Chapter 11 Carcinogenesis and neoplasia
GENERAL CHARACTERISTICS OF NEOPLASMS (TUMOURS)
Definitions
The word tumour means literally an abnormal swelling. However, in modern medicine, the word has a much more specific meaning. A tumour (neoplasm) is a lesion resulting from the autonomous or relatively autonomous abnormal growth of cells that persists after the initiating stimulus has been removed.
Tumours result from the neoplastic transformation of any nucleated cell in the body; the transformed cells are called neoplastic cells. By transformation involving a series of genetic alterations (e.g. mutations), cells escape permanently from normal growth regulatory mechanisms. The neoplastic cells in tumours designated malignant possess additional potentially lethal abnormal characteristics enabling them to invade and to metastasise, or spread, to other tissues.
Neoplastic cells grow to form abnormal swellings (except for leukaemias), but note that swellings or organ enlargement can also result from inflammation, cysts, hypertrophy or hyperplasia.
The term neoplasm (new growth) is synonymous with the medical meaning of the word tumour and is often used in preference because it is less ambiguous and not quite so alarming when overheard by patients. Cancer is a word used more in the public arena than in medicine; it has emotive connotations and generally refers to a malignant neoplasm.
Incidence of tumours
Malignant neoplasms—those that invade and spread and are therefore of greater clinical importance—develop in approximately 25% of the human population. The risk increases with age, but tumours can occur even in infancy (Fig. 11.1). The mortality rate is high, despite modern therapy, so that cancer accounts for about one-fifth of all deaths in developed countries. However, the mortality rate varies considerably between specific tumour types.
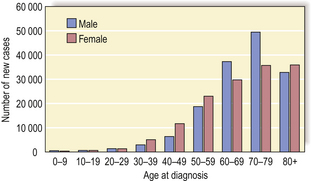
Fig. 11.1 Age and cancer incidence in England (2004). Cancer occurs at all ages but is most common over the age of 50 years.
(Based on data from the Office for National Statistics)
The relative incidence by diagnosis of various common types of cancer is shown in Fig. 11.2. Lung cancer is the most frequent malignant neoplasm in the UK and USA, and its importance is compounded by the extremely poor prognosis. In other countries other cancers are more common, and these differences often provide important aetiological clues.
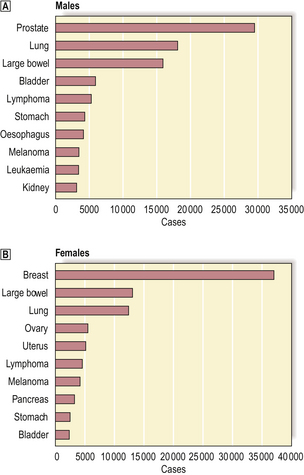
Fig. 11.2 Incidence of the ten most frequent cancers in the UK (2004). Top ten cancers in males.
Top ten cancers in females. The data exclude non-melanoma skin cancers (e.g. basal cell carcinoma) because, although common, they are so rarely fatal.
(Data published by Cancer Research UK, 2007)
For various reasons most epidemiological data on cancer incidence probably underestimate the true incidence. Not all tumours become clinically evident and, unless a thorough autopsy is performed, may never be detected. For example, autopsy surveys have revealed a higher than expected incidence of occult carcinoma of the prostate in elderly men, although these often minute lesions are probably of little clinical consequence. Cancer incidence may also be underestimated due to a failure of detection or diagnosis in countries and communities with poor health care.
Structure of tumours
Solid tumours consist of neoplastic cells and stroma (see below and Fig. 11.3). The neoplastic cells reproduce to a variable extent the growth pattern and synthetic activity of the parent cell of origin. Depending on their functional resemblance to the parent tissue, they continue to synthesise and secrete cell products such as collagen, mucin or keratin; these often accumulate within the tumour where they are recognisable histologically. Other cell products may be secreted into the blood where they can be used clinically to monitor tumour growth and the effects of therapy (p. 234).
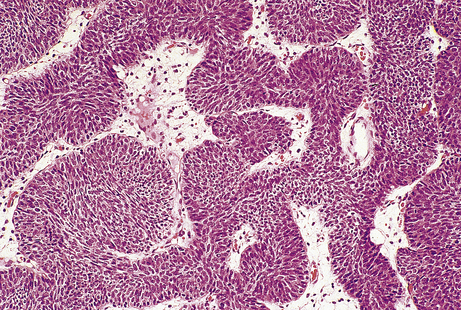
Fig. 11.3 Tumour cells and stroma. Histology of an epithelial neoplasm showing the darkly staining tumour cells embedded in a paler connective tissue stroma.
Stroma
The neoplastic cells are embedded in and supported by a connective tissue framework called the stroma (from the Greek word meaning a mattress), which provides mechanical support and nutrition to the neoplastic cells. The process of stroma formation, called a desmoplastic reaction when it is particularly fibrous, is due to induction of connective tissue proliferation by growth factors in the immediate tumour environment.
The stroma always contains blood vessels which perfuse the tumour (Fig. 11.4). The growth of a tumour is dependent upon its ability to induce blood vessels to perfuse it, for unless it becomes permeated by a vascular supply its growth will be limited by the ability of nutrients to diffuse into it, and the tumour cells will cease growing when the nodule has attained a diameter of no more than 1–2mm (Fig. 11.5). Angiogenesis in tumours is induced by factors such as vascular endothelial growth factor (VEGF). This action is opposed by factors such as angiostatin and endostatin which have potential in cancer therapy.
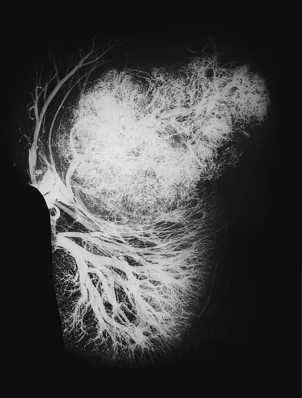
Fig. 11.4 Vascular stroma. Increased vascularity of a malignant tumour in a kidney, revealed by radiology after intra-arterial injection of X-ray contrast fluid.
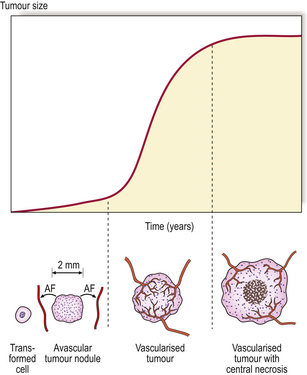
Fig. 11.5 Tumour angiogenesis. Neoplastic transformation of a single cell results in the growth of a tumour nodule, limited by the ability of nutrients to diffuse into it, to a diameter of 1–2 mm. Production of angiogenic factors (AF) stimulates the proliferation and ingrowth of blood vessels, enabling tumour growth to be supported by perfusion. Eventually, the tumour outgrows its blood supply, and areas of necrosis appear, resulting in slower growth.
Fibroblasts and the matrix they secrete give some mechanical support to the tumour cells and may in addition have nutritive properties. Stromal myofibroblasts are often abundant, particularly in carcinomas of the breast; their contractility is responsible for the puckering and retraction of adjacent structures.
The stroma often contains a lymphocytic infiltrate of variable density. This may reflect a host immune reaction to the tumour (Ch. 9), a hypothesis supported by the observation that patients whose tumours are densely infiltrated by lymphocytes tend to have a better prognosis.
Tumour shape and correlation with behaviour
The gross appearance of a tumour on a surface (e.g. gastrointestinal mucosa) may be described as sessile, polypoid, papillary, fungating, ulcerated or annular (Fig. 11.6). The behaviour of a tumour (i.e. whether it is benign or malignant) can often be deduced from its gross appearance: polypoid tumours are generally benign, i.e. unlikely to spread beyond the tissue of origin (Fig. 11.7); ulceration is more commonly associated with aggressive behaviour because invasion is the defining feature of malignancy (Fig. 11.8).
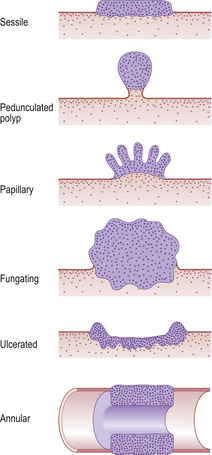
Fig. 11.6 Tumour shapes. Sessile, polypoid and papillary tumours are usually benign. Fungating, ulcerated or annular tumours are more likely to be malignant. Annular tumours encircling a tubular structure (e.g. intestine) are common in the large bowel, where they often cause intestinal obstruction.
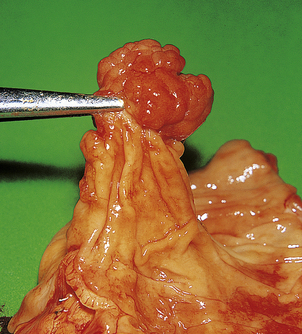
Fig. 11.7 Adenomatous polyp of the colon. This common lesion has a clearly visible stalk. Although benign, these lesions are precursors of adenocarcinoma of the large bowel. When seen endoscopically, adenomatous polyps can be removed by snaring the stalk.
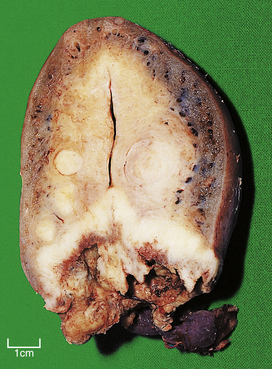
Fig. 11.8 Squamous cell carcinoma of the cervix. This uterus is invaded by a carcinoma arising in and destroying the cervix. The small round tumours in the myometrium are benign leiomyomas (‘fibroids’).
Ulcerated tumours can often be distinguished from non-neoplastic ulcers, such as peptic ulcers in the stomach, because the former tend to have heaped-up or rolled edges.
The shape of connective tissue neoplasms can be misleading. Although circumscription by a clearly defined border is one of the characteristics of benign epithelial tumours, some malignant connective tissue tumours are also well circumscribed.
Tumours are usually firmer than the surrounding tissue, causing a palpable lump in accessible sites such as the breasts. Extremely hard tumours are often referred to as ‘scirrhous’. Softer lesions are sometimes called ‘medullary’; they occur in the thyroid and breast.
The cut surfaces of malignant tumours are often variegated due to areas of necrosis and degeneration, but some, such as lymphomas and seminomas, appear uniformly bland.
Tumour histology
Neoplasms differ histologically from their corresponding normal tissue by various features; these are useful in diagnosis and include:
These features are often seen to their greatest degree in malignant neoplasms (Fig. 11.9).
CLASSIFICATION OF TUMOURS
Tumours are classified according to their behaviour and histogenesis (cell of origin).
Behavioural classification
The behavioural classification divides tumours into:
The principal pathological criteria for classifying a tumour as benign or malignant are summarised in Table 11.1. Some tumours, such as some ovarian tumours, defy precise behavioural classification, because their histology is intermediate between that associated with benign and malignant tumours; these are often referred to as ‘borderline’ tumours.
Table 11.1 Principal characteristics of benign and malignant tumours
Feature | Benign | Malignant |
---|---|---|
Growth rate | Slow | Relatively rapid |
Mitoses | Infrequent | Frequent and often atypical |
Histological resemblance to normal tissue | Good | Variable, often poor |
Nuclear morphology | Often normal | Usually hyperchromatic, irregular outline, multiple nucleoli and pleomorphic |
Invasion | No | Yes |
Metastases | Never | Frequent |
Border | Often circumscribed or encapsulated | Often poorly defined or irregular |
Necrosis | Rare | Common |
Ulceration | Rare | Common on skin or mucosal surfaces |
Direction of growth on skin or mucosal surfaces | Often exophytic | Often endophytic |
Benign tumours
Benign tumours remain localised. They are slowly growing lesions that do not invade the surrounding tissues or spread to other sites in the body. They are often enveloped by a thin layer of compressed connective tissue (i.e. encapsulated).
When a benign tumour arises in an epithelial or mucosal surface, the tumour grows away from the surface, because it cannot invade, often forming a polyp which may be either pedunculated (stalked) or sessile; this non-invasive outward direction of growth creates an exophytic lesion (Fig. 11.10). Histologically, benign tumours closely resemble the parent cell or tissue.
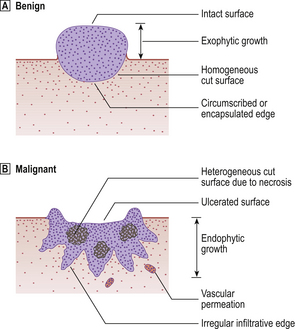
Fig. 11.10 Benign and malignant tumours growing on surfaces (e.g. skin, bowel wall), showing the principal differences in their gross appearances.
Although benign tumours are, by definition, confined to their site of origin, they may cause clinical problems due to:
Malignant tumours
Malignant tumours are, by definition, invasive. They are typically rapidly growing and poorly circumscribed. Histologically, they resemble the parent cell or tissue to a lesser extent than do benign tumours. Malignant tumours encroach on and destroy the adjacent tissues (Fig. 11.10), enabling the neoplastic cells to penetrate the walls of blood vessels and lymphatic channels and thereby disseminate to other sites. This important process is called metastasis and the resulting secondary tumours are called metastases. Patients with widespread metastases are often said to have carcinomatosis.
Not all tumours categorised as malignant exhibit metastatic behaviour. For example, basal cell carcinoma of the skin (rodent ulcer) rarely forms metastases, yet is regarded as malignant because it is highly invasive and destructive.
Malignant tumours on epithelial or mucosal surfaces may form a protrusion in the early stages, but eventually invade the underlying tissue; this invasive inward direction of growth gives rise to an endophytic tumour. Ulceration is common.
Malignant tumours in solid organs tend to be poorly circumscribed, often with strands of neoplastic tissue penetrating adjacent normal structures. The resemblance of the cut surface of these lesions to a crab (Latin: cancer) gives the disease its popular name. Malignant tumours often show central necrosis because of inadequate vascular perfusion.
The considerable morbidity and mortality associated with malignant tumours may be due to:
Histogenetic classification
Histogenesis—the specific cell of origin of an individual tumour—is determined by histopathological examination and specifies the tumour type. This is then incorporated in the name given to the tumour (e.g. squamous cell carcinoma).
Histogenetic classification includes numerous subdivisions, but the major categories of origin are:
Although some general differences exist between the main groups of malignant tumours (Table 11.2), individual lesions have to be categorised more precisely both in clinical practice and for epidemiological purposes. It is inadequate to label the patient’s tumour as merely having an epithelial or connective tissue origin; efforts must be made to determine the precise cell type. The classification of individual tumours is vitally important. Thorough histological examination of the tumour, sometimes using special techniques like genetic analysis and immunocytochemistry, detects subtle features that betray its provenance.
Table 11.2 Principal characteristics of carcinomas and sarcomas
Feature | Carcinoma | Sarcoma |
---|---|---|
Origin | Epithelium | Connective tissues |
Behaviour | Malignant | Malignant |
Frequency | Common | Relatively rare |
Preferred route of metastasis | Lymph | Blood |
In situ phase | Yes | No |
Age group | Usually over 50 years | Usually below 50 years |
Histological grade (degree of differentiation)
The extent to which the tumour resembles histologically its cell or tissue of origin determines the tumour grade (Fig. 11.11) or degree of differentiation. Benign tumours are not usually further classified in this way because they nearly always closely resemble their parent tissue and grading the degree of differentiation offers no further clinical benefit in terms of choosing the most appropriate treatment. However, the degree of differentiation of malignant tumours is clinically useful both because it correlates strongly with patient survival (prognosis), and because it often indicates the most appropriate treatment. Thus, malignant tumours are usually graded either as well, moderately or poorly differentiated, or numerically, often by strict criteria, as grade 1, grade 2 or grade 3.
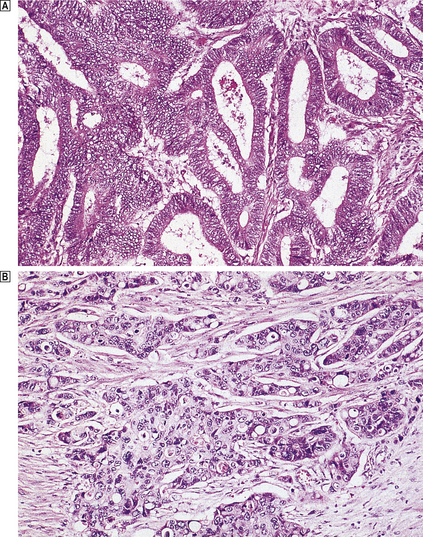
Fig. 11.11 Histological grading of differentiation. Well-differentiated adenocarcinoma of the colon characterised by glandular structures similar to those in normal mucosa.
Poorly differentiated adenocarcinoma of the colon characterised by a more solid growth pattern with little evidence of gland formation.
A well-differentiated tumour more closely resembles the parent tissue than does a poorly differentiated tumour, while moderately differentiated tumours are intermediate between these two extremes. Poorly differentiated tumours are more aggressive than well-differentiated tumours.
A few tumours are so poorly differentiated that they lack easily recognisable histogenetic features. There may even be great difficulty in deciding whether they are carcinomas or lymphomas, for example, although immunocytochemistry and genetic analysis often enable a distinction to be made. Tumours defying precise histogenetic classification are often referred to as ‘anaplastic’, or by some purely descriptive term such as ‘spindle cell’ or ‘small round cell’ tumour. Fortunately, advances in diagnostic histopathology have resulted in considerably fewer unclassifiable tumours and these descriptive terms are rapidly becoming obsolete.
NOMENCLATURE OF TUMOURS
Tumours justify separate names because, although they are all manifestations of the same disease process, each separately named tumour has its own characteristics in terms of cause, appearance and behaviour. Accurate diagnosis and naming of tumours is essential so that patients can be optimally treated. A tumour that defies accurate classification is designated anaplastic; such tumours are always malignant.
The specific name of an individual tumour invariably ends in the suffix ‘-oma’. However, relics of this suffix’s former wider usage remain, as in ‘granuloma’ (an inflammatory aggregate of epithelioid macrophages), ‘tuberculoma’ (the large fibrocaseating lesion of tuberculosis), ‘atheroma’ (lipid-rich intimal deposits in arteries), and ‘mycetoma’ (a fungal mass populating a lung cavity) and ‘haematoma’ (mass of coagulated blood); these are not neoplasms.
There are exceptions to the rules of nomenclature that follow and these are a potential source of misunderstanding. For example, the words ‘melanoma’ and ‘lymphoma’ are both commonly used to refer to malignant tumours of melanocytes and lymphoid cells respectively, even though, from the rules of tumour nomenclature, these terms can be mistakenly interpreted as meaning benign lesions. To avoid confusion, which could be clinically disastrous, their names are often preceded by the word ‘malignant’. Similarly, a ‘myeloma’ is a malignant neoplasm of plasma cells.
The suffix for neoplastic disorders of blood cells is ‘-aemia’, as in leukaemia; but again, exceptions exist. For example, anaemia is not a neoplastic disorder.
Detailed descriptions of individual tumours are, in most instances, included in the relevant systematic chapters. Examples of tumour nomenclature are given below and, for reference, in Table 11.3.
Table 11.3 Examples of tumour nomenclature
Type | Benign | Malignant |
---|---|---|
Epithelial | ||
Squamous cell | Squamous cell papilloma | Squamous cell carcinoma |
Transitional | Transitional cell papilloma | Transitional cell carcinoma |
Basal cell | Basal cell papilloma | Basal cell carcinoma |
Glandular | Adenoma (e.g. thyroid adenoma) | Adenocarcinoma (e.g. adenocarcinoma of breast) |
Mesenchymal | ||
Smooth muscle | Leiomyoma | Leiomyosarcoma |
Striated muscle | Rhabdomyoma | Rhabdomyosarcoma |
Adipose tissue | Lipoma | Liposarcoma |
Blood vessels | Angioma | Angiosarcoma |
Bone | Osteoma | Osteosarcoma |
Cartilage | Chondroma | Chondrosarcoma |
Mesothelium | Benign mesothelioma | Malignant mesothelioma |
Synovium | Synovioma | Synovial sarcoma |
Epithelial tumours
Epithelial tumours are named histogenetically according to their specific epithelial type and behaviourally as benign or malignant.
Benign epithelial tumours
Benign epithelial tumours are either:
A papilloma is a benign tumour of non-glandular or non-secretory epithelium, such as transitional or stratified squamous epithelium (Fig. 11.12). An adenoma is a benign tumour of glandular or secretory epithelium (Fig. 11.13). The name of a papilloma or adenoma is incomplete unless prefixed by the name of the specific epithelial cell type or glandular origin; examples include squamous cell papilloma, transitional cell papilloma, colonic adenoma and thyroid adenoma.
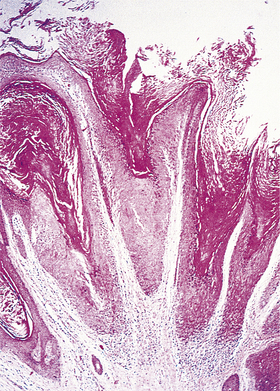
Fig. 11.12 Histology of a benign tumour of non-secretory epithelium: squamous cell papilloma. The tumour is non-invasive and grows outwards from the skin surface (i.e. it is exophytic). The tumour cells closely resemble those of the normal epidermis. This benign tumour is commonly caused by a human papillomavirus.
Malignant epithelial tumours
Malignant tumours of epithelium are always called carcinomas. Carcinomas of non-glandular epithelium are always prefixed by the name of the epithelial cell type; examples include squamous cell carcinoma and transitional cell carcinoma. Malignant tumours of glandular epithelium are always designated adenocarcinomas, coupled with the name of the tissue of origin; examples include adenocarcinoma of the breast, adenocarcinoma of the prostate and adenocarcinoma of the stomach.
Carcinomas should be further categorised according to their degree of differentiation: their resemblance to the tissue of origin.
Carcinoma in situ
The term carcinoma in situ refers to an epithelial neoplasm exhibiting all the cellular features associated with malignancy, but which has not yet invaded through the epithelial basement membrane separating it from potential routes of metastasis—blood vessels and lymphatics (Fig. 11.14). Complete excision at this very early stage will guarantee a cure. Detection of carcinomas at the in situ stage, or of their precursor lesions, is the aim of population screening programmes for cervical, breast and some other carcinomas. The phase of in situ growth may last for several years before invasion commences.
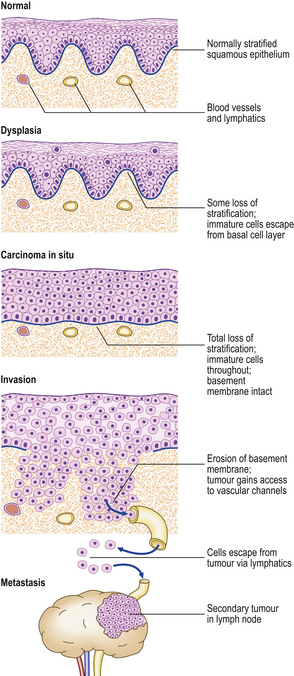
Fig. 11.14 Evolution of an invasive squamous cell carcinomafrom the precursor lesions of dysplasia and carcinoma in situ (usually grouped together as intra-epithelial neoplasia). Note that the tumour cells cannot reach routes of metastasis such as blood vessels and lymphatics until the basement membrane has been breached.
Carcinoma in situ may be preceded by a phase of dysplasia, in which the epithelium shows disordered maturation short of frank neoplasia. Some dysplastic lesions are almost certainly reversible. As there are other applications of the word ‘dysplasia’, as well as some difficulty in reliably distinguishing between carcinoma in situ and dysplasia in biopsies, the term is now less favoured. The term ‘intra-epithelial neoplasia’, as in cervical intra-epithelial neoplasia (CIN), is used increasingly to encompass both carcinoma in situ and the precursor lesions formerly known as dysplasia.
Connective tissue and other mesenchymal tumours
Tumours of connective and other mesenchymal tissues are, like epithelial tumours, named according to their cell of origin and their behavioural classification.
Benign connective tissue and mesenchymal tumours
Benign mesenchymal tumours are named after the cell or tissue of origin suffixed by ‘-oma’, as follows:
Malignant connective tissue and mesenchymal tumours
Malignant tumours of mesenchyme are always designated sarcomas, prefixed by the name that describes the cell or tissue of origin. Examples include:
As with carcinomas, sarcomas can be further categorised according to their grade or degree of differentiation (Fig. 11.15).
Eponymously named tumours
Some tumours have inherited the name of the person who first recognised or described the lesion. Examples include:
Miscellaneous tumours
Most tumours can be categorised according to the scheme of nomenclature already described. There are, however, important exceptions.
Teratomas
A teratoma is a neoplasm formed of cells representing all three germ cell layers: ectoderm, mesoderm and endoderm. In their benign form, these cellular types are often easily recognised; the tumour may contain teeth and hair, and, on histology, respiratory epithelium, cartilage, muscle, neural tissue, etc. In their malignant form, these representatives of ectoderm, mesoderm and endoderm will be less easily identifiable.
Teratomas are of germ cell origin. They occur most often in the gonads, where germ cells are abundant. Although all cells in the body contain the same genetic information, arguably in germ cells this information is in the least repressed state and is therefore capable of programming such divergent lines of differentiation. Supporting evidence for a germ cell origin for teratomas comes from karyotypic analysis of their sex chromosome content. Teratomas in the female are always XX, whereas only 50% of those in the male are XX and the remainder XY; this correlates with the sex chromosome distribution in the germ cells of the two sexes.
Ovarian teratomas are almost always benign and cystic; in the testis, they are almost always malignant and relatively solid. As germ cells in the embryo originate at a site remote from the developing gonads, teratomas arise occasionally elsewhere in the body, usually in the midline, possibly from germ cells that have been arrested in their migration. These extragonadal sites for teratomas include the mediastinum and sacro-coccygeal region.
Embryonal tumours: the ‘blastomas’
Some types of tumour occur almost exclusively in the very young, usually in those below 5 years of age, and bear a histological resemblance to the embryonic form of the organ in which they arise. Examples include:
Mixed tumours
Mixed tumours show a characteristic combination of cell types. The best example is the mixed parotid tumour (pleomorphic salivary adenoma); this consists of glands embedded in a cartilaginous or mucinous matrix derived from the myoepithelial cells of the gland. Another common mixed tumour is the fibroadenoma of the breast, a lobular tumour consisting of epithelium-lined glands or clefts in a loose fibrous tissue matrix.
The occurrence of mixed tumours in an individual organ can sometimes be predicted from its embryology. This is illustrated by the Müllerian tract tumours that occur in the female genital tract; these often contain a mixture of carcinomatous and sarcomatous elements reflecting the intrinsic capacity of the tissue for divergent differentiation.
A tumour may also have a mixed appearance because of metaplasia within it. For example, transitional cell carcinomas of the bladder sometimes have foci of glandular or squamous differentiation.
Carcinosarcomas combine the appearances of carcinoma and sarcoma in one tumour, as a result of either collision of adjacent carcinoma and sarcoma or divergent carcinomatous and sarcomatous differentiation from one original transformed cell.
Neuroendocrine tumours
Neuroendocrine tumours are derived from peptide hormone-secreting cells scattered diffusely in various epithelial tissues. These cells are sometimes referred to as APUD cells; this acronym signifies their biochemical properties (amine content and/or precursor uptake and decarboxylation). (For this reason, the tumours have been called ‘apudomas’.)
The name of those neuroendocrine tumours producing a specific peptide hormone is usually derived from the name of the hormone, together with the suffix ‘-oma’. For example, the insulin-producing tumour originating from the beta-cells of the islets of Langerhans is called an insulinoma. There are exceptions: for example, the calcitonin-producing tumour of the thyroid gland is called a ‘medullary carcinoma of the thyroid gland’ because it was described as a specific entity before calcitonin had been discovered.
Neuroendocrine tumours of the gut and respiratory tract that do not produce any known peptide hormone are called carcinoid tumours. The appendix is the commonest site, but, here, these tumours are usually an incidental finding of little clinical significance. Carcinoids arising elsewhere (the small bowel is the next commonest site) often metastasise to mesenteric lymph nodes and the liver. Extensive metastases lead to the carcinoid syndrome (tachycardia, sweating, skin flushing, anxiety and diarrhoea) due to excessive production of 5-hydroxytryptamine and prostaglandins.
Many neuroendocrine tumours are functionally active, and clinical syndromes often result from excessive secretion of their products (Table 11.4).
Table 11.4 Some neuroendocrine tumours and their associated clinical syndromes
Tumour | Clinical syndrome |
---|---|
Insulinoma | Episodes of hypoglycaemia |
Gastrinoma | Extensive peptic ulceration of the upper gut (Zollinger–Ellison syndrome) |
Phaeochromocytoma | Paroxysmal hypertension |
Carcinoid | If metastases are present, flushing, palpitations and pulmonary valve stenosis |
These neoplasms often pursue an indolent course, growing relatively slowly and metastasising late. Their behaviour cannot always be predicted from their histological features.
Some individuals inherit a familial predisposition to develop neuroendocrine tumours; they have a multiple endocrine neoplasia (MEN) syndrome (Ch. 17).
Hamartomas
A hamartoma is a tumour-like lesion, the growth of which is co-ordinated with the individual; it lacks the autonomy of a true neoplasm. Hamartomas are always benign and usually consist of two or more mature cell types normally found in the organ in which the lesion arises. A common example occurs in the lung, where a hamartoma typically consists of a mixture of cartilage and bronchial-type epithelium (the so-called ‘adenochondroma’; Ch. 14). Pigmented naevi or ‘moles’ (Ch. 24) may also be considered as hamartomatous lesions. Their clinical importance is:
Cysts
A cyst is a fluid-filled space lined by epithelium. Cysts are not necessarily tumours or neoplasms but, because they may have local effects similar to those produced by true tumours and some tumours are typically cystic, it is pertinent to consider them here. Common types of cyst are:
The only type of cyst whose aetiology merits its inclusion within this chapter is the neoplastic cyst. This is seen most commonly in the ovary, where it may be either a benign cystic teratoma, filled with sebaceous material, or a cystadenoma or cystadenocarcinoma, each of which may be filled with either serous fluid or mucus depending on the secretory properties of the lining epithelium.
BIOLOGY OF TUMOUR CELLS
Contrary to past claims and an enduring hope, there is no therapeutically exploitable feature unique to neoplastic cells other than the general property of relative or absolute growth autonomy. Many of the other features have normal counterparts: mitotic activity is a feature also of regenerating cells; placental trophoblast is invasive; and the nucleated cells of the blood and lymph wander freely around the body, settling in other sites.
The autonomy of neoplastic cells is often relative rather than absolute. For example, approximately two-thirds of breast carcinomas contain oestrogen receptors; these tumours are better differentiated than receptor-negative breast carcinomas and they have a better prognosis. Furthermore, if women with oestrogen receptor-positive breast carcinomas are given tamoxifen (a drug that blocks the receptor) they survive longer than women with receptor-positive tumours who have not been treated in this way.
One of the many difficulties in studying tumours is their genetic instability, leading to the formation of many clones with divergent properties within one tumour. This is often reflected in the histology which may show a heterogeneous growth pattern, some areas appearing better differentiated than others. Clinically, this instability and consequent cellular heterogeneity is important because thereby some tumours resist chemotherapy; consequently, many chemotherapy regimes involve a combination of agents administered simultaneously or sequentially.
Cellular immortalisation
Cells that have undergone neoplastic transformation appear immortal, especially when studied in cell cultures. Whereas normal untransformed cells have a limited life-span, neoplastic cells have a prolonged or indefinite life-span. This is enabled by:
DNA of tumour cells
Tumour cells have abnormal nuclear DNA. The total amount of DNA per cell commonly exceeds that of the normal diploid (2N) population. This is evident in histological sections as nuclear hyperchromasia. The amount of DNA may increase in exact multiples of the diploid state (polyploidy) such as tetraploid (4N) and octaploid (8N); alternatively there may be aneuploidy—the presence of inexact multiples of DNA per cell.
Aneuploidy and polyploidy are associated with increased tumour aggressiveness and are recognisable in histological sections as variations in nuclear size and staining (pleomorphism).
At a chromosomal level these abnormalities of DNA are associated with the presence of additional chromosomes and often with chromosomal translocations. Some of these karyotypic abnormalities have a regular association with specific tumours; the best known and one of the most consistent is the association of the Philadelphia chromosome with chronic myeloid leukaemia.
Genetic abnormalities are being discovered with increasing frequency in tumours. Some of these may be relatively late events, epiphenomena with no central role in the cancer process. However, others are of fundamental importance, appearing at an early stage in the development of the tumour. Abnormalities of oncogenes and tumour suppressor genes are of considerable interest in this regard because of their central involvement in carcinogenesis (p. 246).
Mitotic and apoptotic activity
Malignant tumours frequently exhibit more mitotic activity than the corresponding normal cell population. In histological sections, mitoses are abundant, and mitotic figures are often grossly abnormal, showing tripolar and other bizarre arrangements. Cellular proliferation can be estimated by mitosis counting, DNA measurements and determination of the frequency of expression of cell cycle-associated proteins (e.g. Ki-67 antigen). Prognostic information can be derived from these estimations: higher frequencies of cellular proliferation are associated with a worse prognosis.
However, assessment of the growth characteristics of a tumour must involve also an appraisal of the rate of cell loss, through either ischaemic necrosis or apoptotic cell death. Although tumours often contain abundant apoptotic bodies, a common biological defect of neoplastic cells is abrogation of the cell death mechanisms. In some lymphomas, for example, this is mediated by abnormal expression of bcl-2, an apoptosis-inhibiting gene.
Metabolic abnormalities
Although tumour cells show a tendency towards anaerobic glycolysis, there are no metabolic abnormalities entirely specific to the neoplastic process. The known metabolic abnormalities of tumour cells are simply discordant with the normal physiological state of the tissue or host.
The surface of tumour cells is abnormal. Tumour cells are less cohesive. In many neoplasms, poor cellular cohesion is due to a reduction in specialised intercellular junctions such as desmosomes. This loss of adhesiveness enables malignant tumour cells to spread through tissues and detach themselves to populate distant organs.
Tumour cells may retain the capacity to synthesise and secrete products characteristic of the normal cell type from which they are derived, often doing so in an excessive and uncontrolled manner. In addition, tumours often show evidence of gene derepression. All somatic cells contain the same genetic information, but only a small proportion of the genome is transcribed into RNA and translated into protein in any normal cell. Most genes are repressed, and only those required for the function of the particular cell are selectively expressed. However, in many tumour cells, some genes become derepressed, resulting in the inappropriate synthesis of unexpected substances.
Tumour products
The major types of tumour product are:
Some tumour products are useful as markers for diagnosis or follow-up (Table 11.5). They can be detected in histological sections or their concentrations measured in the blood. Rising blood levels suggest the presence of tumour; falling levels indicate a sustained response to therapy (Fig. 11.16).
Table 11.5 Tumours secreting markers used in diagnosis or follow-up
Tumour | Marker | Comment |
---|---|---|
Myeloma |
Hepatocellular carcinomaAlpha-fetoprotein (AFP)Also associated with testicular teratomaGastrointestinal adenocarcinomasCarcinoembryonic antigen (CEA)False positives occur in some non-neoplastic conditionsNeuroendocrine tumoursPeptide hormones (e.g. insulin, gastrin)Excessive hormone production may have clinical effectsPhaeochromocytomaVanillyl mandelic acid (VMA)Metabolite of catecholamines in urineCarcinoid5-Hydroxyindole-acetic acid (5-HIAA)Metabolite of 5-hydroxytryptamine (5-HT) in urineChoriocarcinomaHuman chorionic gonadotrophin (hCG)In blood or urineMalignant teratoma