOP. Because most of these patients are infants, controlled ventilation with muscle relaxants provides optimal operating conditions. The anesthesiologist should be aware of associated systemic disease and the potential systemic effects of the topical preparations used by the ophthalmologist. Rarely (1:200,000), maximal pupillary dilation requires an infusion of epinephrine delivered and then continuously aspirated from the anterior chamber. Attention to the electrocardiogram tracing permits detection of systemic absorption, which fortunately is uncommon (McGoldrick, 1992b). Analgesic requirements are usually minimal and under normal circumstances may be met with acetaminophen.
Postoperative apnea has been reported in the otherwise healthy full-term infant after cataract surgery (Tetzlaff et al., 1988). Speculation has associated ocular pathology or procedures with postoperative apnea in the otherwise healthy infant, but this association has yet to be clearly described.
Surgical postoperative complications include the formation of secondary membranes, glaucoma, and endophthalmitis (Fallaha and Lambert, 2001). Endophthalmitis may be avoided by treating nasolacrimal duct obstruction before cataract surgery and postponing cataract surgery in children with evidence of upper respiratory tract infection.
Glaucoma
Pediatric glaucomas are a diverse group of disorders with a prevalence of 1:10,000 live births (Khaw et al., 2000). Pediatric glaucoma is most often a congenital abnormality inherited in an autosomal recessive pattern that is slightly more prevalent in males (McGoldrick, 1992f). Only 10% of pediatric glaucoma diagnoses are associated with systemic disease, congenital disorders, or other ocular abnormalities. The diagnosis may be suspected in the patient who has buphthalmia, epiphora, photophobia, or corneal clouding (Khaw et al., 2000). The definitive diagnosis is made only by tonometry, corneal examination, funduscopy, and gonioscopy (examination of the iridocorneal angle).
Surgical management varies between patients and is dependent on the measurements and observations made under general anesthesia. Corrective procedures include goniotomy, trabeculotomy, trabeculectomy, and cyclocryotherapy (Beck, 2001). Goniotomy is a relatively brief procedure, whereas the other surgical techniques may require longer periods of general anesthesia. Patients often require repeated evaluations, because surgical correction is typically incremental and performed in carefully monitored stages.
The anesthetic management is quite similar for all procedures. A careful review of the past medical history, comorbid conditions, and concurrent pharmacologic therapy is crucial (see Tables 27-1 and 27-2). Premedication with atropine or glycopyrrolate is acceptable, but the use of scopolamine is generally contraindicated in the patient with glaucoma. Mask inductions are acceptable and followed by gentle laryngoscopy and placement of an oral Ring-Adair-Elwyn (RAE) endotracheal tube. Stable IOP and complete akinesia must be maintained throughout the surgical procedure. Neostigmine with glycopyrrolate or atropine may be safely administered for reversal of neuromuscular blockade; both agents have only minimal ocular effects at the routinely recommended doses. Unlike many other ocular procedures, surgery for glaucoma (particularly cyclocryotherapy) may be associated with moderate to severe postoperative pain. Opioids, in conjunction with prophylaxis for PONV, should be incorporated into the anesthetic plan.
Retinoblastoma
Retinoblastoma is the most common intraocular malignancy in the pediatric population with a prevalence of 1:20,000 live births. Fifty percent of all cases are secondary to mutations of the retinoblastoma gene, although only 25% of patients with the heritable form of the disease have a positive family history (Moore, 2000). The diagnosis is generally made by 3 years of age. Children with a positive family history have a 5% risk for developing retinoblastoma; these patients require regular examinations until the age of 5 years.
Therapy for retinoblastoma varies according to the severity of disease and has evolved dramatically since the 1970s. Treatment modalities may include combinations of enucleation, external beam radiation, localized radiotherapy, laser ablation, thermotherapy, cryotherapy, and chemotherapy (Uusitalo and Wheeler, 1999). Enucleation of the affected eye (or the more involved eye in bilateral disease) followed by external beam radiation was the cornerstone of treatment in the 1990s, leading to survival of over 90%. However, the risk of second cancers in the field of radiation began to appear with the risk increasing by 10% per decade of life. Through chemoreduction and focal ablation methods, many children with retinoblastoma are spared enucleation and serial external beam radiotherapy (Shields and Shields, 1999). Candidates for external beam radiotherapy include patients with bilateral disease that requires enucleation of the more involved eye and patients with diffuse vitreous and subretinal seeding.
External beam radiotherapy may require as many as 24 radiation sessions and anesthetics over the course of 4 to 6 weeks. Each session is of a few minutes’ duration, but the session requires the patient to remain motionless. Various anesthetic methods have been used, including rectal or IM methohexital, IV or IM ketamine, and brief inhalation anesthetics by insufflation methods, laryngeal mask airway, and endotracheal intubation (McGoldrick, 1992f). When a central venous catheter has been placed for adjuvant chemotherapy, a single bolus of propofol is probably the most effective method of providing the required 2 to 3 minutes of anesthesia with minimal recovery requirements.
Vitreoretinal Disorders
Retinal tears and detachment in the pediatric population are most often secondary to ROP but may also result from trauma and vitreous degeneration common to certain syndromes (see Table 27-1). Small tears may be amenable to laser therapy or cryopexy, but more significant tears and detachment often require complex surgical management and up to 3 hours of general anesthesia. Surgical options include scleral buckling (in combination with cryopexy), closed vitrectomy, and open-sky vitrectomy (Hunter et al., 2000).
The scleral buckle procedure involves the attachment of a tiny sponge or silicone band that constricts the sclera and holds the retina in position (Fig. 27-7). The scleral buckle remains permanently attached to the eye and may restrict normal growth of the child’s eye if tension is not released with subsequent surgery. Vitrectomy may be considered in the presence of a failed scleral buckle, for high retinal detachment, and for media opacification. The closed vitrectomy is slightly more difficult in the pediatric population and involves lensectomy for segmentation and removal of the vitreous by microvitreoretinal blades and vitrectomy instruments. The open-sky technique involves complete removal of the cornea, lensectomy, and en bloc removal of the fibrous mass filling the funnel of the detached retina (Hunter et al., 2000).
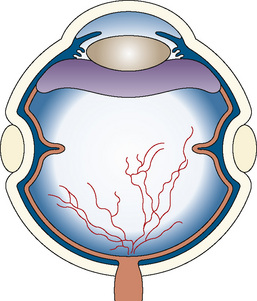
FIGURE 27-7 Scleral buckle.
(From Sears J, Capone A: Retinopathy of prematurity. In Yanoff M, Duker JS, editors: Ophthalmology, Philadelphia, 1999, Mosby.)
Anesthetic considerations for such procedures include prophylaxis for the OCR (the extraocular muscles are often bridled to permit optimal positioning), complete neuromuscular blockade, and the potential need to lower IOP with agents such as mannitol and acetazolamide. Silicone oil or one of the long-acting inert gases may be injected into the vitreous chamber at the conclusion of the procedure in an effort to improve surgical success (see Table 27-2). Should reoperation be necessary within a month of injection of an inert gas, nitrous oxide may be contraindicated because of its potential to expand the gaseous space.
Traumatic Injury and Ruptured Globe
The optimal management for the patient with ocular injury has been widely debated for many years, although many contemporary pundits consider the controversy mundane. Ophthalmic injuries are most often superficial, but a disproportionate number of serious penetrating injuries occur in children (Johnson and Forrest, 1994). It is often difficult to differentiate the emergent case (penetrating injury) from the nonemergent (nonpenetrating injury) case in children without examination under general anesthesia.
The importance of maintaining a quiet environment in the preoperative period cannot be overemphasized. Crying and thrashing can have devastating effects on IOP. Forceful eyelid closure may increase IOP by as much as 70 mm Hg (McGoldrick, 1993). Venous cannulation can be facilitated by pentobarbital, oral or rectal midazolam, and transdermal anesthetics. Access subsequently permits the administration of additional anxiolytics, analgesics, and prophylaxis for aspiration. Once sedation is adequate, the injured eye should be patched to provide comfort and minimize ocular movement.
When IV access is available, the anesthesiologists must consider the risks and benefits of succinylcholine (Vachon et al., 2003). Current consensus remains in favor of succinylcholine for rapid provision of optimal intubating conditions and reliable attenuation of coughing and bucking immediately after the endotracheal tube is secured. It is widely accepted that succinylcholine increases IOP despite various methods of blunting the response, but it is also clear that the succinylcholine-induced rise in IOP is inconsequential in relation to the possible rise with laryngoscopy and intubation (Cunningham and Barry, 1986). Support for the use of succinylcholine is derived from feline models of both anterior and posterior segment trauma and retrospective chart reviews and testimonials from the Wills Eye Hospital and the Massachusetts Eye and Ear Infirmary (Libonati et al., 1985; Donlon, 1986; Moreno et al., 1991).
Some anesthesiologists use nondepolarizing neuromuscular blockers for modified rapid sequence inductions in patients with penetrating ocular injuries. The nondepolarizing agents provide either no effect on or a slight decrease in IOP. Three described methods for accelerating the onset of the older nondepolarizing agents are divided doses of pancuronium, high-dose vecuronium, and synergism with d-tubocurarine and pancuronium (Mehta et al., 1985; Abbott and Samuel, 1987; Abdulla, 1993). Most anesthesiologists concede that none of these techniques provides the speed and reliability of succinylcholine. Rocuronium (1.2 mg/kg) has been demonstrated to have no effect on IOP and may provide intubating conditions comparable to succinylcholine within 60 seconds (Mazurek et al., 1998; Mitra et al., 2001; Perry et al., 2003). For patients with comorbid conditions that mandate avoidance of succinylcholine, rocuronium is probably the most effective alternative.
Summary
For questions and answers on topics in this chapter, go to “Chapter Questions” atwww.expertconsult.com.
Abbott M.A., Samuel J.R. The control of intra-ocular pressure during the induction of anaesthesia for emergency eye surgery: a high-dose vecuronium technique. Anaesthesia. 1987;42:1008.
Abdulla W.Y. The synergistic effect of two different nondepolarizing muscle relaxants on intraocular pressure. J Clin Anesth. 1993;5:5.
Allen L.E., Sudesh S., Sandramouli S., et al. The association between the oculocardiac reflex and post-operative vomiting in children undergoing strabismus surgery. Eye. 1998;12(Pt 2):193.
Allison C.E., De Lange J.J., Koole F.D., et al. A comparison of the incidence of the oculocardiac and oculorespiratory reflexes during sevoflurane or halothane anesthesia for strabismus surgery in children. Anesth Analg. 2000;90:306.
Almenrader N., Passariello M., Coccetti B., et al. Premedication in children: a comparison of oral midazolam and oral clonidine. Paediatr Anaesth. 2007;17:1143-1149.
Anninger W., Forbes B., Quinn G., et al. The effect of topical tetracaine eye drops on emergence behavior and pain relief after strabismus surgery. J AAPOS. 2007;11:273-276.
Arai Y.P., Ito H., Kandatsu N., et al. Parental presence during induction enhances the effect of oral midazolam on emergence behavior of children undergoing general anesthesia. Acta Anaesthesiol Scand. 2007;51:858-861.
Arnold R.W., Farah R.F., Monroe G. The attenuating effect of intraglossal atropine on the oculocardiac reflex. Binocul Vis Strabismus Q. 2002;17:313.
Ates Y., Unal N., Cuhruk H., et al. Postoperative analgesia in children using preemptive retrobulbar block and local anesthetic infiltration in strabismus surgery. Reg Anesth Pain Med. 1998;23:569.
Ausinsch B., Rayburn R.L., Munson E.S., et al. Ketamine and intraocular pressure in children. Anesth Analg. 1976;55:773.
Bailey P.L. Timolol and postoperative apnea in neonates and young infants. Anesthesiology. 1984;61:622.
Bauer C.R., Trottier M.C., Stern L. Systemic cyclopentolate (Cyclogyl) toxicity in the newborn infant. J Pediatr. 1973;82:501.
Baum V., O’Flaherty J. Anesthesia for genetic, metabolic and dysmorphic syndromes of childhood. Philadelphia: Lippincott Williams and Wilkins, 2006.
Beck A. Diagnosis and management of pediatric glaucoma. Ophthalmol Clin North Am. 14, 2001.
Bhardwaj N., Bala I., Kaur C., et al. Comparison of ondansetron with ondansetron plus dexamethasone for antiemetic prophylaxis in children undergoing strabismus surgery. J Pediatr Ophthalmol Strabismus. 2004;41:100-104.
Bharti N., Shende D. Comparison of anti-emetic effects of ondansetron and low-dose droperidol in pediatric strabismus surgery. J Pediatr Ophthalmol Strabismus. 2003;40:23-26.
Birmingham P.K., Tobin M.J., Fisher D.M., et al. Initial and subsequent dosing of rectal acetaminophen in children: a 24-hour pharmacokinetic study of new dose recommendations. Anesthesiology. 2001;94:385.
Blanc V.F., Jacob J.L., Milot J., et al. The oculorespiratory reflex revisited. Can J Anaesth. 1988;35:468.
Borromeo-McGrail V., Bordiuk J.M., Keitel H. Systemic hypertension following ocular administration of 10 per cent phenylephrine in the neonate. Pediatrics. 1973;51:1032.
Bowhay A.R., May H.A., Rudnicka A.R., et al. A randomized controlled trial of the antiemetic effect of 3 doses of ondansetron after strabismus surgery in children. Paediatr Anaesth. 2001;11:215.
Braun U., Feise J., Muhlendyck H. Is there a cholinergic and an adrenergic phase of the oculocardiac reflex during strabismus surgery? Acta Anaesthesiol Scand. 1993;37:390.
Bridge H.S., Montgomery C.J., Kennedy R.A., et al. Analgesic efficacy of ketorolac 0.5% ophthalmic solution (Acular) in paediatric strabismus surgery. Paediatr Anaesth. 2000;10:521.
Broadman L.M., Ceruzzi W., Patane P.S., et al. Metoclopramide reduces the incidence of vomiting following strabismus surgery in children. Anesthesiology. 1990;72:245.
Brown G., Tasman W., Naidoff M., et al. Systemic complications associated with retinal cryoablation for retinopathy of prematurity. Ophthalmology. 1990;97:855.
Brown R.Jr, James D., Weaver R., et al. Low-dose droperidol versus standard-dose droperidol for prevention of postoperative vomiting after pediatric strabismus surgery. J Clin Anesth. 1991;3:306.
Butera C., Plotnik J., Bateman J. Ocular genetics. In: Gallin P., editor. Pediatric ophthalmology. New York: Thieme Medical Publishing, Inc; 2000:78.
Calla S., Gupta A., Sen N., et al. Comparison of the effects of etomidate and thiopentone on intraocular pressure. Br J Anaesth. 1987;59:437.
Calobrisi B.L., Lebowitz P. Muscle relaxants and the open globe. Int Anesthesiol Clin. 1990;28:83.
Carroll J.B. Increased incidence of masseter spasm in children with strabismus anesthetized with halothane and succinylcholine. Anesthesiology. 1987;67:559.
Carter K., Faberowski L.K., Sherwood M.B., et al. A randomized trial of the effect of midazolam on intraocular pressure. J Glaucoma. 1999;8:204.
Chen S.D., Sundaram V., Wilkinson A., et al. Variation in anaesthesia for the laser treatment of retinopathy of prematurity-a survey of ophthalmologists in the UK. Eye. 2007;8:1033-1036.
Chisakuta A.M., Mirakhur R.K. Anticholinergic prophylaxis does not prevent emesis following strabismus surgery in children. Paediatr Anaesth. 1995;5:97.
Chow L.C., Wright K.W., Sola A. Can changes in clinical practice decrease the incidence of severe retinopathy of prematurity in very low birth weight infants? Pediatrics. 2003;111:339.
Chung C.J., Lee J.M., Choi S.R., et al. Effect of remifentanil on oculocardiac reflex in paediatric strabismus surgery. Acta Anaesthesiol Scand. 2008;52:1273-1277.
Comm.ittee for the Classification of Retinopathy of Prematurity. an international classification of retinopathy of prematurity. Pediatrics. 1984;74:127.
Cooper K., Singh K., Zimmerman T. Ocular pharmacology in children. In: Gallin P., editor. Pediatric ophthalmology. New York: Thieme Medical Publishers, Inc; 2000:92.
Coté C.J., Cohen I.T., Suresh S., et al. A comparison of three doses of a commercially prepared oral midazolam syrup in children. Anesth Analg. 2002;94:37.
Culy C.R., Bhana N., Plosker G.L. Ondansetron: a review of its use as an antiemetic in children. Paediatr Drugs. 2001;3:441.
Cunningham A.J., Barry P. Intraocular pressure-physiology and implications for anaesthetic management. Can Anaesth Soc J. 1986;33:195.
Deb K., Subramaniam R., Dehran M., et al. Safety and efficacy of peribulbar block as adjunct to general anaesthesia for paediatric ophthalmic surgery. Paediatr Anaesth. 2001;11:161.
DeFrances C.J., Hall M.J. 2005 National Hospital Discharge Survey. Advance data from vital and health statistics; no 385. Hyattsville, MD: National Center for Health Statistics, 2007.
Donlon J. Succinylcholine and open eye injury. Anesthesiology. 1986;64:524.
Dorland’s Illustrated Medical Dictionary. Philadelphia: WB Saunders, 2000.
Dsida R.M., Wheeler M., Birmingham P.K., et al. Age-stratified pharmacokinetics of ketorolac tromethamine in pediatric surgical patients. Anesth Analg. 2002;94:266.
Eippert G.A., Burnstine R.A., Bates J.H. Lacrimal-duct-probing-induced bacteremia: should children with congenital heart defects receive antibiotic prophylaxis? J Pediatr Ophthalmol Strabismus. 1998;35:38.
Ellis H., Feldman S. Anatomy for anaesthetists. Oxford, UK: Blackwell Science, 1997.
Enyedi L.B., Freedman S.F. Safety and efficacy of brimonidine in children with glaucoma. J AAPOS. 2001;5:281.
Eti Z., Yayci A., Umuroglu T., et al. The effect of propofol and alfentanil on the increase in intraocular pressure due to succinylcholine and intubation. Eur J Ophthalmol. 2000;10:105.
Everitt D.E., Avorn J. Systemic effects of medications used to treat glaucoma. Ann Intern Med. 1990;112:120.
Fallaha N., Lambert S. Pediatric cataracts. Ophthalmol Clin North Am. 14, 2001.
Fazi L., Jantzen E.C., Rose J.B., et al. A comparison of oral clonidine and oral midazolam as preanesthetic medications in the pediatric tonsillectomy patient. Anesth Analg. 2001;92:56.
Flynn J.T. Oxygen and retrolental fibroplasia: update and challenge. Anesthesiology. 1984;60:397.
France N.K., France T.D., Woodburn J.D.Jr, et al. Succinylcholine alteration of the forced duction test. Ophthalmology. 1980;87:1282.
Fraunfelder F.W., Fraunfelder F.T., Jensvold B. Adverse systemic effects from pledgets of topical ocular phenylephrine 10%. Am J Ophthalmol. 2002;134:624.
Freitag S., Woog J. Congenital nasolacrimal obstruction. Ophthalmol Clin North Am. 13, 2000.
Gan T.J. Society for ambulatory anesthesia guidelines for the management of postoperative nausea and vomiting. Anesth Analg. 2007;105:1615-1628.
Gan T.J., White P.F., Scuderi P.E., et al. FDA “black box” warning regarding use of droperidol for postoperative nausea and vomiting: is it justified? Anesthesiology. 2002;97:287.
Gaynon M.W. Rethinking STOP-ROP: Is it worthwhile trying to modulate excessive VEGF levels in prethreshold ROP eyes by systemic intervention? A review of the role of oxygen, light adaptation state, and anemia in prethreshold ROP. Retina. 2006;26:S18-S23.
Ghai B., Ram J., Makkar J.K., et al. Subtenon block compared to intravenous fentanyl for perioperative analgesia in pediatric cataract surgery. Anesth Analg. 2009;108(4):1132-1138.
Ghignone M., Noe C., Calvillo O., et al. Anesthesia for ophthalmic surgery in the elderly: the effects of clonidine on intraocular pressure, perioperative hemodynamics, and anesthetic requirement. Anesthesiology. 1988;68:707.
Goodarzi M., Matar M.M., Shafa M., et al. A prospective randomized blinded study of the effect of intravenous fluid therapy on postoperative nausea and vomiting in children undergoing strabismus surgery. Pediatric Anesthesia. 2005;16:49-53.
Gross R., Pineyro A. Current use of ophthalmic beta blockers. J Glaucoma. 1997;6:188.
Guise P. Sub-Tenon anesthesia: a prospective study of 6,000 blocks. Anesthesiology. 2003;98:964-968.
Gupta N., Kumar R., Kumar S., et al. A prospective randomised double blind study to evaluate the effect of peribulbar block or topical application of local anaesthesia combined with general anaesthesia on intra-operative and postoperative complications during paediatric strabismus surgery. Anaesthesia. 2007;62:1110-1113.
Guthrie M.E., Wright K. Congenital esotropia. Ophthalmol Clin North Am. 2001:14.
Hahnenkamp K., Honemann C.W., Fischer L.G., et al. Effect of different anaesthetic regimens on the oculocardiac reflex during paediatric strabismus surgery. Paediatr Anaesth. 2000;10:601.
Haigh P.M., Chiswick M.L., O’Donoghue E.P. Retinopathy of prematurity: systemic complications associated with different anaesthetic techniques at treatment. Br J Ophthalmol. 1997;81:283.
Hamunen K., Vaalamo M.O., Maunuksela E.L. Does propofol reduce vomiting after strabismus surgery in children? Acta Anaesthesiol Scand. 1997;41:973.
Handa F., Fujii Y. The efficacy of oral clonidine premedication in the prevention of postoperative vomiting in children following strabismus surgery. Paediatr Anaesth. 2001;11:71.
Hartung J. Twenty-four of twenty-seven studies show a greater incidence of emesis associated with nitrous oxide than with alternative anesthetics. Anesth Analg. 1996;83:114.
Henderson B.A., Naveiras M., Butler N., et al. Incidence and causes of ocular surgery cancellations in an ambulatory surgical center. J Cataract Refract Surg. 2005;32:95-102.
Hunter D.G., Mukai S., Hirose T. Advanced retinopathy of prematurity. In: Albert D.M., Jakobiec F.A., editors. Principles and practice of ophthalmology. Philadelphia: WB Saunders; 2000:1936.
Indu B., Batra Y.K., Puri G.D., et al. Nifedipine attenuates the intraocular pressure response to intubation following succinylcholine. Can J Anaesth. 1989;36:269.
International Committee for the Classification of Retinopathy of Prematurity. the International Classification of Retinopathy of Prematurity revisited. Arch Ophthal. 2005;123:991-999.
Johnson R., Forrest F. Local and general anaesthesia for ophthalmic surgery. Oxford, UK: Butterworth-Heinemann Medical, 1994.
Kain Z.N., Mayes L.C., Caldwell-Andrews A.A., et al. Predicting which children benefit most from parental presence during induction of anesthesia. Paediatr Anaesth. 2006;16:627-634.
Karl H.W., Rosenberger J.L., Larach M.G., et al. Transmucosal administration of midazolam for premedication of pediatric patients: comparison of the nasal and sublingual routes. Anesthesiology. 1993;78:885.
Karl H.W., Swedlow D.B., Lee K.W., et al. Epinephrine-halothane interactions in children. Anesthesiology. 1983;58:142.
Kathirvel S., Shende D., Madan R. Comparison of anti-emetic effects of ondansetron, metoclopramide or a combination of both in children undergoing surgery for strabismus. Eur J Anaesthesiol. 1999;16:761.
Kelly R.E., Dinner M., Turner L.S., et al. Succinylcholine increases intraocular pressure in the human eye with the extraocular muscles detached. Anesthesiology. 1993;79:948.
Kennerdell J.S., Wucher F.P. Cyclopentolate associated with two cases of grand mal seizure. Arch Ophthalmol. 1972;87:634.
Kerr W.J., Vance J.P. Oculocardiac reflex from the empty orbit. Anaesthesia. 1983;38:883.
Khaw P., Narita A., Armas R., et al. The paediatric glaucomas. In: Moore A., editor. Fundamentals of clinical ophthalmology: paediatric ophthalmology. London: BMJ Books; 2000:92.
Kuhn I., Scheifler G., Wissing H. Incidence of nausea and vomiting in children after strabismus surgery following desflurane anaesthesia. Paediatr Anaesth. 1999;9:521.
Lang S.A., Van der Wal M. Death from the oculocardiac reflex. Can J Anaesth. 1994;41:161.
Lapin S.L., Auden S.M., Goldsmith L.J., et al. Effects of sevoflurane anaesthesia on recovery in children: a comparison with halothane. Paediatr Anaesth. 1999;9:299.
Larsson S., Asgeirsson B., Magnusson J. Propofol-fentanyl anesthesia compared to thiopental-halothane with special reference to recovery and vomiting after pediatric strabismus surgery. Acta Anaesthesiol Scand. 1992;36:182.
Lerman J. Are antiemetics cost-effective for children? Can J Anaesth. 1995;42:263.
Libonati M.M., Leahy J.J., Ellison N. The use of succinylcholine in open eye surgery. Anesthesiology. 1985;62:637.
Lin D.M., Furst S.R., Rodarte A. A double-blinded comparison of metoclopramide and droperidol for prevention of emesis following strabismus surgery. Anesthesiology. 1992;76:357.
Lyon F., Dabbs T., O’Meara M. Ketamine sedation during the treatment of retinopathy of prematurity. Eye. 2008;22:684-686.
Madan R., Bhatia A., Chakithandy S., et al. Prophylactic dexamethasone for postoperative nausea and vomiting in pediatric strabismus surgery: a dose ranging and safety evaluation study. Anesth Analg. 2005;100:1622-1626.
Madan R., Perumal T., Subramaniam K., et al. Effect of timing of ondansetron administration on incidence of postoperative vomiting in paediatric strabismus surgery. Anaesth Intensive Care. 2000;28:27.
Mahajan R.P., Grover V.K., Munjal V.P., et al. Double-blind comparison of lidocaine, tubocurarine and diazepam pretreatment in modifying intraocular pressure increases. Can J Anaesth. 1987;34:41.
Marshall B., Longnecker D. General anesthetics. In: Hardman J., Limbird L., editors. Goodman and Gilman’s the pharmacologic basis of therapeutics. New York: McGraw-Hill Medical Publishing; 2001:295.
Massumi R.A., Mason D.T., Amsterdam E.A., et al. Ventricular fibrillation and tachycardia after intravenous atropine for treatment of bradycardias. N Engl J Med. 1972;287:336.
Mazurek A.J., Rae B., Hann S., et al. Rocuronium versus succinylcholine: are they equally effective during rapid-sequence induction of anesthesia? Anesth Analg. 1998;87:1259.
McGoldrick K. Anatomy and physiology of the eye. In: McGoldrick K., editor. Anesthesia for ophthalmic and otolaryngologic procedures. Philadelphia: WB Saunders; 1992:176.
McGoldrick K. Anesthetic ramifications of ophthalmic drugs. In: McGoldrick K., editor. Anesthesia for ophthalmic and otolaryngologic surgery. Philadelphia: WB Saunders; 1992:227.
McGoldrick K. Anesthetics and intraocular pressure: Management of penetrating eye injuries. In: McGoldrick K., editor. Anesthesia for ophthalmic and otolaryngologic surgery. Philadelphia: WB Saunders; 1992:183.
McGoldrick K. Ocular pathology and systemic diseases: anesthetic implications. In: McGoldrick K., editor. Anesthesia for ophthalmic and otolaryngologic surgery. Philadelphia: WB Saunders; 1992:210.
McGoldrick K. Ophthalmologic and systemic complications of surgery and anesthesia. In: McGoldrick K., editor. Anesthesia for ophthalmic and otolaryngologic surgery. Philadelphia: WB Saunders; 1992:272.
McGoldrick K. Pediatric ophthalmic surgery: Anesthetic considerations. In: McGoldrick K., editor. Anesthesia for ophthalmic and otolaryngologic surgery. Philadelphia: WB Saunders; 1992:190.
McGoldrick K. The open globe: is an alternative to succinylcholine necessary? J Clin Anesth. 1993;5:1.
Mehta M.P., Choi W.W., Gergis S.D., et al. Facilitation of rapid endotracheal intubations with divided doses of nondepolarizing neuromuscular blocking drugs. Anesthesiology. 1985;62:392.
Mendel H.G., Guarnieri K.M., Sundt L.M., et al. The effects of ketorolac and fentanyl on postoperative vomiting and analgesic requirements in children undergoing strabismus surgery. Anesth Analg. 1995;80:1129.
Meyers E.F., Krupin T., Johnson M., et al. Failure of nondepolarizing neuromuscular blockers to inhibit succinylcholine-induced increased intraocular pressure, a controlled study. Anesthesiology. 1978;149:48.
Mikawa K., Nishina K., Maekawa N., et al. Oral clonidine premedication reduces vomiting in children after strabismus surgery. Can J Anaesth. 1995;42:977.
Miller R.D., Way W.L., Hickey R.F. Inhibition of succinylcholine-induced increased intraocular pressure by non-depolarizing muscle relaxants. Anesthesiology. 1968;29:123.
Mirakhur R.K. Anticholinergic drugs in anaesthesia. Br J Hosp Med. 1991;46:409.
Mirakhur R.K., Jones C.J., Dundee J.W., et al. IM or IV atropine or glycopyrrolate for the prevention of oculocardiac reflex in children undergoing squint surgery. Br J Anaesth. 1982;54:1059.
Mirakhur R.K., Shepherd W.F. Intraocular pressure changes with propofol (Diprivan): comparison with thiopentone. Postgrad Med J. 1985;61(Suppl 3):41.
Mirakhur R.K., Shepherd W.F., Darrah W.C. Propofol or thiopentone: effects on intraocular pressure associated with induction of anaesthesia and tracheal intubation (facilitated with suxamethonium). Br J Anaesth. 1987;59:431.
Mitra S., Gombar K.K., Gombar S. The effect of rocuronium on intraocular pressure: a comparison with succinylcholine. Eur J Anaesthesiol. 2001;18:836.
Moore A. Disorders of the vitreous and retina. In: Moore A., editor. Fundamentals of clinical ophthalmology: paediatric ophthalmology. London: BMJ Books; 2000:121.
Moore M., Weiskopf R., Eger E.2nd, et al. Arrhythmogenic doses of epinephrine are similar during desflurane or isoflurane anesthesia in humans. Anesthesiology. 1993;79:943.
Moreno R.J., Kloess P., Carlson D.W. Effect of succinylcholine on the intraocular contents of open globes. Ophthalmology. 1991;98:636.
Morton N.S., Benham S.W., Lawson R.A., et al. Diclofenac vs oxybuprocaine eye drops for analgesia in paediatric strabismus surgery. Paediatr Anaesth. 1997;7:221.
Munro H.M., Riegger L.Q., Reynolds P.I., et al. Comparison of the analgesic and emetic properties of ketorolac and morphine for paediatric outpatient strabismus surgery. Br J Anaesth. 1994;72:624.
Munro H.M., D’Errico C.C., Lauder G.R., et al. Oral granisetron for strabismus surgery in children. Can J Anaesth. 1999;46:45.
Navarro R., Weiskopf R.B., Moore M.A., et al. Humans anesthetized with sevoflurane or isoflurane have similar arrhythmic response to epinephrine. Anesthesiology. 1994;80:545.
Oh A., Yun M.J., Kim H.J., et al. Comparison of desflurane with sevoflurane for the incidence of oculocardiac reflex in children undergoing strabismus surgery. Br J Anaesth. 2007;99:262-265.
Olson R.J., Bromberg B.B., Zimmerman T.J. Apneic spells associated with timolol therapy in a neonate. Am J Ophthalmol. 1979;88:120.
Pandey K., Badola R.P., Kumar S. Time course of intraocular hypertension produced by suxamethonium. Br J Anaesth. 1972;44:191.
Parulekar M.V., Chen S.D.M., Patel C.K. Sub-Tenon’s local anaesthesia for the treatment of retinopathy of prematurity with diode laser. Eye. 2008;22:375-379.
Pensiero S., Da Pozzo S., Perissutti P., et al. Normal intraocular pressure in children. J Pediatr Ophthalmol Strabismus. 1992;29:79.
Perry J., Lee J., Wells G. Rocuronium versus succinylcholine for rapid sequence induction intubation (Cochrane Review). Cochrane Database Syst Rev. 2003. CD002788
Peuler M., Glass D.D., Arens J.F. Ketamine and intraocular pressure. Anesthesiology. 1975;43:575.
Polarz H., Bohrer H., Fleischer F., et al. Effects of thiopentone/suxamethonium on intraocular pressure after pretreatment with alfentanil. Eur J Clin Pharmacol. 1992;43:311.
Polarz H., Bohrer H., Martin E., et al. Oral clonidine premedication prevents the rise in intraocular pressure following succinylcholine administration. Ger J Ophthalmol. 1993;2:97.
Reimer E.J., Montgomery C.J., Bevan J.C., et al. Propofol anaesthesia reduces early postoperative emesis after paediatric strabismus surgery. Can J Anaesth. 1993;40:927.
Rosales T., Isenberg S., Leake R., et al. Systemic effects of mydriatics in low weight infants. J Pediatr Ophthalmol Strabismus. 1981;18:42.
Rose J.B., Martin T.M., Corddry D.H., et al. Ondansetron reduces the incidence and severity of poststrabismus repair vomiting in children. Anesth Analg. 1994;79:486.
Rose J.B., Watcha M.F. Postoperative nausea and vomiting in paediatric patients. BJA. 1999;83:1.
Russell-Eggitt I. Disorders of the lens. In: Moore A., editor. Fundamentals of clinical ophthalmology: paediatric ophthalmology. London: BMJ Books; 2000:111.
Ruta U., Mollhoff T., Markodimitrakis H., et al. Attenuation of the oculocardiac reflex after topically applied lignocaine during surgery for strabismus in children. Eur J Anaesthesiol. 1996;13:11.
Sadhasivam S., Shende D., Madan R. Prophylactic ondansetron in prevention of postoperative nausea and vomiting following pediatric strabismus surgery: a dose-response study. Anesthesiology. 2000;92:1035.
Seaberg R.R., Freeman W.R., Goldbaum M.H., et al. Permanent postoperative vision loss associated with expansion of intraocular gas in the presence of a nitrous oxide-containing anesthetic. Anesthesiology. 2002;97:1309.
Shende D., Bharti N., Kathirvel S., et al. Combination of droperidol and ondansetron reduces PONV after pediatric strabismus surgery more than single drug therapy. Acta Anaesthesiol Scand. 2001;45:756.
Shende D., Haldar M. Prophylactic metoclopramide administered immediately after the induction of anesthesia has no effect on the incidence of postoperative emesis after strabismus surgery. Ind Pediatr. 1998;35:237.
Shields C.L., Shields J.A. Recent developments in the management of retinoblastoma. J Pediatr Ophthalmol Strabismus. 1999;36:8.
Simons B.D., Flynn J.T. Retinopathy of prematurity and associated factors. Int Ophthalmol Clin. 1999;39:29.
Sinha S.K., Tin W. The controversies surrounding oxygen therapy in neonatal intensive care units. Curr Opin Pediatr. 2003;15:161.
Smith R.B. Death and the oculocardiac reflex. Can J Anaesth. 1994;41:760.
Smith R.B., Babinski M., Leano N. The effect of lidocaine on succinylcholine-induced rise in intraocular pressure. Can Anaesth Soc J. 1979;26:482.
Splinter W.M. Prevention of vomiting after strabismus surgery in children: dexamethasone alone versus dexamethasone plus low-dose ondansetron. Paediatr Anaesth. 2001;11:591.
Splinter W.M., Rhine E.J. Low-dose ondansetron with dexamethasone more effectively decreases vomiting after strabismus surgery in children than does high-dose ondansetron. Anesthesiology. 1998;88:72.
Steib A., Karcenty A., Calache E., et al. Effects of subtenon anesthesia combined with general anesthesia on perioperative analgesic requirements in pediatric strabismus surgery. Reg Anesth Pain Med. 2005;30:478-483.
Stevens J.D. A new local anesthesia technique for cataract extraction by one quadrant sub-Tenon’s infiltration. Br J Ophthalmol. 1992;76:670-674.
Steward D.J. Anticholinergic premedication for infants and children. Can Anaesth Soc J. 1983;30:325.
STOP-ROP Multicenter Study Group. Supplemental therapeutic oxygen for prethreshold retinopathy of prematurity (STOP-ROP), a randomized, controlled trial. I: Primary outcomes. Pediatrics. 2000;105:295.
Strazis K.P., Fox A.W. Malignant hyperthermia: a review of published cases. Anesth Analg. 1993;77:297.
Subramaniam B., Madan R., Sadhasivam S., et al. Dexamethasone is a cost-effective alternative to ondansetron in preventing PONV after paediatric strabismus repair. Br J Anaesth. 2001;86:84.
Subramaniam R., Subbarayudu S., Rewari V., et al. Usefulness of pre-emptive peribulbar block in pediatric vitreoretinal surgery: a prospective study. Reg Anesth Pain Med. 2003;28:43.
Sullivan T.J., Clarke M.P., Tuli R., et al. General anesthesia with endotracheal intubation for cryotherapy for retinopathy of prematurity. Eur J Ophthalmol. 1995;5:187.
Sweeney J., Underhill S., Dowd T., et al. Modification by fentanyl and alfentanil of the intraocular pressure response to suxamethonium and tracheal intubation. Br J Anaesth. 1989;63:688.
Taylor C., Wilson F., Roesch R. Prevention of the oculo-cardiac reflex in children. Anesthesiology. 1963;24:646.
Tetzlaff J.E., Annand D.W., Pudimat M.A., et al. Postoperative apnea in a full-term infant. Anesthesiology. 1988;69:426.
Tramer M., Moore A., McQuay H. Omitting nitrous oxide in general anaesthesia: meta-analysis of intraoperative awareness and postoperative emesis in randomized controlled trials. Br J Anaesth. 1996;76:186.
Tramer M., Moore A., McQuay H. Meta-analytic comparison of prophylactic antiemetic efficacy for postoperative nausea and vomiting: propofol anaesthesia vs omitting nitrous oxide vs total i.v. anaesthesia with propofol. Br J Anaesth. 1997;78:256.
Tramer M.R., Sansonetti A., Fuchs-Buder T., et al. Oculocardiac reflex and postoperative vomiting in paediatric strabismus surgery: a randomised controlled trial comparing four anaesthetic techniques. Acta Anaesthesiol Scand. 1998;42:117.
Ueda W., Hirakawa M., Mae O. Appraisal of epinephrine administration to patients under halothane anesthesia for closure of cleft palate. Anesthesiology. 1983;58:574.
Uusitalo M., Wheeler S. New approaches in the clinical management of retinoblastoma. Ophthalmol Clin North Am. 12, 1999.
Vachon C.A., Warner D.O., Bacon D.R. Succinylcholine and the open globe: tracing the teaching. Anesthesiology. 2003;99:220.
Valley R.D., Freid E.B., Bailey A.G., et al. Tracheal extubation of deeply anesthetized pediatric patients: a comparison of desflurane and sevoflurane. Anesth Analg. 2003;96:1320.
Virkkila M., Ali-Melkkila T., Kanto J. Premedication for outpatient cataract surgery: a comparative study of intramuscular alfentanil, midazolam and placebo. Acta Anaesthesiol Scand. 1992;36:559.
Virkkila M., Ali-Melkkila T., Kanto J., et al. Dexmedetomidine as intramuscular premedication for day-case cataract surgery: a comparative study of dexmedetomidine, midazolam and placebo. Anaesthesia. 1994;49:853.
Vivian A. The management of childhood strabismus. In: Moore A., editor. Fundamentals of clinical ophthalmology: paediatric ophthalmology. London: BMJ Books; 2000:177.
Wagner D., Pandit U., Voepel-Lewis T., et al. Dolasetron for the prevention of postoperative vomiting in children undergoing strabismus surgery. Paediatr Anaesth. 2003;13:522-526.
Ward B., Bass S. The oculocardiac reflex in a congenitally anophthalmic child. Paediatr Anaesth. 2001;11:372.
Warner L.O., Bremer D.L., Davidson P.J., et al. Effects of lidocaine, succinylcholine, and tracheal intubation on intraocular pressure in children anesthetized with halothane-nitrous oxide. Anesth Analg. 1989;69:687.
Watcha M.F., Chu F.C., Stevens J.L., et al. Effects of halothane on intraocular pressure in anesthetized children. Anesth Analg. 1990;71:181.
Watcha M.F., Simeon R.M., White P.F., et al. Effect of propofol on the incidence of postoperative vomiting after strabismus surgery in pediatric outpatients. Anesthesiology. 1991;75:204.
Weir P.M., Munro H.M., Reynolds P.I., et al. Propofol infusion and the incidence of emesis in pediatric outpatient strabismus surgery. Anesth Analg. 1993;76:760.
Welborn L.G., Hannallah R.S., Norden J.M., et al. Comparison of emergence and recovery characteristics of sevoflurane, desflurane, and halothane in pediatric ambulatory patients. Anesth Analg. 1996;83:917.
Williams E.L. Postoperative blindness. Anesthesiol Clin North America. 2002;20:367.
Wilson W., Taubert K., Gewitz M., et al. Prevention of infective endocarditis: guidelines from the American Heart Association. Circulation. 2007;116:1736-1754.
Wolf G.L., Capuano C., Hartung J. Nitrous oxide increases intraocular pressure after intravitreal sulfur hexafluoride injection. Anesthesiology. 1983;59:547.
Yi C., Jee D. Influence of the anaesthetic depth on the inhibition of the oculocardiac reflex during sevoflurane anaesthesia for paediatric strabismus surgery. Br J Anaesth. 2008;101:234-238.
Yoshitake S., Sendaya K., Mizutani A., et al. The changes of intraocular pressure during sevoflurane anesthesia in children. Masui. 1993;42:52.
Zahl K. Selection of techniques for regional blockade of the eye and adnexa. In: McGoldrick K., editor. Anesthesia for ophthalmic and otolaryngologic surgery. Philadelphia: WB Saunders; 1992:235.
Zimmerman T.J., Kooner K.S., Kandarakis A.S., et al. Improving the therapeutic index of topically applied ocular drugs. Arch Ophthalmol. 1984;102:551.
* Open-angle glaucoma, also known as chronic simple glaucoma, is a condition of elevated IOP in an eye with an anatomically open anterior chamber angle. The trabecular meshwork is thought to be sclerosed, resulting in inefficient aqueous filtration and drainage. Closed-angle glaucoma is a mechanical closing of the pathway for aqueous egress for the eye. The iris may move into direct contact with the posterior surface of the cornea, impeding the aqueous outflow path, or the crystalline lens may swell, resulting in papillary blocking. In the latter case, the lens blocks the route for aqueous humor to travel from the posterior to the anterior chamber.