Chapter 23 Blood and bone marrow
COMMON CLINICAL PROBLEMS FROM BLOOD AND BONE MARROW DISEASE
Pathological basis of haematological signs and symptoms
Sign or symptom | Pathological basis |
---|---|
Tiredness, dyspnoea | Reduced oxygen-carrying capacity of blood due to anaemia |
Mucosal pallor | Anaemia |
Glossitis (sore mouth, smooth tongue) | Mucosal effects of haematinic deficiency |
Spoon-shaped nails | Due to iron deficiency |
Jaundice | Bilirubin accumulation from haemolysis |
Abnormal tendencyto infections | Neutropenia, e.g. in leukaemia or hypoplastic anaemiaImmune deficiency, e.g. in myeloma, and due to chemotherapy in leukaemia and lymphoma |
Splenomegaly | Due to expansion of haemopoiesis in myeloproliferative disorders, red cell pooling and destruction in haemolytic anaemias, infiltration in leukaemias and lymphomas |
Also non-haematological causes, e.g. portal hypertension | |
Lymphadenopathy | Non-neoplastic causes, e.g. infectious mononucleosisInfiltration with leukaemia or lymphoma |
Bone pain and fractures | Osteoclast activation in myeloma |
Purpura, bruising,mucosal or traumaticbleeding | Thrombocytopenia or platelet dysfunction |
Bruising, muscle and joint bleeding, andtraumatic bleeding | Coagulation factor deficiency |
COMPOSITION, PRODUCTION AND FUNCTIONS OF BLOOD
Blood is a unique organ: it is fluid and comes into contact with almost all other tissues. The blood cells are non-cohesive and supported in the fluid medium of blood—the plasma. The blood cells comprise the non-nucleated erythrocytes and platelets, and the nucleated cells or leukocytes.
In addition to primary disease of the blood-forming organ—the bone marrow—many disease states produce secondary changes in the blood. For this reason, the counting and morphological examination of blood cells is routine in the clinical assessment of disease, frequently providing valuable diagnostic information.
CELLULAR COMPONENTS
The peripheral blood is investigated by microscopy of a droplet spread evenly over the surface of a glass slide—the blood film. Routinely, the blood film is treated with a combination of stains which allow identification of nuclear and cytoplasmic detail (Fig. 23.1).
Quantitation of blood cells is essential; in modern laboratories this is routinely performed by automated cell-counting equipment. The size and concentration of erythrocytes, and the leukocyte and platelet concentrations are measured. Haemoglobin is automatically measured. Also, the proportion of leukocytes of each category—the differential white cell count—is measured from cell size and granule content.
Erythrocytes
Erythrocytes (red blood cells) are deformable, non-nucleated and biconcave discs (Fig. 23.2). They are the most abundant blood cell. When blood is separated, by centrifugation, into cellular and plasma components, the red cell portion is approximately 45% of the total volume: this is the ‘packed cell volume’ or haematocrit.
The erythrocyte is a special oxygen-carrying cell because it is rich in haemoglobin. The cell membrane is composed of a phospholipid bilayer with integral proteins. The shape of the cell is maintained by structural proteins, such as spectrin, which form a cytoskeleton. Enzyme systems protect the haemoglobin from irreversible oxidation. The mature erythrocyte has no nuclear material, so new protein cannot be synthesised. The mature erythrocyte circulates for around 120 days before it is removed by the reticulo-endothelial system.
Absolute values
The absolute values are measures of red cell size and haemoglobin content which provide valuable information in the assessment of anaemia, as they provide diagnostic clues as to the likely cause. Absolute values are calculated from the red cell concentration, haemoglobin concentration and haematocrit as follows:
In the modern laboratory, automated cell counters provide these data on each blood sample analysed.
Morphology
The biconcave erythrocyte shape provides a large surface area for oxygen diffusion. By light microscopy erythrocytes appear as uniform round cells with central pallor. Up to 1% of cells stain with a purplish tinge and are of rather greater diameter. These are polychromatic cells; this purple staining is due to the residual ribonucleic acid (RNA) of the immature erythrocyte. These young cells become indistinguishable from the mature red cell population after 48 hours in the blood. When stained with a supravital stain (such as methylene blue) polychromatic cells are more easily identified by the presence of characteristic inclusions; they are then termed reticulocytes. The inclusions are remnants of RNA. When bone marrow production of erythrocytes is increased, the proportion of polychromatic cells, or reticulocytes, in the peripheral blood becomes greater than 1% or 100 × 109/l. This occurs most commonly in recovery from acute haemorrhage or when there is an increased rate of destruction of red cells, which is called haemolytic anaemia. Failure to produce a reticulocyte response to anaemia suggests that the patient has bone marrow failure or haematinic deficiency.
Changes in disease
Anaemia is present when the haemoglobin concentration is less than approximately 130g/l in a male or 115g/l in a female (Table 23.1); the haematocrit is also reduced. Conversely, polycythaemia describes an increased red cell concentration; it is usually accompanied by a raised haemoglobin concentration and haematocrit.
Anaemias may be simply classified according to red cell size (MCV) and haemoglobin content (MCH). This classification is of great diagnostic value in most common types of anaemia (Table 23.2). Further diagnostic information is obtained by the microscopic examination of the red cell morphology on a blood smear. Disease of the blood is frequently associated with increased variation in red cell size—anisocytosis—and the presence of erythrocytes of abnormal shape—poikilocytosis (Fig. 23.3). Increased erythrocyte anisocytosis and poikilocytosis are non-specific abnormalities present in many haematological and systemic disorders. An example is the marked aniso-poikilocytosis that occurs in the absence of a functioning spleen, due to surgical removal or secondary to disease. In this situation there are also inclusions in red cells. They are called Howell–Jolly bodies (Fig. 23.4) and are remnants of nuclear material that would normally be removed when newly formed erythrocytes released from bone marrow circulate for the first time through the spleen.
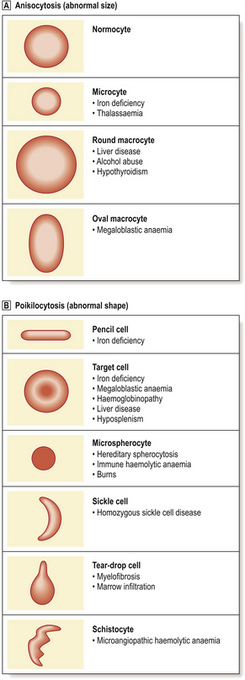
Fig. 23.3 Abnormalities of red cell size and shape. Increased variation in size: anisocytosis.
Variation in shape: poikilocytosis.
In addition to Howell–Jolly bodies, red cells may contain other inclusions (Fig. 23.4) under certain circumstances. The basophilic stippling of the ‘stipple cell’ is due to the presence of residual RNA; stipple cells may be present in several anaemias, especially thalassaemia. Siderotic granules contain iron and may occur in states of iron overload, for example in chronically anaemic subjects who have received treatment by frequent transfusion of red cells. Occasionally, nucleated red cell precursors may escape into the peripheral blood; when these normoblasts are accompanied by immature neutrophil leukocytes the film is described as leukoerythroblastic. A leukoerythroblastic blood film results from gross marrow disturbance such as infiltration by malignancy, or fibrous tissue (myelofibrosis) or in severe anaemia due to deficiency of vitamin B12 or folate (megaloblastic anaemia).
Supravital staining is used to detect the presence of reticulocytes, as described above. This technique also identifies another type of red cell inclusion—Heinz bodies. These inclusions represent denatured haemoglobin and are seen typically in certain haemolytic anaemias, especially those due to a deficiency in the protective enzyme systems such as glucose-6-phosphate dehydrogenase deficiency.
Leukocytes
The nucleated cells of the peripheral blood are termed white blood cells or leukocytes. Their primary role is protection against infection or infestation of the body. Morphologically, on a stained blood film, five varieties of leukocyte are identified.
The normal concentrations of these are:
These are typical values for healthy adults and older children. The normal counts differ in infants, who have a higher proportion of lymphocytes, for example.
Also, it is important to appreciate how such laboratory normal ranges are established in order to avoid misinterpretation. Cell counts are performed on a large number of healthy subjects and the range is determined from the population mean and two standard deviations above and below the mean. This dictates that, for a particular measurement, 2.5% of healthy subjects have a count just below the lower limit of ‘normal’ and a further 2.5% just above.
The granulocytes and monocytes are phagocytic leukocytes produced from precursor cells in the bone marrow. The lymphocytes are broadly composed of B-cells which mediate humoral immunity via the maturation to immunoglobulin-producing plasma cells; they are produced initially in the bone marrow and subsequently mature by antigen selection in the germinal centres of secondary lymphoid tissues; and T-cells which provide cell-mediated immunity such as killing virally infected cells. T-cells are produced and selected for antigen in the thymus gland. B- and T-cells circulate in the blood as small lymphocytes.
Neutrophil granulocytes
Neutrophils are the most numerous leukocytes in the blood of the healthy adult. The nucleus of the neutrophil granulocyte is characteristically segmented into up to five lobes and the nuclear chromatin stains densely (Fig. 23.1). The abundant cytoplasm stains pink and contains characteristic granules. Within the granules are enzymes, including myeloperoxidase, alkaline phosphatase and lysozyme. Neutrophils have a scavenging function and are of particular importance in defence against bacterial infection.
Neutrophil precursors and neutrophils spend 14 days in the bone marrow, whereas the half-life of neutrophils in the blood is only 6–9 hours. Peripheral blood counts therefore measure less than 10% of the total body neutrophils. Within the circulation the cells move between a circulating and a ‘marginating’ pool, margination being attachment to vascular endothelial cells. To perform their scavenging function, granulocytes irreversibly enter the tissues by penetrating endothelial cells modified by inflammatory mediators. Cytokine-stimulated endothelial cells present adhesion molecules which interact with neutrophils and facilitate their passage: one such is ICAM-1 (intercellular adhesion molecule 1).
Lymphocytes
The peripheral blood lymphocytes are small leukocytes with a round or only slightly indented nucleus and scanty sky-blue-staining cytoplasm which may contain an occasional pink- or red-staining granule. Circulating B- and T-cells are not distinguishable by morphology alone. Immunological staining shows that in health approximately 70% of circulating small lymphocytes are T-cells and 30% B-cells.
A small proportion of lymphocytes may be larger with abundant cytoplasm, sometimes referred to as ‘activated’ lymphocytes. These are believed to represent cells that have been stimulated, perhaps by foreign antigen. A more complete description of the classification and role of lymphocytes is to be found in Chapter 9.
Monocytes
Monocytes are the largest blood cells. The nucleus is oval or reniform but not lobed. The abundant cytoplasm stains pale blue and often contains pink granules; vacuoles are often present. The function of monocytes is similar to that of neutrophil granulocytes: they enter the tissues and, as tissue macrophages, are responsible for the phagocytosis and digestion of foreign material and dead tissue.
Eosinophil granulocytes
Eosinophil granulocytes have much larger red-staining granules. They contain enzymes, including a peroxidase. The nucleus is lobulated, but usually only two or three lobes are seen. The eosinophil is important in the mediation of the allergic response and in defence against parasitic infestation.
Basophil granulocytes
Basophil granulocytes are the least frequent leukocytes in normal blood. The granules are large, blue–black and obscure the bilobed nucleus; they contain heparin and histamine. Basophils are closely related to tissue mast cells but their function has not been determined precisely. They appear to be key mediators of immediate hypersensitivity reactions, involving release of histamine.
Changes in disease
Changes may be quantitative or qualitative; the former are more important and often of diagnostic value. Knowledge of the causes of increased numbers of the various leukocytes in the peripheral blood is useful clinically.
Quantitative changes
Leukocytosis means an increase in numbers of circulating white blood cells. Depending on the cause, there may be a polymorphonuclear leukocytosis (neutrophilia—increased neutrophil leukocytes), monocytosis, eosinophil leukocytosis (eosinophilia), basophil leukocytosis (basophilia) or lymphocytosis.
Causes of reactive neutrophil leukocytosis include:
Monocytosis may be reactive to:
Eosinophil leukocytosis may be reactive to:
Lymphocytosis is most commonly associated with an infection such as infectious mononucleosis, tuberculosis, etc.
In some disorders the leukocytosis may be extreme (for example 100 × 109/l), particularly in children. There may also be a tendency for immature leukocytes, particularly myelocytes and metamyelocytes, to appear in the peripheral blood. Severe bacterial infection may result in such an extreme reactive picture, which has in the past been referred to as a ‘leukaemoid reaction’ because of the similarity of the blood picture, with immature forms present, to that of chronic myeloid leukaemia. Occasionally, the lymphocyte series may be involved in such an extreme reactive process, especially during childhood viral infection.
A characteristic leukocytosis composed of ‘atypical’ lymphocytes is a feature of infectious mononucleosis (glandular fever). The infection is common in young adults and often manifests as a sore throat with enlarged lymph nodes and spleen and skin rash. It is due to infection with Epstein–Barr (EB) virus and is common between 15 and 25 years of age in developed countries, but occurs in young children in heavily populated developing countries. The major additional features are:
The atypical cells in peripheral blood are recognisable as lymphocytes but are much larger and have abundant cytoplasm and nuclear irregularities (Fig. 23.5). They are probably reactive T-lymphocytes responding to B-lymphocytes containing the virus, are detectable in blood about 7 days after the onset of illness and may persist for 6 weeks or more. Apparently fortuitously, but usefully, antibodies reactive against horse, sheep and ox red cells (heterophile antibodies) typically develop during the second week and may persist for a few months; they are detected in the Paul–Bunnell test or by more convenient commercial screening slide tests such as the ‘Monospot’ test, and are of diagnostic value. A very similar clinical and haematological (but not serological) picture can develop as a result of other infections, especially with human immunodeficiency virus (HIV), cytomegalovirus and toxoplasma.
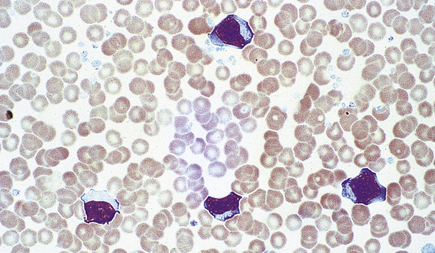
Fig. 23.5 Atypical mononuclear cells in infectious mononucleosis. These large T-lymphocytes have copious basophilic cytoplasm with irregular cell outline.
All of the above are examples of reactive leukocytosis. Increased white cell counts in peripheral blood, often with immature forms present, are also a typical feature of some primary disorders of the bone marrow, especially leukaemias and myeloproliferative disorders.
A reduction in circulating leukocytes is termed leukopenia. Most important is a deficiency of neutrophil granulocytes— neutropenia. Neutropenia is commonly seen in association with a reduction in other blood cells, that is, as part of a pancytopenia. Important causes of pancytopenia are:
Important causes of selective neutropenia are:
In cyclical forms the neutropenia is temporary and recurrent, often with a periodicity of 3–4 weeks. It is an uncommon condition.
Neutropenia with counts of less than 0.5 × 109/l may result in severe sepsis, especially of the mouth, pharynx (Fig. 23.6) and peri-anal regions, and also in disseminated infection. This clinical picture is now most commonly seen in patients receiving drug or irradiation therapy for malignant disorders.
Qualitative changes
Qualitative leukocyte changes are less important than quantitative abnormalities. Defects of phagocytic cell function resulting in an increased tendency to bacterial infection are recognised, particularly as acquired defects after splenectomy, in leukaemic disorders and due to corticosteroid therapy. Congenital abnormalities of leukocyte function are uncommon. ‘Atypical’ lymphocytes in infectious mononucleosis have been described earlier. Other abnormalities of neutrophil morphology are also recognised (Fig. 23.7).
Deficiency of lymphocytes in blood is termed lymphopenia. It is often due to medication with immunosuppressive or cytotoxic drugs, for example. Lymphopenia is an important feature of infection with HIV.
Platelets
On a stained blood film platelets appear as non-nucleated fragments of granular cytoplasm, approximately one-fifth the diameter of erythrocytes and in a concentration of 150–400 × 109/l. Platelets are contractile and adhesive cells, the function of which is the maintenance of vascular integrity. Exposure of vascular subendothelial structures results in rapid adhesion of platelets to the exposed area and aggregation of platelets to each other in the formation of a primary haemostatic plug (Fig. 23.8). Platelets are rich in intracellular granules, which are released during stimulation. The most abundant granules, alpha granules, contain proteins and peptides, including von Willebrand factor, some coagulation factors and growth factors. Platelets deliver these to sites of vascular injury, where they contribute to clot formation and the repair process. Dense bodies are less abundant platelet granules and are rich in calcium, serotonin and adenine nucleotides.
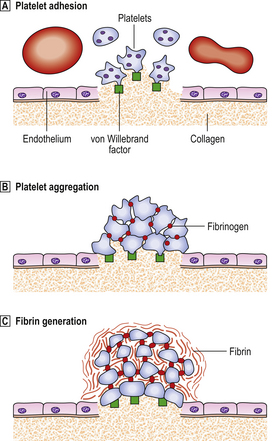
Fig. 23.8 The physiology of primary haemostasis. Platelet adhesion. Exposure of subendothelial material causes activation of platelets, which change shape and produce pseudopodia, and rapidly adhere to the area via receptor sites which interact with von Willebrand factor (vWF). vWF is a multimeric protein synthesised by endothelial cells and megakaryocytes. It associates with a coagulation factor, factor VIII, in plasma.
Platelet aggregation. Platelets interact with each other via receptor sites which use fibrinogen as an intercellular bridge. Platelets contract and release granules which contain pro-aggregatory substances that promote the aggregation response. These include ADP, 5-hydroxytryptamine, fibrinogen and vWF. Metabolism of arachidonic acid, a fatty acid of the cell membrane, to the prostaglandin-like metabolite thromboxane A2 also promotes aggregation and, in addition, vasoconstriction.
Fibrin generation. Exposure of tissue factor activates the extrinsic coagulation system. Thrombin generation augments the platelet activation and activated platelets provide phospholipid, which is an essential co-factor at several points in the coagulation cascade.
A deficiency of blood platelets is termed thrombocytopenia, the causes and consequences of which are described on page 670. Thrombocytosis, or increased platelet numbers, may be due to a primary bone marrow problem or may be reactive. Examples of reactive thrombocytosis are seen in:•
Thrombocytosis may also occur in primary disorders of bone marrow—the myeloproliferative diseases and chronic myeloid leukaemia. Morphological platelet abnormalities are of minor importance, although ‘giant’ platelets, with a diameter exceeding that of an erythrocyte, are a feature of the myeloproliferative disorders rather than reactive thrombocytosis. Giant platelets are also a feature of some inherited syndromes, including those associated with mutations of the myosin heavy chain gene.
Blood count and morphology in disease
Changes in the blood are present in a wide range of diseases of other organs. These changes are most commonly reactive or secondary but may be useful in providing a clue to the presence and type of underlying disease, e.g. polymorphonuclear leukocytosis in bacterial sepsis; eosinophilia in some parasitic infections. In other cases the abnormalities of cell number and morphology are due to a primary haematological disorder.
BLOOD PLASMA
Plasma amounts to greater than 50% of blood volume. While changes in the innumerable constituents of plasma are outside the scope of this text, consideration of certain major plasma proteins is necessary for an understanding of the pathology of some blood and systemic disorders. The plasma proteins that are components of the blood coagulation and fibrinolytic systems are considered first.
Blood coagulation
For normal homeostasis, blood must be fluid; however, the capacity to minimise loss of blood through breaches of the vascular system is essential. The rapid plugging of defects in small vessels is the function of platelets (primary haemostasis, Fig. 23.8) but a more permanent and secure seal results from the generation of insoluble fibrillar fibrin from its soluble plasma protein precursor fibrinogen in the process of blood coagulation. Failure of primary haemostasis, due to platelet disorders, or of coagulation due to clotting factor deficiency or presence of a coagulation inhibitor, can each result in life-threatening haemorrhage. In contrast, inappropriate activation of platelets or blood coagulation may result in vascular occlusion, ischaemia and tissue death. A complex system of activators and inhibitors in plasma has therefore evolved in order to allow localised clot formation at sites of injury but to minimise the risk of inappropriate and undesirable clotting, i.e. thrombosis. These are the coagulation and fibrinolytic factors and their inhibitors (Fig. 23.9).
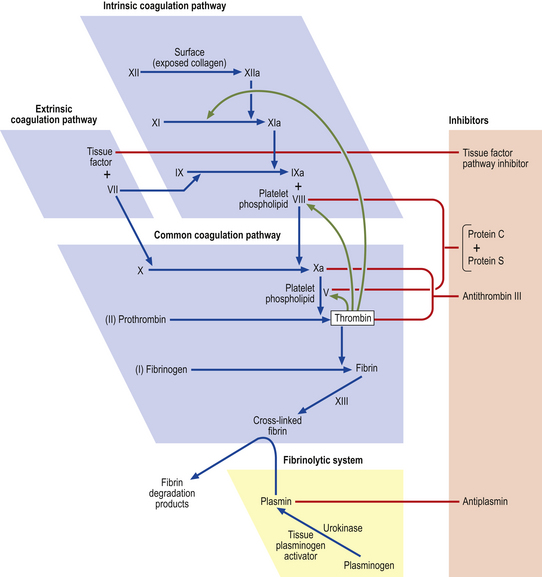
Fig. 23.9 The coagulation and fibrinolytic systems. Activators (blue boxes). These are enzyme precursors or co-factors. The nomenclature uses Roman numerals, the suffix ‘a’ designating the active enzyme. Ca2+ is required at several points in the cascade, as is phospholipid surface provided by activated platelets. Inhibitors. These have an anticoagulant action. Fibrinolytic system. This leads to plasmin generation. Plasmin has its own principal inhibitor, antiplasmin.
Important features of the haemostatic mechanism are as follows.
Coagulation inhibitors limit unwanted clotting and protect against vessel occlusion, especially in veins:
Although the scheme for the initial stages of coagulation activation can be conveniently divided into extrinsic and intrinsic pathways (Fig. 23.9), this is a simplification. Coagulation activation in vivo is initiated through tissue factor, an integral cell membrane protein which is not expressed by vascular endothelial cells in an unstimulated state but is expressed by subendothelial cells and smooth muscle as well as other cells. As soon as blood leaks from a vessel it is exposed to tissue factor. Tissue factor activates factor VII. The much slower pathway for fibrin generation through activation of factor XII on contact with subendothelial components is of minor importance. This partially explains the absence of any increased tendency to haemorrhage in subjects who are congenitally deficient in factor XII. It is the tissue factor–activated factor VII complex that rapidly activates factors X and IX, leading to thrombin generation. When the procoagulant stimulus is sufficiently strong, the degree of amplification through thrombin activation of factors V and VIII overcomes inhibition by activated protein C and fibrin generation proceeds.
The final step in clot formation is the stabilisation of fibrin by cross-linking through the activity of factor XIII.
In the laboratory, the function of the components of the coagulation system can be assessed by the time required for clotting of recalcified plasma prepared from a blood sample anticoagulated with sodium citrate. The citrate binds calcium ions, which are required at several points in the mechanism. Recalcification allows fibrin formation to take place. The two principal screening tests used in clinical practice are:
The pathology and consequences of deficiency of the components of the coagulation and fibrinolytic system are described on page 673.
Rheological considerations
Blood is a viscous fluid and changes in its physical properties accompany some diseases. The major determinant of blood viscosity is the haematocrit.
The plasma fibrinogen concentration is the major determinant of red cell aggregation and is second only to haematocrit as a factor in determination of blood viscosity. Other plasma protein molecules tend to be smaller and more symmetrical than fibrinogen and consequently have a much lesser effect on viscosity. However, when they are present in increased concentrations, blood viscosity may be affected. This may result in a hyperviscosity syndrome, in which there is stasis within the microcirculation and tissue anoxia. Cerebral dysfunction, with headache, visual disturbance and drowsiness progressing to coma may result. Very high plasma immunoglobulin concentration, which is a common feature of the malignant disorders multiple myeloma and macroglobulinaemia, is a common cause of the hyperviscosity syndrome. Numbers of leukocytes and platelets have little influence on blood flow in health. However, when leukocyte counts exceed 300 × 109/l, usually in leukaemia, flow may be adversely affected, resulting in clinical hyperviscosity.
The hyperviscosity syndrome represents an extreme abnormality of blood flow producing organ dysfunction. However, epidemiological studies suggest that even minor increases in blood viscosity, due to increased haematocrit or fibrinogen concentration, may result in a tendency to vascular occlusion, manifesting as an increased incidence of myocardial infarction and cerebral infarction. The concentration of plasma fibrinogen is a risk factor for atherosclerosis and arterial thrombosis that is at least as potent as the level of serum cholesterol. The interplay between rheological and haemostatic changes in thrombotic disease is not yet fully understood.
Erythrocyte sedimentation rate
The erythrocyte sedimentation rate (ESR) measures the rate at which red cells sediment by gravity in plasma in 1 hour and is a widely used laboratory test. Increased aggregation and sedimentation occur in the presence of high concentrations of immunoglobulin and fibrinogen. As the latter is an acute phase reactant, the ESR is increased in a wide variety of inflammatory and neoplastic conditions. It is an entirely non-specific test and a normal value for ESR can never be used to exclude the presence of significant disease. Direct measurement of plasma viscosity provides equally useful data and has replaced ESR measurement in some diagnostic laboratories.
HAEMOPOIESIS AND BLOOD CELL KINETICS
Haemopoiesis is the formation of blood cells.
Sites of haemopoiesis
In the adult, all blood cells are produced in the red marrow, which is restricted to the bones of the axial skeleton—vertebrae, ribs, sternum, skull, sacrum, pelvis and proximal femora. In these regions the bone marrow is composed of approximately 50% fat, within adipocytes, and 50% blood cells and their precursors (Fig. 23.10). The fatty marrow of other bones is capable of haemopoiesis when requirements for blood cells are increased in some diseases.
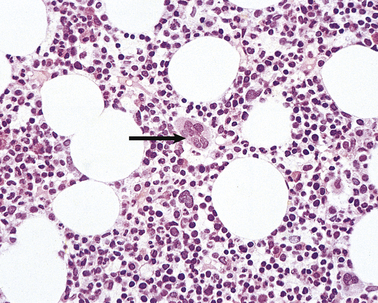
Fig. 23.10 Normal bone marrow. From a section of a bone marrow biopsy from the pelvis. Marrow cells are interspersed between fat spaces. The arrow points to a megakaryocyte.
In the infant and young child, practically all of the bones contain haemopoietically active marrow.
In fetal life, the liver and spleen are the major haemopoietic organs between about 6 weeks and 6–7 months’ gestation; the yolk sac is the main site before 6 weeks. In disease, the liver and spleen can again become haemopoietic organs, even in adult life; this development is referred to as extramedullary haemopoiesis and is particularly associated with the progressive fibrosis of bone marrow seen in the myeloproliferative disorders.
The bone marrow is examined histologically in two ways. Marrow can be aspirated through a needle inserted into a marrow cavity (usually sternum or pelvis), smeared on a slide and stained in a method similar to that for peripheral blood. Further information, particularly on the structure and cellularity of the marrow, can be obtained by preparation of sections of a marrow trephine biopsy: this is a core of tissue obtained using a wide-bore needle (Fig. 23.10).
Additional investigations carried out on bone marrow samples include staining of individual cells with monoclonal antibodies (immunophenotyping and immunohistochemistry) and genetic analysis including karyotyping, fluorescent in situ hybridisation (FISH) and molecular analysis of individual genes.
Haemopoietic stem cells
Studies of bone marrow in culture lead to the conclusion that erythrocytes, leukocytes (including lymphocytes) and platelets are derived from a common, self-replicating precursor cell or ‘pluripotential stem cell’. By a series of cell divisions, cells committed to each line are produced and further divisions result in mature cells—erythrocytes, granular leukocytes, megakaryocytes and T- and B-lymphocytes (Fig. 23.11). The pluripotential stem cells possess the ability to renew in addition to the capacity to differentiate. It is now clear that bone marrow also contains mesenchymal stem cells which can give rise to connective tissues such as fat cells, fibroblasts, bone and cartilage. The development and preferential survival of a malignant clone of haemopoietic cells, derived from mutated bone marrow stem cells, explains the pathological features of the leukaemias and myelodysplastic syndromes.
If human bone marrow is infused intravenously into a subject without functioning marrow, as during bone marrow transplantation treatment, normal blood cell production returns after a period of several weeks. This finding confirms the presence of pluripotential stem cells in bone marrow and also indicates that the microenvironment of the bone marrow is central to normal blood production; stem cells do not tend to thrive in other sites, and blood production resumes only in the marrow cavities after marrow infusion. Stem cells can be made to circulate in the peripheral blood. This is most conveniently achieved by the administration of one of the cytokines (most commonly G-CSF) responsible for stimulation of haemopoiesis—the colony stimulating factors. Using an extracorporeal centrifugation technique these cells can be harvested and used as an alternative to bone marrow cells in transplantation therapy—a ‘peripheral blood stem cell transplant’. Peripheral blood is used as a source of haemopoietic stem cells more commonly now than bone marrow because engraftment is faster and procurement of peripheral blood stem cells does not require the donor to have a general anaesthetic.
There is great interest in the possibility that marrow stem cells may be able to differentiate into diverse tissue types, including neuronal, muscle, liver and vascular cells. This is referred to as transdifferentiation or stem cell plasticity. Although definitive proof of this is still lacking, should it be confirmed it offers exciting new possibilities for treatment of common disorders through transfer of stem cells. Such studies are ongoing and to date show some benefit in treating damaged myocardium and improving blood flow to ischaemic limbs.
Erythropoiesis
The pronormoblast is the earliest red cell precursor that can be identified in the bone marrow. It is a large cell with prominent nucleoli within the nucleus. By a series of four cell divisions a fully haemoglobinised, non-nucleated erythrocyte is produced. During differentiation the nucleus becomes increasingly condensed and the cytoplasm contains increasing amounts of haemoglobin and less RNA; the early, intermediate and late normoblasts can be distinguished morphologically through the increasing haemoglobin content and progressive nuclear condensation (Fig. 23.12). The nucleus is eventually extruded, leaving a ‘polychromatic’ erythrocyte which remains in the marrow for a further 48 hours; it then circulates for approximately 48 hours before maturing in the spleen to an erythrocyte.
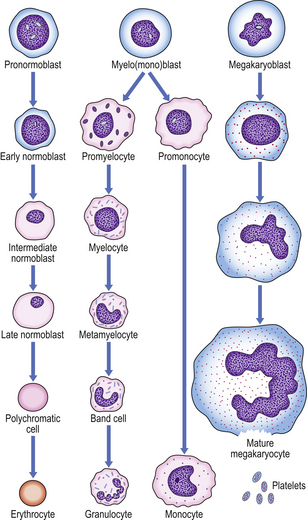
Fig. 23.12 Haemopoiesis. The later morphological stages of erythrocyte, megakaryocyte and myeloid cell development from the committed progenitor cell stage.
Only polychromatic erythrocytes and mature erythrocytes normally circulate. However, nucleated red cell precursors are present in the peripheral blood in some marrow disorders.
Leukopoiesis
The normal bone marrow contains many more myeloid than nucleated erythroid cells (around 5:1). In the granulocyte series these include the myeloblast, promyelocyte and myelocyte, which are capable of cell division, and the metamyelocyte and band cell, which are undergoing maturation without further division (Fig. 23.12). Maturation of granulocyte precursors involves a reduction in cell size, development of cytoplasmic granules, increased condensation of nuclear chromatin, and irregularity of nuclear outline.
Monoblasts cannot easily be distinguished from myeloblasts. They mature in the bone marrow to monocytes via a promonocyte stage. Peripheral blood contains mature granulocytes and monocytes only, in health. However, as previously mentioned, their precursors may enter the circulation in the presence of severe infection or bone marrow disease.
Megakaryocytopoiesis
Megakaryocyte maturation is unique. The earliest identifiable precursor, the megakaryoblast, is a large cell that undergoes nuclear replication without cell division, the cytoplasmic volume increasing as the nuclear material increases, in multiples of 2, up to 32N (Fig. 23.12). Cytoplasmic maturation occurs, often at the 8N stage, and platelets are released. Megakaryocytes are not seen in peripheral blood by routine methods.
Blood cell kinetics
Erythrocytes circulate for an average of 120 days and are then destroyed, predominantly in the bone marrow, but also in liver and spleen. There is no significant storage pool of erythrocytes in humans. In contrast, some 10 times more granulocytes are present in the bone marrow than in the peripheral blood, constituting a storage pool of leukocytes which can be mobilised rapidly in response to some stimuli, such as infection or tissue damage. Granulocytes spend only a few hours in the circulation before they enter the tissues, where they act as phagocytes, surviving for several days under normal circumstances. Monocytes also spend a limited time in the circulation, after which they enter the tissues and become tissue macrophages; they may survive for many months.
Platelets circulate for approximately 10 days. The spleen acts as a reservoir of platelets; some 30% are present in the spleen at any time.
Control of haemopoiesis
Peripheral blood cell counts are normally maintained within close limits. However, the ability of each cell line to respond appropriately to increased requirement is exemplified by the increased red cell production after haemorrhage, the granulocyte leukocytosis in response to sepsis and the enhanced platelet production that results from chronic bleeding.
Erythropoietin is a glycoprotein hormone, produced by peritubular fibroblasts in the kidney, that increases erythropoietic activity. The production of erythropoietin is increased in response to a reduced oxygen tension in the blood reaching the kidney. It results in an increase in the number of cells committed to the erythroid line, reduced maturation time and early release of erythrocytes from the bone marrow. Erythropoietin mediates the physiological response of the bone marrow to anaemia or hypoxia. In pathological states, failure of erythropoietin production is a major contributor to the anaemia of chronic renal failure and this can be corrected by erythropoietin administration; inappropriate erythropoietin production by some renal cysts and tumours results in secondary polycythaemia.
Numerous growth factors have been found to govern production of leukocytes in the bone marrow. They are synthesised mainly by T-lymphocytes, monocytes/macrophages, endothelial cells and fibroblasts of the bone marrow stroma. Examples are interleukins 1, 3 and 6 and the colony stimulating factors. GM-CSF increases stem cell commitment to granulocyte and monocyte production, G-CSF to granulocytes and M-CSF to monocytes. Recombinant forms of some of these cytokines are now in therapeutic use, particularly in cancer chemotherapy, where the duration of drug-induced neutropenia can be limited by cytokine administration.
Thrombopoietin, capable of the stimulation of platelet production, is synthesised principally in the liver. Analogues of thrombopoietin may be of use in stimulating platelet production in bone marrow failure states and as shown recently in immune thrombocytopenic purpura (ITP).
Haemoglobin
Structure, synthesis and metabolism
Some knowledge of haemoglobin structure and metabolism is necessary for an understanding of the pathology of the anaemias. Haemoglobin is the oxygen-carrying pigment. The haem group of haemoglobin is responsible for oxygen carriage and is composed of a protoporphyrin ring structure with an iron atom.
By 1 month of age red cell precursors synthesise predominantly haemoglobin A, composed of four haem groups and four polypeptide (globin) chains (Fig. 23.13), of which two molecular forms are present: alpha and beta chains. Haemoglobin A thus has the structure α2β2. Up to 2.5% of the haemoglobin in adults has delta chains (α2δ2)—haemoglobin A2; and up to 1% of the haemoglobin in adults has gamma chains (α2γ2)—haemoglobin F. Adult blood therefore has predominantly haemoglobin A with some A2 and F (Table 23.3).
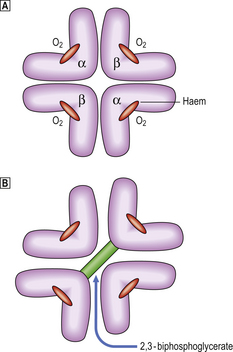
Fig. 23.13 Oxygenated and deoxygenated adult haemoglobin. Uptake and release of oxygen (O2) is associated with movement of the globin chains. On release of O2 the beta chains are moved apart, allowing entry of 2,3-BPG and a reduction in the affinity of the haemoglobin molecule for O2.
In later fetal and early neonatal life haemoglobin F predominates. In early fetal life three other haemoglobins are present: Gower 1, Gower 2 and Portland (Table 23.3).
The whole haemoglobin molecule is thus composed of a tetramer of globin chains, each with a haem group. The complex structure of the molecule is responsible for its oxygen (O2) binding characteristics, the globin chains moving against each other during transfer of O2. The affinity of the haemoglobin molecule for O2 is also controlled by its ability to bind the metabolite 2,3-biphosphoglycerate (2,3-BPG) produced during anaerobic respiration. When 2,3-BPG enters the haemoglobin molecule as the beta chains pull apart during release of O2, the affinity for O2 of the haemoglobin–2,3-BPG complex is reduced, allowing O2 to be given up more readily. Haemoglobin F cannot bind 2,3-BPG and thus has a relatively high O2 affinity, facilitating O2 transfer from maternal blood across the placenta.
At the end of the erythrocyte life-span haemoglobin is metabolised, with conservation of iron and amino acids. Iron is carried by plasma transferrin to the bone marrow and utilised in the synthesis of haem. Globin is degraded to its constituent amino acids, which enter the general pool. Liver, gut and kidneys are all involved in excretion of products of haem breakdown as derivatives of bilirubin.
In the congenital disorders collectively known as haemoglobinopathies, the rate of synthesis of one globin chain type is defective (the thalassaemias) or an abnormal chain is synthesised (the sickle haemoglobinopathies and other haemoglobin variants).
FUNCTIONS OF THE BLOOD
From a consideration of the preceding sections the functions of blood and the major pathological consequences of blood and marrow disease will be apparent.
Oxygen transport is the primary function of the red blood cells. Failure of red cell production, or loss or dysfunction thereof, results in tissue hypoxia affecting the metabolism of all organs.
The cells responsible for host defence against infection are carried, in the blood, from the bone marrow to sites of infection. Infections with bacteria, viruses and fungi are the predictable results of a failure to produce normal leukocytes in adequate numbers.
The primary haemostatic and coagulation mechanisms allow the transport functions of blood to operate without risk of exsanguination from breaches of the vascular compartment. Failure of these leads to spontaneous haemorrhage, whereas a defect in the control mechanisms can result in thrombosis and vascular occlusion.
The diseases that interfere with the function of the blood and their pathological consequences are now described.
ANAEMIAS
Anaemia is present when the haemoglobin level falls below around 130g/l in a male or 115g/l in a female. The different lower limits of normal haemoglobin concentration for neonates, infants and children should be noted (Table 23.1).
The consequences of anaemia are dependent upon the speed of onset. Thus the rapid loss of 10% or more of the circulating blood volume through haemorrhage will result in shock, i.e. the failure of adequate perfusion of all tissues and organs, with consequent hypoxia. In this situation the subject may not initially be anaemic, as both red cells and plasma are lost through haemorrhage. The plasma component is more rapidly replaced, however, and anaemia will be present after several hours have elapsed.
Anaemia that develops more gradually is better tolerated. A haemoglobin concentration as low as 20g/l may be consistent with survival if it develops over a protracted period. The inevitable result of anaemia, however, is a reduction in the oxygen-carrying capacity of the blood and thus chronic tissue hypoxia.
The general consequences of anaemia are due to the tissue hypoxia, which can result in fatty change, especially in the myocardium and liver, and even infarction. Lethargy, increased breathlessness on exertion, and new or worsened ischaemic phenomena are typical clinical features. Breathlessness at rest implies the development of heart failure, a result of severe anaemia. Expansion of the red marrow is present in those anaemias where a marrow response is possible—generally the haemolytic anaemias. Other features are specific to anaemias resulting from a particular mechanism, such as the jaundice of haemolytic anaemias, or are specific to anaemia of a particular type, such as the nail changes of iron deficiency anaemia. Such pathological features are described in the relevant sections.
A low haemoglobin concentration usually reflects a reduction in the body red cell mass. An important exception is pregnancy, when both red cell mass and plasma volume increase, but the latter to a greater degree. This process results in a haemoglobin concentration in blood that is lower than in the non-pregnant state in the presence of a relatively increased red cell mass and overall oxygen-carrying capacity; this condition is often referred to as the physiological ‘anaemia’ of pregnancy. The increased red cell mass during pregnancy is necessary to support the increased metabolic requirement of the mother and fetus. The reason for the expansion of the plasma compartment is obscure, but it may be explained in part by a need for increased skin perfusion for heat loss due to the increased metabolic rate.
Expansion of the plasma volume, resulting in dilutional anaemia, may also occur when the spleen is pathologically enlarged. (The spleen appears to exert a controlling influence on plasma volume.) Other mechanisms also operate in this situation, however, as described under hypersplenism (p. 654).
Classification
Table 23.4 outlines a classification of anaemias. Anaemias are divided into two categories: those where anaemia is due to failure to produce erythrocytes, and those in which erythrocyte loss is increased but production is normal (or usually increased, in response to the anaemia). While useful, this categorisation is an oversimplification, as both mechanisms are present in some anaemias. Thus, in the megaloblastic states, cell production is defective due to lack of vitamin B12 or folic acid for nucleic acid synthesis but, in addition, the erythrocytes that are produced are abnormal and of diminished survival. In thalassaemia, cell production is not optimal due to abnormal haemoglobin synthesis, and there is also increased erythrocyte destruction.
Table 23.4 A classification of anaemias
Type | Cause |
---|---|
Production failure anaemia | |
Haematinic deficiency | Insufficiency of iron, vitamin B12 or folic acid |
Anaemia of chronic disorders | Infection, inflammation and neoplasia |
Dyserythropoiesis |
Hypoplasia Marrow infiltration
Increased red cell loss, lysis or pooling
Haemolysis due toabnormality outsidethe red cell
Hypersplenism
The myeloid and megakaryocytic lines are also involved in some anaemias due to failure of haemopoiesis (megaloblastic anaemia, hypoplastic anaemia) but not in others (iron deficiency anaemia).
Despite these qualifications, the classification described is useful as an aid to determining the cause of the anaemia.
PRODUCTION FAILURE ANAEMIAS
The most commonly encountered anaemias are in the production failure group.
Haematinic deficiency
Haematinics are dietary factors essential for either haemoglobin synthesis or erythrocyte production.
Iron deficiency
Iron deficiency is the commonest cause of anaemia worldwide. It is also the commonest cause of a microcytic hypochromic blood picture, the others being thalassaemias and (rarely) sideroblastic anaemias.
Iron metabolism
Iron is an essential requirement. It is also one of the commonest elements present in the Earth’s crust. Excessive iron deposited in the tissues is, however, toxic, causing damage to the myocardium, pancreas and liver in particular (Ch. 16). As the body has no active method for iron excretion, iron status is controlled largely by its absorption; the capacity to absorb iron is, however, limited and any tendency to increased loss of iron, due to haemorrhage, is highly likely to result in a negative iron balance and iron deficiency. These factors explain the high prevalence of iron deficiency.
Normally, at least 60% of the body iron is in the haemoglobin of erythroid cells. Approximately 30% is stored within the reticulo-endothelial system (also known as the mononuclear phagocyte system), especially in the bone marrow, as ferritin and haemosiderin. A small proportion of total body iron is present in other tissues, especially muscle, and iron-containing enzymes. This tissue iron is relatively conserved during states of iron deficiency. Only a small fraction of the total body iron is in transport, attached to the carrier protein transferrin.
Ferritin is a protein–iron complex. The protein, apoferritin, is a shell made up of 22 subunits. The core is composed of ferric oxyhydride. Haemosiderin consists of partially degraded ferritin aggregates. Ferritin is present in all tissues, but especially in the macrophages of the bone marrow and spleen and in hepatocytes. A small amount is detectable in plasma and, as it is derived from the storage pool of body iron, its concentration is thus an accurate indicator of body iron stores. Low serum ferritin concentration is a useful confirmatory test for iron deficiency. However, because ferritin is an acute phase response protein, the concentration in plasma is not a reliable guide to body iron stores in the presence of infection, inflammation and neoplasia. In those situations serum ferritin may be normal or high despite tissue iron depletion.
Ferritin is water soluble and not visible by light microscopy; haemosiderin is insoluble and forms yellow granules. When exposed to potassium ferrocyanide (Perls’ stain) the granules are blue–black. Examination of aspirated bone marrow stained with Perls’ stain can therefore be used to assess body iron stores reliably. When iron stores are normal, haemosiderin is visible, mainly in the reticulo-endothelial cells of the bone marrow. In iron overload, most of the iron is in the form of haemosiderin and can be easily identified.
Transferrin is an iron-binding beta-globulin responsible for iron transport and delivery to receptors on immature erythroid cells. Each molecule of transferrin can bind two atoms of iron, but normally the transferrin is only one-third saturated (thus the serum iron concentration is normally one-third of the total serum iron-binding capacity). Transferrin is reutilised after delivering its iron. A low transferrin saturation is therefore diagnostic of iron deficiency while high levels are a feature of iron overload with deposition of iron in tissues.
In order to maintain iron balance, sufficient iron must be absorbed to replace that lost from the urinary and gastrointestinal tracts as shed cells and in sweat, together with any extra requirements.
Thus, requirements vary with circumstances, extra iron being required for growth during childhood, for the fetus and placenta and expansion of maternal red cell mass during pregnancy, and to compensate for menstrual loss of women of child-bearing age.
As a Western diet contains only 10–20 mg of iron per day and only a maximum of one-third of this can be absorbed, excess losses of iron of just a few milligrams will inevitably result in negative iron balance and eventual depletion of iron stores. One millilitre of blood contains 0.5 mg iron. Thus, loss of 10 ml of blood daily will inevitably exceed the capacity to absorb sufficient iron, even from a good diet. This explains the finding of some degree of iron depletion in 25% or more of menstruating women.