CHAPTER 96 Anatomy, Histology, Embryology, and Developmental Anomalies of the Small and Large Intestine
ANATOMY
MACROSCOPIC FEATURES
Colon and Rectum
The colon is a tubular structure approximately 30 to 40 cm in length at birth in the full-term infant. In the adult, the colon measures approximately 150 cm, about one quarter of the length of the small intestine. The diameter of the colon is greatest in the cecum (7.5 cm) and narrowest in the sigmoid (2.5 cm). The colon is continuous with the small intestine proximally at the ileocecal valve and ends distally at the anal verge (Fig. 96-1). The external appearance of the colon differs from that of the small intestine because the longitudinal muscle fibers of the colon coalesce into three discrete bands called taeniae, located at 120-degree intervals about the colonic circumference: taenia liberis, taenia omentalis, and taenia mesocolica. The taeniae start at the base of the appendix and extend continuously to the proximal rectum. Outpouchings of the colon, the haustra, are found between the taeniae. Semilunar folds characterize the mucosa between haustra. Small sacs of peritoneum filled with adipose tissue, the appendices epiploicae, are found on the external surface of the colon.
The most proximal portion of the colon, the cecum, lies in the right iliac fossa and projects downward as a blind pouch below the entrance of the ileum. The cecum is a sacculated structure 6 to 8 cm in length and breadth. Because of its large diameter, it is the part of the colon most apt to rupture with distal obstruction, and cecal tumors can grow to be quite large without producing symptoms of obstruction. The mobility of the cecum normally is fixed by a small mesocecum; an anomaly in fixation exists in 10% to 20% of people, especially women, predisposing them to cecal volvulus. The vermiform appendix is a blind outpouching of the ceum that begins inferior to the ileocecal valve. Appendiceal anatomy is discussed further in Chapter 116.
Anal Canal
The anal canal is approximately 5 cm in length in the adult and has discrete upper and lower demarcations. The anorectal ring is located proximally and is composed of the upper portion of the internal sphincter, the longitudinal muscle of the rectum, the deep portion of the external sphincter, and the puborectalis portion of the levator ani muscle; distally, the anal verge represents the transition of anoderm to true skin. The mucosa of the distal 3 cm of the rectum and the anal canal contains 6 to 12 redundant longitudinal folds called the columns of Morgagni, which terminate in the anal papillae. These columns are joined together by mucosal folds called the anal valves, which are situated at the dentate line. The muscularis mucosae disappears in the anorectal canal, and the inner circular coat of muscularis propria thickens to form the internal anal sphincter. The external anal sphincter surrounds the anal canal, and its fibers blend with those of the levator ani muscle to attach posteriorly to the coccyx and anteriorly to the perineal body. The anatomy and function of these muscles are described in more detail in Chapter 125.
Vasculature
The superior mesenteric artery delivers oxygenated blood to the distal duodenum, the jejunum and ileum, the ascending colon, and the proximal two thirds of the transverse colon. The remainder of the colon is supplied by branches of the inferior mesenteric artery. The arterial supply of the anal area is from the superior, middle, and inferior hemorrhoidal arteries, which are branches of the inferior mesenteric, hypogastric, and internal pudendal arteries respectively. Venous drainage of the anus is by both the systemic and portal systems. The internal hemorrhoidal plexus drains into the superior rectal veins and then into the inferior mesenteric vein, which, with the superior mesenteric vein, joins the splenic vein to form the portal vein. The distal anus drains by the external hemorrhoidal plexus through the middle rectal and pudendal veins into the internal iliac vein. (See Chapter 114 for additional discussion of the intestinal blood supply.)
Extrinsic Innervation
The autonomic nervous system—sympathetic, parasympathetic, and enteric—innervates the gastrointestinal tract. The sympathetic and parasympathetic nerves constitute the extrinsic nerve supply and connect with the intrinsic nerve supply, which is composed of ganglion cells and nerve fibers within the intestinal wall. Innervation of the small intestine and colon is discussed in detail in Chapters 97 and 98, respectively.
MICROSCOPIC FEATURES
General Considerations
The small and large intestine share certain histologic characteristics. The wall of the small intestine and colon is composed of four layers: mucosa (or mucous membrane), submucosa, muscularis (or muscularis propria), and adventitia (or serosa) (Fig. 96-2).
Mucosa
The mucosa is the innermost layer formed by glandular epithelium, lamina propria, and muscularis mucosae (Fig. 96-3A and B). The glandular epithelium forms cylindrical structures called crypts. The lamina propria, which supports the epithelium, is a layer of reticular connective tissue with elastin, reticulin, and collagen fibers, lymphocytes, plasma cells, and eosinophilic granulocytes, as well as lymphatics and capillaries. The muscularis mucosae consists of a thin layer of smooth muscle at the boundary of the mucosa and submucosa.
Signaling pathways such as Wnt, bone marrow protein (Bmp), PTEN/PI3K, Notch, hedgehog, platelet-derived growth factor, and SOX9 play important roles in the development of the intestinal epithelium.1–4
Wnt signaling plays a role in promoting cell proliferation; maintains stem cells in an undifferentiated state; defines compartmentalization into Paneth cells, proliferative, and differentiation zones along the crypt-villus axis; and directs early secretory lineage development as well as terminal differentiation of Paneth cells through the transcription factor SOX9.1
Bmps belong to the transforming growth factor-β family. Bmp signaling is important in intestinal development and homeostasis. It antagonizes crypt formation and stem cell self-renewal and has a role in directing maturation of all three secretory cell types (goblet, enteroendocrine, and Paneth). Bmp signaling in the mesenchyme plays a significant role in crypt morphogenesis; loss of Bmp leads to multiplication and elongation of crypts.2
PTEN/PI3K pathway plays a role in cell survival, proliferation, and growth.1
Notch proteins mediate cell fate decisions and pattern by regulating the helix-loop-helix factor that controls terminal differentiation. Notch directs development of absorptive cells and depletion of secretory lineage cells, and increases proliferation.1
The hedgehog (Hh) signaling pathway is important in crypt and villus morphogenesis and maintenance of stem cells.3 Both Sonic (Shh) and Indian (Ihh) play a role. Ihh is critical for the maintenance of intestinal stem cells, whereas Shh inhibits the growth of the villi. The contractile subepithelial pericryptal myofibroblasts represent a major target for Hh signaling. Hh signals sent to the epithelium-associated subepithelial myofibroblasts localize the precrypt structure and maintain the organization of the crypt-villus axis. Hh signaling also inhibits the proliferation or differentiation of smooth muscle and the proliferation compartment of the intestinal epithelium.3
Platelet-derived growth factor A stimulates mesenchymal condensation, proliferation, and evagination of overlying epithelium to form villi.3
Studies in animals also have contributed to the understanding of the molecular mechanism of the different pathways.1,2
Stem cells are pluripotential cells located at the base of the intestinal crypts. Stem cells give rise to all types of mature intestinal epithelial cells and at the same time replenish themselves through self-renewal. Undifferentiated cells have fewer intracellular organelles and microvilli than do absorptive cells. The absorptive cells (see Fig. 96-3A) are high columnar cells with oval basal nuclei, eosinophilic cytoplasm, and a periodic acid–Schiff (PAS)–positive free surface, the brush border (see Fig. 96-3B). On electron microscopic examination, the brush border is seen to be composed of microvilli (see Fig. 96-3C), which are more numerous in the small intestinal than in the colonic epithelium. Small bowel enterocyte microvilli are estimated to increase the luminal surface area of the cell 14- to 40-fold. Goblet cells are oval or round, with flattened basal nuclei (Fig. 96-4A); their cytoplasm is basophilic, metachromatic (see Fig. 96-4B), and PAS positive (see Fig. 96-4C). Paneth cells are flask shaped and have an eosinophilic granular cytoplasm and a broad base positioned against the basement membrane (Fig. 96-5). Paneth cells contain zinc, antimicrobial peptides, and growth factors and secrete lysoenzymes. Enteric antimicrobial peptides produced by Paneth cells protect against intestinal infection and maintain enteric homeostasis.5 A cathelin-related antimicrobial peptide (CRAMP) identified in neonatal epithelium during the first weeks after birth, confers protection from Listeria monocytogenes.5
These neuroendocrine cells historically have been divided into argentaffin cells (granules able to reduce silver nitrate) and argyrophilic cells (granules that reduce silver nitrate only in the presence of a chemical reducer). Argentaffin cells stain positive with bichromate salts and also are called enterochromaffin cells. These cells are oval or triangular (also called “halo cells”) and have a basal position in relation to the remaining epithelial cells (Fig. 96-6A) and a pale cytoplasm filled with dark-stained granules. Variation in shapes and cell types has been detected with immunohistochemical staining. The unifying APUD concept—amine precursor, uptake, and decarboxylation—ascribes common characteristics to these neuroendocrine cells. APUD cells are a group of cells with a common embryonic neural crest origin and with similar cytochemical and electron microscopic features; however, embryologic and morphologic data support an endodermal origin of these cells.
Ultrastructurally, enteroendocrine cells contain membrane-bound granules with variably sized electrodense cores (see Fig. 96-6B), averaging 100 to 250 nm in diameter, and consisting of large dense-core vesicles and smaller, synaptic-type microvesicles. Neurosecretory granules can be demonstrated with the Grimelius stain by light microscopy as dark granules (see Fig. 96-6C), or, more specifically, by immunofluorescence, and with immunohistochemical stains such as neuron-specific enolase, chromogranin, and synaptophysin. Chromogranin enables visualization of the large dense-core vesicles, and synaptophysin targets the small synaptic-like microvesicles (see Fig. 96-6D).6 Vesicular monoamine transporter 1 (VMAT1) and 2 (VMAT2) are two isoforms of the adenosine triphosphate (ATP)–dependent vesicular monoamine transporters. These antigens, derived from both the large and small dense-core vesicles, are expressed differentially in small dense-core vesicles. Both are expressed in neuroendocrine cells, but VMAT1 is restricted to serotonin-producing enterochromaffin cells, and VMAT2 is expressed in histamine-producing cells, enterochromaffin-like cells, and pancreatic islet cells.7 Specific immunohistochemical stains allow for identification of individual protein products of the neuroendocrine cells.
Besides releasing hormones in the blood, neuroendocrine cells also regulate secretion, absorption, motility, mucosal cell proliferation, and possibly immunobarrier control.6 Electron microscopy and immunohistochemistry have led to the identification of a variety of cell types (Table 96-1). Designation according to the nature of the stored peptide is preferable to characterization of neuroendocrine cells by letters. Serotonin-producing enterochromaffin cells, vasoactive intestinal polypeptide (VIP), and somatostatin D cells are distributed throughout the small and large intestine. Gastrin-, ghrelin-, gastric inhibitory peptide (GIP)-, secretin-, and cholecystokinin-producing cells are found predominantly in the stomach and proximal small intestine; peptide YY-, glucagon-like peptide (GLP)-1-, GLP-2-, and neurotensin-secreting cells are found in the ileum.8
Table 96-1 Enteroendocrine Cells of the Intestinal Tract: Cell Types and Products, Vesicle Markers, and Distribution
Neuroendocrine cells originate from a common precursor cell in the intestinal crypt. The earliest cell fate is regulated by the Notch signaling pathway (see earlier). Math1 is the first factor involved in endocrine specification, followed by neurogenin3.8 Pax4 and Pax6, paired ox homeodomain transcription factors, and Nkx2.2 also are required for neuroendocrine differentiation.8,9 As mature neuroendocrine cells migrate to the tip of the villi, they undergo apoptosis and are extruded into the lumen.
Interstitial cells of Cajal (ICC) are present in both the small intestine and the colon and are mesenchymal cells, located in the myenteric plexus, the muscularis propria, and the submucosa (Fig. 96-7). The distribution of the ICC is similar in children and in adults although a difference in their distribution is seen in fetuses of different gestational ages.10 Recognized as the pacemaker cells of the intestine, the ICC regulate intestinal motility by generating slow waves and determining frequency of smooth muscle contraction; they also amplify the neuronal signals, mediate neurotransmission from enteric motor neurons to smooth muscle cells, and set the smooth muscle membrane potential gradient. The ICC are spindle shaped or stellate, with long ramified processes, and have large, oval light-staining nuclei with sparse perinuclear cytoplasm. The ICC express the receptor for tyrosine kinase (c-Kit) or CD117 which is necessary for their maintenance. Serotonin regulates the number of the ICC by increasing their proliferation.11 Immunohistochemical stains that use antibodies against c-Kit allow the ICC to be labeled. The distribution and onset of appearance of these cells in the gastrointestinal tract have been described.10
Muscularis or Muscularis Propria
The muscularis propria, mainly responsible for contractility, consists of two layers of smooth muscle: an inner circular coat and an outer longitudinal coat arranged in a helicoidal pattern. A prominent nerve fiber plexus called the myenteric plexus, or Auerbach’s plexus, is found between these two muscle layers (Fig. 96-8). Parasympathetic and postganglionic sympathetic fibers terminate in parasympathetic ganglion cells, and postganglionic parasympathetic fibers terminate in smooth muscle.
Small Intestine
The mucosa of the small intestine is characterized by mucosal folds (plicae circulares, or valves of Kerckring) and villi. The mucosal folds are composed of mucosa and submucosa. Villi are mucosal folds that decrease in size from the proximal to distal small intestine and are of different shapes in the various segments of the small intestine: they may be broad, short, or leaf-like in the duodenum; tongue-like in the jejunum; and finger-like more distally (Fig. 96-9A). The villous pattern also may vary in different ethnic groups. Thus, for example, biopsy specimens from Africans, Indians, South Vietnamese, and Haitians have shorter and thicker villi, an increased number of leaf-shaped villi, and more mononuclear cells in comparison with specimens from North Americans.
Intestinal villus morphogenesis begins when mesenchymal aggregates impinge on the basal aspect of the epithelium to produce primitive folds. By nine to 10 weeks of gestation, the pseudostratified squamous epithelium converts to a single layer of columnar cells that lines mesenchymal stalks or the lamina propria.12 During mid- to late gestation, the basic tissue architecture of the intestine is established through epithelial-mesenchymal interaction. Induced by signals from mesoderm-derived mesenchyme, the endoderm-derived epithelium evaginates to form villi and intervillus regions. The intervillus region eventually invaginates into the mucosa to form crypts.1 Contractile subepithelial pericryptal myofibroblasts contribute mechanically to crypt formation and are the major source of instructive signals to the epithelium.3
Two types of glands are present in the small intestine: Brunner’s glands and crypts of Lieberkühn (intestinal crypts). Brunner’s glands are submucosal glands (see Fig. 96-9B) found primarily in the first portion of the duodenum and in decreased numbers in the distal duodenum; their function is to secrete a bicarbonate-rich alkaline secretion that helps neutralize gastric chyme. In children these glands also may be present in the proximal jejunum. Brunner’s glands open into the intestinal crypts and morphologically resemble pyloric glands.
Crypts of Lieberkühn are tubular glands that extend to the muscularis mucosae (see Fig. 96-5). The crypts are occupied mainly by undifferentiated cells and Paneth cells. Cells are generated at the crypt base and proceed to migrate toward the villus. During this migration, these cells mature and differentiate into a secretory lineage (goblet cells, enteroendocrine cells, Paneth cells) and enterocytes. The commitment of the stem cells to differentiate is acquired in the upper third of the crypt, where cells lose their ability to divide. The constant renewal of enterocytes is regulated by human acyl-coenzyme A synthetase.13
Most types of enteroendocrine cells are present in the duodenum. Cells that produce ghrelin, gastrin, cholecystokinin, motilin, neurotensin, GIP, and secretin are restricted to the small intestine.6
The proportions of these cells differ in the villi and crypts, as well as in different segments of the intestine. Ninety percent of the villus epithelial cells are absorptive cells intermingled with goblet and enteroendocrine cells. The proportion of goblet to absorptive cells is increased in the ileum. The ICC are more abundant in the myenteric plexus of the small bowel than in the colon.10
Colon
Colonic epithelial cells are generated from stem cells at the base of the crypts and migrate toward the intestinal lumen after three to five days, on initiation of apoptosis. Most epithelial cells undergo apoptosis when they lose contact with the extracellular matrix and are shed into the lumen through caspase activation. Caspase activation is responsible for the cleavage of essential intracellular proteins leading to apoptosis and therefore loss of anchorage.14
Anal Canal
Microscopically the anal canal is divided into three zones: proximal, intermediate or pectinate, and distal or anal skin. The proximal zone is lined by stratified cuboidal epithelium, and the transition with the rectal mucosa, which is lined by high columnar mucus-producing cells, is called the anorectal histologic junction (Fig. 96-10A). The intermediate or pectinate zone is lined by stratified squamous epithelium but without adnexae (e.g., hair, sebaceous glands) and also is referred to as anoderm. Its proximal margin, in contact with the proximal zone, is called the dentate line; its distal margin, in contact with the anal skin, constitutes the pectinate line, also referred to as the mucocutaneous junction (see Fig. 96-10B). The anal skin is lined by squamous stratified epithelium and contains hair and sebaceous glands.
Nerves
The intrinsic nervous system (enteric nervous system) consists of subserosal, muscular, and submucosal plexuses. The subserosal plexus contains a network of thin nerve fibers, without ganglia, that connects the extrinsic nerves with the intrinsic plexus. The myenteric plexus, or Auerbach’s plexus, is situated between the outer and inner layers of the muscularis propria (see Fig. 96-8); it consists of ganglia and bundles of unmyelinated axons that connect with the ganglia forming a meshwork. These axons originate from processes of the ganglion cells and extrinsic vagus and sympathetic ganglia. The deep muscular plexus is situated on the mucosal aspect of the circular muscular layer of the muscularis propria. It does not contain ganglia; it innervates the muscularis propria and connects with the myenteric plexus. The submucosal plexus, or Meissner’s plexus, consists of ganglia and nerve bundles. The nerve fibers of this plexus innervate the muscularis mucosae and smooth muscle in the core of the villi. Fibers from this plexus also form a mucosal plexus that is situated in the lamina propria and provides branches to the intestinal crypts and villi. The ganglion cells of the submucosal plexus are distributed in two layers: one is adjacent to the circular muscular layer of the muscularis propria; the other is contiguous to the muscularis mucosae. Ganglion cells are large cells, isolated or grouped in small clusters called ganglia (Fig. 96-11). Ganglion cells have an abundant basophilic cytoplasm, a large vesicular round nucleus, and a prominent nucleolus. Ganglion cells are scarce in the physiologically hypoganglionic segment 1 cm above the anal verge.
EMBRYOLOGY
MOLECULAR REGULATION OF INTESTINAL MORPHOGENESIS
The induction of endoderm appears to be governed by nodal or transforming growth factor-β signaling.15 Specification is initiated by transcription factors expressed in the different regions of the intestinal tube. Thus, PDX1 specifies the duodenum, CDXC the small intestine, and CDXA the large intestine and rectum.16 Differentiation of the gastrointestinal tract depends on the interaction between the endoderm and mesoderm through the Hox code. Signaling from the mesoderm to endoderm is regulated by the Hox genes that encode homeodomain-containing transcription factors. Induction of the Hox code in the mesoderm results from expression of Shh through the endoderm of the midgut and hindgut. Shh is a signaling molecule that acts as a morphogen or form-producing substance in a variety of organ systems. When prompted by this code, the mesoderm instructs the endoderm to form the various components of the midgut and hindgut regions, for example, the small bowel, cecum, colon, and cloaca.16 As indicated by animal studies, Hox genes contribute to the subdivision of the intestine, and formation of the ileocecal valve that separates the small and the large intestine. Shh also plays a crucial role in the development of the hindgut.17
The primitive gut results from incorporation of the endoderm-lined yolk sac cavity into the embryo, following embryonal cephalocaudal and lateral folding. The primitive gut is composed of a blind-ended tube in the cephalic and caudal portions of the embryo, which is the progenitor of the foregut and hindgut; the midgut (Fig. 96-12) is connected to the yolk sac by the vitelline duct. The endoderm gives rise to the epithelial lining of the gastrointestinal tract; muscle, connective tissue, and peritoneum originate from the splanchnic mesoderm. During the ninth week of development, the epithelium begins to differentiate from the endoderm with villus formation and differentiation of epithelial cell types. Organogenesis is complete by 12 weeks of gestation.
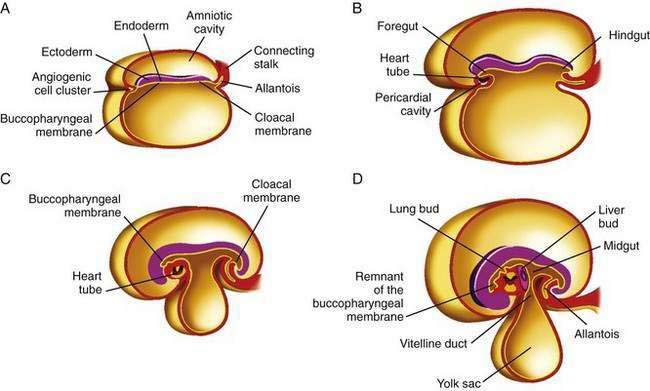
Figure 96-12. Formation of the foregut, midgut, and hindgut (see text for details).
(From Sun B, editor. Langman’s Medical Embryology. 9th ed. Philadelphia: Lippincott Williams & Wilkins; 2004.)
Initially the foregut, midgut, and hindgut are in broad contact with the mesenchyma of the posterior abdominal wall. The intraembryonic cavity is in open communication with the extraembryonic cavity. Subsequently the intraembryonic cavity loses its wide connection with the extraembryonic cavity. By week five of embryonic development, splanchnic mesoderm layers are fused in the midline and form a double-layered membrane, the dorsal mesentery, between the right and left halves of the body cavity. The mesoderm surrounds the intestinal tube and suspends it from the posterior body wall, allowing it to hang into the body cavity. The caudal portions of the foregut, the midgut, and most of the hindgut thus are suspended from the abdominal wall by the dorsal mesentery extending from the duodenum to the cloaca. The dorsal mesentery forms the mesoduodenum in the duodenum, the dorsal mesocolon in the region of the colon, and the mesentery proper in the region of the jejunum and ileum.16
SPECIFIC STRUCTURES AND SYSTEMS
Duodenum
The duodenum originates from the terminal portion of the foregut and cephalic part of the midgut. With rotation of the stomach, the duodenum becomes C-shaped and rotates to the right; the fourth portion becomes fixed in the left upper abdominal cavity. The mesoduodenum fuses with the adjacent peritoneum; both layers disappear, and the duodenum becomes fixed in its retroperitoneal location. The lumen of the duodenum is obliterated during the second month of development by proliferation of its cells; this phenomenon is shortly followed by recanalization. Because the foregut is supplied by the celiac artery and the midgut by the superior mesenteric artery, the duodenum is supplied by both arteries and therefore is relatively protected from ischemic injury.16
Midgut
In a 5-week embryo, the midgut is suspended from the dorsal abdominal wall by a short mesentery and communicates with the yolk sac by way of the vitelline duct. The midgut gives rise to the duodenum distal to the ampulla, to the entire small bowel, and to the cecum, appendix, ascending colon, and the proximal two thirds of the transverse colon. The midgut rapidly elongates with formation of the primary intestinal loop. The cephalic portion of this loop, which communicates with the yolk sac by the narrow vitelline duct, gives rise to the distal portion of the duodenum, the jejunum, and a portion of the ileum; the distal ileum, cecum, appendix, ascending colon, and proximal two thirds of the transverse colon originate from the caudal limb. During week 6 of embryonic development, the primary intestinal loop enters the umbilical cord (physiologic umbilical herniation) (Fig. 96-13), and by week 10 it re-enters the abdominal cavity. The proximal portion of the jejunum is the first portion of the intestine to re-enter the abdominal cavity and becomes located on the left side; the subsequent loop that re-enters the abdominal cavity locates to the right. The cecal bud is the last segment to re-enter the abdominal cavity. The cecum originates as a small dilatation of the caudal limb of the primary intestinal loop by approximately 6 weeks of development. Initially it lies in the right upper quadrant; then it descends to the right iliac fossa, placing the ascending colon and hepatic flexure in the right side of the abdominal cavity. The appendix originates from the distal end of the cecal bud. Because the appendix develops during descent of the colon, its final position frequently is retrocecal or retrocolonic.
The primary intestinal loop rotates counterclockwise for approximately 270 degrees around an axis formed by the superior mesenteric artery. This rotation occurs in three stages (Fig. 96-14): the first stage occurs between six and eight weeks (90 degrees), the second stage is at nine weeks (180 degrees), and the third stage is at 12 weeks of gestation (270 degrees). Elongation of the bowel continues, and the jejunum and ileum form a number of coiled loops within the peritoneal cavity.16
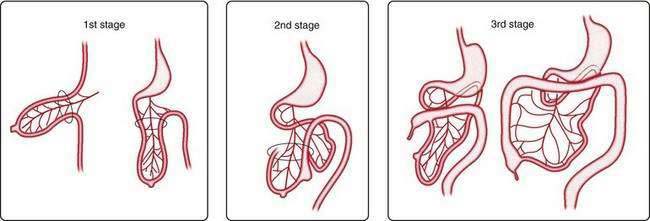
Figure 96-14. The three stages of normal intestinal rotation (see text for details).
(From Gosche JR, Touloukian RJ. Congenital anomalies of the midgut. In: Wyllie R, Hyams JS, editors. Pediatric Gastrointestinal Disease. Pathophysiology, Diagnosis, Management. 2nd ed. Philadelphia: WB Saunders; 1999.)
Mesentery
When the caudal limb of the primitive intestine moves to the right side of the abdominal cavity, the dorsal mesentery twists around the origin of the superior mesenteric artery. After the ascending and the descending portions of the colon reach their final destinations, their mesenteries fuse with the peritoneum of the posterior abdominal wall, and they become retroperitoneal organs. The appendix, cecum, and descending colon retain their free mesentery. The transverse mesocolon fuses with the posterior wall of the greater omentum. The mesentery of the jejunum and ileum at first is in continuity with the ascending mesocolon; after the ascending colon becomes retroperitoneal, the mesentery only extends from the duodenum to the ileocecal junction.16
Arterial System
Vascular endothelial growth factor (VEGF)-A and its receptors, VEGFR-1 and VEGFR-2, are important for endothelial cell proliferation, migration, and sprouting. Angiopoietins and their receptors, Tie1 and Tie2, play a role in remodeling and maturation of the developing vasculature. Mutation in Tie2 has been reported in vascular dysmorphogenesis. Vascular malformation is briefly discussed in Chapter 36.