CHAPTER 55 Anatomy, Histology, Embryology, and Developmental Anomalies of the Pancreas
The pancreas was one of the last organs in the abdomen to receive the critical attention of anatomists, physiologists, physicians, and surgeons.1,2 It was first referred to as the “finger of the liver” in the Talmud, written between 200 bc and ad 200. Galen named it (though Ruphos, circa ad 100, should probably be credited2), and thought the pancreas served to support and protect blood vessels. Vesalius considered the organ a cushion for the stomach. Little further information was available until Wirsung demonstrated the pancreatic ducts of humans in 1642 and de Graaf discovered pancreatic secretion from the pancreatic fistula of dogs in 1664.
The histologic structure of the pancreas was first described in 1869 by Langerhans. Shortly thereafter, Heidenhain3 characterized the periodic postprandial changes that occurred in the histology of the canine pancreas. He found that as the granular regions of cells disappeared after feeding, the enzyme activity in pancreatic juice increased; he concluded that the granules contained the precursors of the digestive enzymes.
ANATOMY
The pancreas is a soft, elongated, flattened gland 12 to 20 cm in length.4–6 The adult gland weighs between 70 and 110 g. The head lies behind the peritoneum of the posterior abdominal wall and has a lobular structure. The pancreas is covered with a fine connective tissue but does not have a true capsule. The head of the pancreas is on the right side and lies within the curvature of the duodenum. The neck, body, and tail of the pancreas lie obliquely in the posterior abdomen, with the tail extending as far as the gastric surface of the spleen (Fig. 55-1).
The body passes laterally and merges with the tail of the pancreas without a discernible junction point. The tail is relatively mobile, its tip usually reaching the hilus of the spleen. With the splenic artery and vein, the tail is contained between the two layers of the splenorenal ligament. The splenocolic ligament attaches the splenic flexure of the colon to the spleen and brings it near the tail of the pancreas. The relationship of the pancreas to important structures in the posterior abdomen is seen in Figure 55-2.
The distal end of the common bile duct, the duodenum, and the head of the pancreas form a unit. The common bile duct is located to the right of the gastroduodenal artery in the posterior wall of the duodenum. The bile duct passes through the substance of the pancreatic head, usually to join with the main pancreatic duct for some distance to reach the duodenal papilla (Fig. 55-3A).
DUCTAL STRUCTURES
The main pancreatic duct (of Wirsung) begins near the tail of the pancreas. It is formed from anastomosing ductules draining the lobules of the gland. It courses left to right and is enlarged by additional ducts. Through the tail and body, the duct lies midway between the superior and inferior margins and slightly posterior. The main duct turns caudal and posterior on reaching the head of the pancreas. At the level of the major papilla, the duct turns horizontally to join usually with the common bile duct (see Fig. 55-3A). This short common segment is the ampulla of the bile duct, which terminates in the duodenal papilla.
The relationship of the common bile duct and the duct of Wirsung at the papilla is complex. The ducts may open separately at the ampulla and have an interposed septum or may have a common channel. A common channel for bile and pancreatic secretion is ordinarily formed by the absence of a septum between the biliary and pancreatic ducts as they approach the ampulla of Vater. In adults studied by endoscopic retrograde cholangiopancreatography (ERCP), the length of the common channel averages 4.5 mm, with a range of 1 to 12 mm.7,8 In various series, more than two thirds of patients had some degree of a common channel.9–13 In a large autopsy series, 74% of patients had a common channel, 19% had separate openings, and 7% had an interposed septum.9
The accessory pancreatic duct of Santorini is frequently present and usually communicates with the main duct (see Fig. 55-3A). The accessory duct lies anterior to the bile duct and usually drains into the minor papilla, which lies proximal to the ampulla of Vater in the second duodenum. The accessory duct is patent in 70% of autopsy specimens. In about 10% of individuals there is no connection between the accessory duct and the main duct.14 A number of variations in the two pancreatic ducts may be encountered.
The greatest diameter of the main pancreatic duct is in the head of the pancreas, and the duct gradually tapers, progressing to the tail of the pancreas. The main duct ranges from 3.1 to 4.8 mm in the head of the pancreas and tapers to 0.9 to 2.4 mm in the tail.15 Specific normal limits of pancreatic duct diameter in the head (4 to 5 mm), body (3 to 4 mm), and tail (2 to 3 mm) are generally accepted. However, studies have shown an increase in pancreatic duct size with age and pancreatic disease.16–18
CIRCULATION
The pancreas has a rich circulation derived from branches of the celiac and superior mesenteric arteries.18,19 The head of the pancreas and surrounding duodenum are supplied by two pancreaticoduodenal arterial arcades. They are formed by the anterior and posterior superior pancreaticoduodenal arteries from the hepatic branch of the celiac artery that join a second pair of anterior and posterior inferior pancreaticoduodenal arteries.
LYMPHATIC DRAINAGE
The lymphatics, in general, drain the surface network of lymph toward regional nodes and are formed near the larger blood vessels.20,21 The superior lymphatic vessels run along the upper border of the pancreas closely with the splenic blood vessels. Those on the left side of the body and tail empty into nodes in the splenic hilum. Those on the right side of the body and the pancreatic neck empty into nodes near the upper border of the head. They also receive tributaries from the anterior and posterior pancreatic surfaces. The inferior lymphatic vessels run with the inferior pancreatic artery. Those that drain the lower left side of the body and tail drain toward nodes in the splenic hilum. The remaining regions of the neck and body drain toward the right.
HISTOLOGY AND ULTRASTRUCTURE
The pancreas is a compound, finely nodular gland that is grossly similar to but less compact than the salivary glands. It is surrounded by fine connective tissue but does not have a fibrous tissue capsule. The lobules are visible on gross examination and are connected by connective tissue septa that contain the blood vessels, nerves, lymphatics, and excretory ducts (constituting about 18% of this organ). The gland is a mixed exocrine (about 80%) and endocrine (about 2%) organ (Fig. 55-4). The endocrine portion consists of the islets of Langerhans, which are spherical clusters of light-staining cells scattered throughout the pancreas (see Chapter 1). The exocrine portion consists of numerous dark-staining acini composed of tubular and spherical masses of cells, which are the subunits of the lobule.22,23 Silicone casts of the duct lumen formed by retrograde injection indicate that the tubular portions of the acini are extensive and that the exocrine cells are arranged primarily as curved, branching tubules that anastomose and end blindly (Fig. 55-5).24
The lumen of the acinus is the origin of the secretory duct and contains centroacinar cells, which are unique to the pancreas. These cells are pale staining in histologic sections and smaller than the acinar cells. The lumen of the acinus leads into the intralobular ducts, which are covered by low columnar epithelial cells similar in appearance to the centroacinar cells. These ducts are nonstriated and anastomose to form the interlobular ducts, which are lined by a columnar epithelium (see Fig. 55-4). Goblet cells and occasional argentaffin cells also are present. The interlobular ducts anastomose to become the main pancreatic duct. The larger ducts have a somewhat thick wall consisting of connective tissue and elastic fibers. Acinar, ductal, and islet cells can be distinguished by monoclonal antibodies specifically reactive with these cell types.25–27
Acinar cells are tall, pyramidal, or columnar epithelial cells, with their broad bases on a basal lamina and their apices converging on a central lumen (Fig. 55-6). In the resting state, numerous eosinophilic zymogen granules fill the apical portion of the cell. The basal portion of the cells contains one or two centrally located, spherical nuclei and extremely basophilic cytoplasm. The Golgi complex lies between the nucleus and zymogen granules and can be seen as a clear, nonstaining region (see Fig. 55-6).
The acinar cells undergo cyclic changes in morphology in response to feeding and digestion.28,29 After a large meal, the zymogen granule content of the cells is depleted. This apparently occurs by a decrease in the size and number of granules.28 After depletion of the granules, the Golgi apparatus may be observed at the apex of the cell and appears more extensive than in the resting state. The reductions in size and number of granules occur with a substantial increase in pancreatic enzyme secretion (see Chapter 56).
The subcellular structure of the acinar cells can be visualized at the electron-microscopic level (Fig. 55-7). The acinar cell has several short, slender microvilli about 0.2 µm in length that extend into the lumen of the acinus. The lumen typically contains flocculent electron-dense material, which presumably is the secreted digestive enzymes. Thin filaments form the axis of the microvilli as well as a network beneath the apical plasmalemma. These microfilaments apparently play a structural role because their disruption causes expansion of the acinar lumen and loss of microvilli.30 Adjacent cells are joined at the apical surface by electron-dense intercellular junctions. Tight junctions form a belt-like band around the apical end of the cell and are produced by the apposition of the external membrane leaflets of neighboring cells.31 These junctions prevent the reflux of secreted substances from the duct into the intercellular space. Gap junctions are distributed on the lateral cellular membranes and are formed by the apposition of larger, disk-shaped membrane plaques. They allow communication between cells. Below the junctions, the lateral cell borders are relatively straight and have a few, small interdigitations. Pancreatic lateral cell membranes display unique antigenic determinants that are not found on apical cell membranes.32
The nucleus usually is spherical, about 6 µm in diameter,33 with one or more nucleoli in the interior and patches of dense heterochromatin along the inner nuclear membrane. Numerous conspicuous nuclear pores are located at regions where the lightly stained euchromatin makes contact with the nuclear membrane. These pores presumably are the sites where messenger and transfer ribonucleic acids (RNAs) are transported out of the nucleus into the cytoplasm. Binucleate cells also are seen occasionally.
Mitochondria are elongate cylindrical structures that may appear oval in cross-section and may contain well-developed cristae and many matrix granules. They occur throughout the cytoplasm, among the granular endoplasmic reticulum or zymogen granules, and adjacent to the basolateral cell border. The cytoplasmic matrix occupies about 45% of the cell volume.34
Granular endoplasmic reticulum (see Fig. 55-7) occupies about 20% of the cell volume34,35 and fills most of the basal region of the acinar cells, although small amounts also occur in the apical region adjacent to and among the zymogen granules. This reticulum is composed of numerous parallel cisternal membranes covered with closely spaced attached ribosomes, giving the structures a granular appearance. On the basis of studies with laboratory animals, the ribosomes of the granular endoplasmic reticulum have been found to be the site of protein synthesis.36,37
The Golgi complex is located between the nucleus and the mass of zymogen granules present in the resting gland (see Fig. 55-7). It consists of flattened, membranous saccules as well as small vesicles or vacuoles that contain flocculent electron-dense material. The Golgi saccules have been distinguished from other intracellular vesicles by enzyme cytochemistry and by immunohistochemistry using antienzyme and antireceptor antibodies.38 The Golgi complex is believed to play an important role in the transport of secretory proteins and the formation of zymogen granules. The mechanisms by which these processes occur are still unresolved.
The secretory granules of the pancreas usually are divided into two types, electron-lucent condensing vacuoles and electron-dense zymogen granules. The condensing vacuoles are typically seen in the vicinity of the Golgi complex and, on the basis of autoradiographic data,36,37 are believed to be precursors of the zymogen granules. They are membrane-bound vesicles slightly larger than zymogen granules and much less numerous, occupying only about 2% of the cytoplasm.35 Zymogen granules also are spherical, membrane-bound vesicles, slightly less than 1 mm in diameter,28,39,40 filled with electron-dense material that apparently represents the digestive enzymes (see Fig. 55-7).
Studies of the chemical composition of the zymogen granules have shown that they contain about 12 to 15 different digestive enzymes, which make up about 90% of the granule protein.41–44 Each granule apparently contains the entire complement of secreted digestive enzymes, because labeled antibodies to several different enzymes have been located over single zymogen granules from different cells.45,46 Individual zymogen granules can differ markedly in the concentration of specific digestive enzymes contained within the granules.47 The digestive enzymes within the granules are not in solution or suspension but in a solid-state array, which exhibits specific binding between the enzymes themselves and between the enzymes and the granule membrane.48–51 Isolated zymogen granules are stable at slightly acid pH; at alkaline pH, they release their enzymes into solution.49,51,52 This behavior may account for the solubilization of digestive enzymes within the alkaline duct lumen.
The centroacinar cells (Fig. 55-8) and duct cells have electron-lucent cytoplasm containing few cytoplasmic organelles or specializations. They typically contain free ribosomes and small, round mitochondria. They contain virtually no granular endoplasmic reticulum and therefore are not active in protein synthesis for secretion. Farther down the ducts, the cells contain more mitochondria, but they are never associated with invaginations of the basolateral surface as occur in the transporting ductal epithelium of the salivary glands. Both centroacinar and duct cells secrete bicarbonate and water. Carbonic anhydrase, the enzyme responsible for formation of bicarbonate, has been demonstrated in the epithelium.23
The islets of Langerhans number about 1 million in the human pancreas and consist of anastomosing cords of polygonal endocrine cells (see Fig. 55-4). Each islet is about 0.2 mm in diameter, much larger than an acinus, and separated from the surrounding exocrine tissue by fine connective tissue fibers, which are continuous with those of the exocrine gland.
Each islet is surrounded and penetrated by a rich network of capillaries lined by a fenestrated endothelium. The capillaries are arranged in a portal system that conveys blood from the islets to acinar cells (Fig. 55-9).53–57 This insula-acinar portal system consists of afferent arterioles that enter the islet, form a capillary glomerulus, and leave the islet as efferent capillaries passing into the exocrine tissue. A parallel arterial system supplies blood directly to the exocrine pancreas (see Fig. 55-9), and yet this portal system permits the local action of islet hormones, especially insulin, on the exocrine pancreas.55–57 Acinar cells surrounding islets of Langerhans, termed peri-insular acini, are morphologically and biochemically different from acini situated farther away (tele-insular acini).58,59 Peri-insular acini have larger cells, nuclei, and zymogen granule regions58 and different ratios of specific digestive enzymes.59
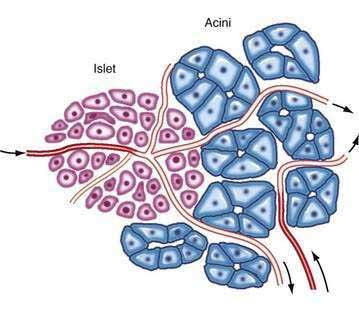
Figure 55-9. Schematic diagram of the insuloacinar portal system, illustrating the dual blood supply to the exocrine pancreas.
(From Goldfine ID, Williams JA. Receptors for insulin and CCK in the acinar pancreas: Relationship to hormone action. Int Rev Cytol 1983; 85:1.)
Although the acinar cell secretes several different digestive enzymes in the exocrine pancreas, each cell type in the endocrine pancreas appears to secrete a single hormone. The four major types of cells found are B cells, A cells, D cells, and pancreatic polypeptide (PP) cells.60,61 B cells (beta cells), the most numerous (50% to 80%), secrete insulin.60 A cells or alpha cells (5% to 20%) secrete glucagon. PP cells (10% to 35%) secrete pancreatic polypeptide. D cells (5%) secrete somatostatin. Other rare cell types occur in the islet.62 In humans the islets are subdivided into units, each of which exhibits a central aggregation of B cells surrounded by varying numbers of peripherally located cells that secrete the other hormones.
EMBRYOLOGY
The pancreas first appears in embryos of about 4 mm in the fourth week of gestation.63,64 The outpouchings from the endodermal lining of the duodenum develop at this time: the ventral pancreas and the dorsal pancreas (Fig. 55-10A). The dorsal anlage grows more rapidly, and by the sixth week, it is an elongated nodular structure extending into the dorsal mesentery, within which its growth continues (see Fig. 55-10B). The ventral pancreas remains smaller and is carried away from the duodenum by its connection with the common bile duct. The two primordia are brought into apposition by uneven growth of the duodenum, and they fuse by the seventh week (see Fig. 55-10C). The tail, body, and part of the head of the pancreas are formed by the dorsal component; the remainder of the head and the uncinate process derive from the ventral pancreas. These primitive relations are still distinguishable in the adult pancreas.64
Both of the primitive pancreata contain an axial duct. The dorsal duct arises directly from the duodenal wall, and the ventral duct arises from the common bile duct. On fusion of the ventral and dorsal components, the ventral duct anastomoses with the dorsal one, forming the main pancreatic duct (see Fig. 55-10D). The proximal end of the dorsal duct becomes the accessory duct of Santorini in the adult and is patent in 70% of specimens.65 The common outlet of the bile duct and pancreatic duct observed in most adults is the result of the common origin of the bile duct and the ventral pancreas.
Distinct differences in morphology, enzyme content, and secretory capacity exist between the embryonic pancreas and the adult pancreas.66–72 Early in development, the pancreas has no zymogen granules and little granular endoplasmic reticulum.66–70 In humans, the pancreas is composed of undifferentiated epithelial cells at 9 weeks of gestation.66 During subsequent cell differentiation, the specific activity of the digestive enzymes increases some thousand-fold,71,72 and the granules increase in size and come to occupy most of the cytoplasm of the cells, including the basolateral regions.67–70 At 12 weeks of gestation in humans, zymogen granules are first seen on electron microscopy. The cells also contain a Golgi complex and granular endoplasmic reticulum in relatively small amounts. By 20 weeks of gestation, larger zymogen granules typical of the adult are seen.66 Each digestive enzyme has a characteristic rate of accumulation and increases in concentration at different times.71,72 At birth, the granules in laboratory animals are the largest normally found in the pancreas, being about six times the volume of the granules in adults.73,74 At about this time, the capacity for stimulated secretion is attained. Differentiation of the pancreas continues beyond birth with regard to the size of the zymogen granules as well as the enzyme content of the tissue.75,76
The endocrine pancreas differentiates on roughly the same time course as the exocrine portion,77,78 and the appearance of islet hormones also precedes the appearance of secretion granules in the cells. In humans, endocrine cells are first observed singly or in small clusters along the basolateral portion of undifferentiated acinar cells (9 to 10 weeks), but by 12 to 16 weeks, distinct islets in various stages of complexity can be observed. The development of islets in the PP-rich regions lags slightly behind that of the glucagon-rich regions.79 Insulin cell numbers increase continuously with age, whereas glucagon cell numbers increase during fetal life and then decrease in infants and adults. The number of somatostatin cells is elevated in fetal and infant stages, whereas PP cells are the least abundant cells during these stages.79
SIGNALING AND GROWTH FACTORS
Hedgehog (Hh) proteins are signaling molecules that regulate various aspects of morphogenesis, including cellular proliferation and differentiation (see also Chapter 3).80 There are three mammalian hedgehog genes: sonic (Shh), desert (Dhh), and Indian (Ihh), which encode secreted proteins that elicit concentration-dependent responses from target cells.81 Hedgehogs are extracellular proteins that act as signaling ligands. The transmembrane receptors patched (Ptc) and smoothened (Smo) are regulated through this interaction.82 Ptc is a negative regulator of Smo function. When Hh binds to Ptc, Smo is released from the inhibitory effects. This leads to the activation of transcriptional factors.
Indian hedgehog protein stimulates pancreatic growth. In contrast, sonic hedgehog protein expression inhibits pancreatic development and is excluded from developing pancreatic tissue.83 Transgenic mice that overexpress sonic hedgehog demonstrate marked reductions in exocrine and endocrine pancreatic tissue. Indian hedgehog, when blocked in animal models, leads to a reduction in pancreatic size and impaired endocrine cell development in mice. Furthermore, homozygous inactivation of Ihh in mice resulted in the development of an annular pancreas in 42% of the mice.84 Inactivation of sonic hedgehog has also been linked with annular pancreatic development.85 A model for pancreas divisum in mice includes genotypes heterozygous for the null alleles of Shh and Ihh and deficient Smad2.84,86 Shh mutations in humans are associated with gut malrotations and imperforate anus, and with annular pancreas.84,85 The sonic hedgehog gene also functions to inhibit ectopic pancreatic formation in the stomach, duodenum, and liver.
Inhibition of hedgehog signaling in chick embryos leads to ectopic budding of pancreatic structures in the stomach and duodenum.87 This offers a potential explanation of pancreatic heterotopia (pancreatic rests).
The role of undifferentiated cells and their control in pancreatic development again becomes relevant in adults when the same mechanisms influence repair, replacement, and regeneration. Uncontrolled proliferation seen in pancreatic neoplasms has been linked with abnormal hedgehog signaling.82,88 Sonic hedgehog is normally not found in the mature pancreas (as opposed to Indian and desert hedgehog, which are normally expressed).89 Pancreatic cancer is associated with expression of Shh and up-regulation of Ihh. Higher levels of expression of Shh are seen with advancing degrees of atypia. Pancreatic intraepithelial neoplasia (PanIN) type lesions are also seen in transgenic mice overexpressing Shh. PanIN lesions are associated with intraductal papillary mucinous neoplasms (IPMN) of the pancreas and can progress to adenocarcinoma (see Chapter 60).
The oncogenic function of hedgehog proteins has been term a “clever trick” because it does not appear to be directly targeted to tumor cells but rather to nonmalignant stromal cells that, as part of the tumor microenvironment, support tumor growth.90,91 Inhibitors of hedgehog activity may be novel therapies for neoplasia; furthermore, expression of Shh offers a potential means of diagnosing pancreatic adenocarcinoma.
Pancreatic duct primary cilia and hedgehog proteins interface in animal and human cell lines during both pancreatic development and homeostasis. Primary cilia are organelles that act as sensors in the extracellular environment and coordinate multiple signal transduction pathways. Defects in ciliary assembly have been associated with pancreatic cysts, pancreatitis, and diabetes.92 Regulation of the Hh pathway is complex, with Gli transcription factors, effectors of Hh signaling, having either activation or repressor function and localization of these modulating functions to the cilium and cell body. In the developing human pancreas, Hh was linked to a ciliary localization in pancreatic duct epithelial cells. PANC1 and CFPAC-1 are human cancer cells lines derived from an epithelioid carcinoma and a patient with a cystic fibrosis transmembrane regulator (CFTR) mutation 508, respectively. The cilia in the proliferating areas expressed the transmembrane receptors Ptc and Smo. The abnormal cilia have been linked with a marker of proliferative risk.93 Abnormal pancreatic cilia associated with pancreatitis and pancreatic ductal dilation have increased beta-catenin expression, which is associated with activation of the Wnt pathway. Abnormal Wnt signaling has also been noted in cystic kidney disease.
Dorsal and ventral pancreatic buds develop from the endoderm of the foregut and appear as two independent thickenings or anlages.11 These two anlages are not identical, are asynchronous, and receive distinct signals from their surrounding tissues. In order to understand their development further, we must reacquaint ourselves with two terms—notochord and homeobox (Pdx1). Notochord is composed of cells derived from the mesoderm defining the primitive axis of the body, and is the center of development of the axial skeleton. Homeobox refers to a class of highly conserved DNA sequences encoding protein domains involved in binding to DNA. These occur in genes involved in the control of development in humans. The dorsal pancreatic bud develops in proximity to the notochord. As the notochord is displaced by the dorsolateral splanchnic mesenchyme, a variety of new signals emerge from the aorta. Shh activity along with fibroblast growth factor (FGF), transforming growth factor-β (TGF-β), and vascular endothelial growth factor (VEGF) allows homeobox transcription factor (Pdx1) expression to mark duodenal cells and promote the dorsal pancreatic bud. The ventral bud development does not depend on the notochord but instead is closely connected to liver organogenesis. The initial pancreatic endoderm from both areas consist of progenitors of endocrine and exocrine cells. The cells express the homeobox transcription factor Pdx1, which is necessary for pancreatic and duodenal differentiation after the formation of the ventral and dorsal pancreatic buds. Defects that occur in Pdx1-null mice include abnormalities in the gastroduodenal junction, submucosal Brunner’s glands, enteroendocrine cell numbers in the stomach and duodenum, and formation of peribiliary glands and mucin-producing cells in the gallbladder.94–97
The interplay between the morphogenesis and cytodifferentiation is less well understood.98 The availability of a pool of precursor cells involved in maintenance, replacement, and repair of cells offers the potential for hypoproliferative and proliferative disorders. The mesenchymal growth factors and pancreatic epithelium work through the Notch pathway to regulate exocrine and endocrine cell differentiation.99
There are four Notch genes in mammals, each of which encodes a transmembrane receptor. Binding of ligands leads to intracellular cleavage of the Notch receptor with activation of the intracellular domain, allowing interaction with the deoxyribonucleic acid (DNA)–binding protein RBP-Jh. This induces expression of the basic helix-loop-helix HES genes, which down-regulate expression of target genes. The pancreatic anlage epithelium expresses the Notch1 and HES genes. The anlage develops into a branching epithelium, which is believed to be the source of endocrine and exocrine stem cells. Notch2 is expressed in high levels in this branched epithelium.100 Notch signaling defect knockout mice have a marked endocrine cell differentiation with depletion of epithelial precursors and concomitant exocrine hypoplasia.101
The Notch pathway operates as a negative regulator of endodermal endocrine differentiation. The Notch pathway controls expression of HES1, a basic helix-loop-helix gene that represses positive basic helix-loop-helix genes. In HES1-deficient mice, biliary epithelium differentiates into pancreas-like endocrine and exocrine cells, forming acini and islet cells.102 Thus, biliary epithelium has the potential for pancreatic differentiation, and HES1 determines biliary organogenesis by preventing pancreatic differentiation. Notch signaling allows cells to remain in a nondifferentiated proliferative state. It likely has the role in adults of maintaining the undifferentiated pool of pancreatic stem cells that are involved in islet cell turnover, replacement, and organ repair.101 Up-regulation of the Notch pathway has been linked to metaplastic conversion of exocrine cells in adult pancreatic explants encoded with adenovirus-activated Notch. The exocrine epithelium converted to ductal cells, and the precursor population expanded.
DEVELOPMENTAL ANOMALIES
ANNULAR PANCREAS
Annular pancreas is a congenital anomaly in which a portion of the pancreas forms a thin band around the preampullary portion of the duodenum, leading to complete or partial obstruction (Fig. 55-11). The incidence of annular pancreas is estimated to be between 1 in 1000103–105 and 3 in 20,000,106–107 based on retrospective studies of patients undergoing abdominal imaging and autopsy, respectively.
Strong evidence suggests that genetic factors are involved in the pathogenesis of annular pancreas. There are reports of annular pancreas occurring in siblings108 and identical twins.109 Moreover, annular pancreas is more common in patients with other congenital anomalies such as trisomy 21, cardiac defects, malrotation, genitourinary anomalies, and tracheoesophageal fistula.110,111 The role of Hh genes in pancreatic organogenesis is discussed earlier in this chapter. Shh and Ihh gene defects have shown 42% to 85% incidence of annular pancreas in mouse models.112,113
Although annular pancreas is often diagnosed prenatally or during infancy, it is erroneous to consider it solely a disease of infancy. A review by Zyromski and associates revealed a second peak of detection in the fourth through seventh decades of life.111 In this study, symptoms differed dramatically in adults diagnosed with annular pancreas compared to children. Pediatric patients not identified by prenatal ultrasound tended to present with vomiting, typically nonbilious, and feeding intolerance. In adults, the majority presented with abdominal pain, pancreatitis, evidence of biliary obstruction, or with nausea, vomiting, and bloating.111 Diagnosis in children is suspected by findings on abdominal radiographs, ultrasonography, or upper gastrointestinal (GI) series. In adults, computed tomography (CT) scan, magnetic resonance cholangiopancreatography (MRCP), or endoscopic retrograde cholangiopancreatography (ERCP) may be more commonly used. Not infrequently, the diagnosis of annular pancreas is made at laparotomy.
Surgical duodenoduodenostomy appears to be an effective therapeutic approach, and is considered the treatment of choice in pediatric patients and in some adult patients. Complex pancreatic surgery is more likely to be required in adults compared with children.110,111 The risk of pancreaticobiliary neoplasia is significantly increased in adults with annular pancreas.111,114 Thus, ongoing cancer screening and surveillance should be considered in this population.
PANCREAS DIVISUM
Pancreas divisum (PD) results from a failure of the dorsal and ventral pancreatic ducts to fuse during embryogenesis (Fig. 55-12). Thus, the bulk of pancreatic exocrine secretions must drain through the relatively small dorsal duct of Santorini and minor papilla rather than the ventral duct of Wirsung and the major papilla.
Pancreas divisum has been detected in 5% to 10% of the population in autopsy studies and in a similar percentage of patients undergoing ERCP.115–117 However, Cotton reported that 25.6% of adult patients undergoing ERCP for evaluation of unexplained recurrent pancreatitis were found to have pancreas divisum.118 Pancreas divisum can be diagnosed by ERP, endoscopic ultrasound, abdominal CT, or MRCP.
While only a minority of patients with PD develop symptoms due to their altered anatomy, it is becoming clear that endoscopic therapy can relieve symptoms in some patients with acute recurrent and chronic pancreatitis (see Chapters 58 and 59). In a small randomized, controlled trial by Lans and colleagues, 90% of patients with pancreas divisum and acute pancreatitis experienced symptomatic improvement after undergoing dorsal duct stent placement compared with only 11% of control patients with pancreas divisum and pancreatitis not stented.119 More recently, Gerke and associates reported that 73% of patients with pancreas divisum and acute recurrent pancreatitis had short-term improvement from minor papilla sphincterotomy. Clinical improvement was much less likely in patients with pancreatic-type pain rather than true acute recurrent pancreatitis.120
ECTOPIC PANCREATIC TISSUE
Ectopic (heterotopic) pancreatic tissue, often referred to as a pancreatic rest, occurs in 0.55% to 13.7% of the population according to autopsy material, the most common sites being the stomach (Fig. 55-13), duodenum, proximal jejunum, and ileum. Less frequently heterotopic pancreatic tissue can be found in the umbilicus, common bile duct, gallbladder, and Meckel’s diverticula.121 Although rarely clinically significant, ectopic pancreatic tissue has been associated with ulceration and bleeding, intussusception,122 and even adenocarcinoma.123 Treatment is surgical resection when the tissue is symptomatic, but whether to remove ectopic pancreatic tissue that is found incidentally remains controversial.
PANCREATIC AGENESIS
Pancreatic agenesis is a rare condition that can be complete or partial. Complete pancreatic agenesis is mostly fatal as infants are stricken with diabetes and malabsorption, as well as intrauterine growth restriction due to lack of insulin, an important intrauterine growth factor.124,125 Mice homozygous for a targeted mutation in the insulin-promoter-factor 1 gene are born without a pancreas.126 Partial pancreatic agenesis, or agenesis of the dorsal pancreas, may be less significant clinically due to the presence of some functioning pancreatic tissue. Also known as congenital short pancreas, agenesis of the dorsal pancreas has been associated with polysplenia and intestinal malrotation.127 Pancreatic agenesis should be suspected based on clinical findings, and confirmed with MRI. This condition is discussed in further detail, as well as its genetic basis, in Chapter 57.
CONGENITAL CYSTS
Congenital cysts of the pancreas are rare and may be diagnosed at any age, even prenatally. The cysts may be solitary or multiple, and are distinguished from pancreatic pseudocysts by the presence of an epithelial lining. The clinical presentation is variable, ranging from an incidental finding on an imaging study to an abdominal mass, vomiting, biliary obstruction, or acute pancreatitis. Multiple pancreatic cysts tend to occur in patients with associated anomalies and may be seen in systemic disorders such as von Hippel-Landau syndrome or polycystic kidney disease.128 A review of 15 cases of congenital pancreatic cysts revealed that the majority of patients presented before the age of two years, and associated anomalies were found in 30% of cases.129 Congenital pancreatic cysts are more often located in the body and tail of the pancreas than in the head, and surgical therapy consists of total excision when possible. Cysts in the pancreatic head may be addressed using endoscopic or surgical drainage procedures when necessary.
ANOMALOUS PANCREATICOBILIARY UNIONS
Anomalous pancreaticobiliary union (APBU) is a congenital malformation of the confluence of the pancreatic and bile ducts. A common channel for the bile and pancreatic fluid is formed by the absence of a septum between the ducts. When this malunion occurs, the influence of the sphincter of Oddi is lost, allowing reflux of pancreatic exocrine secretions into the biliary system. This places affected patients at increased risk for pancreatitis, choledochal cysts, and neoplasms that manifest in adulthood. A classification for pancreaticobiliary anomalous junction has been proposed by dividing it into three types: a pb type, in which the pancreatic duct appears to join the common bile duct; a bp type, in which the insertion of the bile duct is into the pancreatic duct; and a Y type, in which there is a long common channel measuring greater than 15 mm in length.130,131 The bp and pb types have each been reported to be the most common type in large series.132,133 Approximately 15% of children with recurrent pancreatitis have this disorder.133 Choledochal cysts are frequent with this abnormality (94% and 100% in two series).132,133
The increased risk of biliary tract malignancy in patients with APBU is well documented. Malunions were seen in 62.5% of adults with gallbladder cancer, in 50% of patients with gallbladder adenomyomatosis, and in 33.3% of patients with common bile duct cancer.132 Patients with APBU have increased cellular proliferative activity of the gallbladder mucosa, even in early childhood.134 Although there is controversy concerning management of APBU among patients of various ethnic groups,135–137 given the cancer risk for the patient with a dilated bile duct associated with a pancreaticobiliary malunion, consideration for cholecystectomy, resection of the bile duct, and hepaticojejunostomy may be advised.138
Cano DA, Hebrok M, Zenker M. Pancreatic development and disease. Gastroenterology. 2007;132:745-62. (Ref 125.)
Cano DA, Sekine S, Hebrok M. Primary cilia deletion in pancreatic epithelial cells results in cyst formation and pancreatitis. Gastroenterology. 2006;131:1856-69. (Ref 92.)
Cotton PB. Congenital anomaly of pancreas divisum as cause of obstructive pain and pancreatitis. Gut. 1980;21:105-14. (Ref 118.)
Fogel EL, Zyromski NJ, McHenry L, et al. Annular pancreas in the adult: Experience at a large pancreatobiliary endoscopy center. Gastrointest Endosc. 2006;63:308. (Ref 105.)
Foo FJ, Gill U, Verbeke CS, et al. Ampullary carcinoma associated with an annular pancreas. JOP. 2007;8:50-4. (Ref 114.)
Gerke H, Byrne MF, Stiffler HL, et al. Outcome of endoscopic minor papillotomy in patients with symptomatic pancreas divisum. JOP. 2004;5:122-31. (Ref 120.)
Hebrok M, Kim SK, St. Jacques B, et al. Regulation of pancreas development by hedgehog signaling. Development. 2000;127:4905-13. (Ref 113.)
Jimenez JC, Emil S, Podnon Y, Nguyen N. Annular pancreas in children: A recent decade’s experience. J Pediatr Surg. 2004;39:1654-7. (Ref 110.)
Kapa S, Gleeson FC, Vege SS. Dorsal pancreas agenesis and polysplenia/heterotaxy syndrome: A novel association with aortic coarctation and a review of the literature. JOP. 2007;8:433-7. (Ref 127.)
Matsushita M, Takakuwa H, Matsubayashi Y, et al. Management of anomalous pancreaticobiliary union. Endoscopy. 2005;37:682. (Ref 136.)
Nielsen S, Mollgård K, Clement CA, et al. Characterization of primary cilia and hedgehog signaling during development of the human pancreas and in human pancreatic duct cancer cell lines. Dev Dyn. 2008;237:2039-52. (Ref 93.)
Ramalho-Santos M, Melton DA, McMahon AP. Hedgehog signals regulate multiple aspects of gastrointestinal development. Development. 2000;127:2763-72. (Ref 112.)
Tashiro S, Imaizumi T, Ohkawa H, et al. Committee for registration of the Japanese study group on pancreaticobiliary maljunction: Retrospective and nationwide survey in Japan. J Hepatobiliary Pancreat Surg. 2003;10:345. (Ref 138.)
Zyromski NN, Sandoval JA, Pitt HA, et al. Annular pancreas: Dramatic differences between children and adults. J Am Coll Surg. 2008;206:1019-25. (Ref 111.)
1. Clarke ES. History of gastroenterology. In: Paulson M, editor. Gastroenterologic Medicine. Philadelphia: Lea & Febiger, 1969.
2. Major RH. A History of Medicine. Springfield, Ill: Charles C Thomas; 1954.
3. Heidenhain R. Beitrage zur Kenntnis des Pankreas. Pflugers Arch. 1875;10:557.
4. Basmajian JV. Grant’s Method of Anatomy, 10th ed. Baltimore: Williams & Wilkins; 1980.
5. Clemente CD, editor. Gray’s Anatomy of the Human Body, 30th ed, Philadelphia: Lea & Febiger, 1985.
6. Rottenberg N. Macroscopic and microscopic vasculature of the duodenal-biliary-pancreatic complex. Morphol Embryol. 1989;35:15.
7. Kimura K, Ohto M, Saisho H, et al. Association of gallbladder carcinoma and anomalous pancreaticobiliary ductal union. Gastroenterology. 1985;89:1258.
8. Misra SP, Gulati P, Thorat VK, et al. Pancreaticobiliary ductal union in biliary disease: An endoscopic retrograde cholangiopancreatography study. Gastroenterology. 1989;96:907.
9. Dimagno EP, Shorter RG, Taylor WF, Go VL. Relationships between pancreaticobiliary ductal anatomy and pancreatic ductal and parenchymal histology. Cancer. 1982;49:361.
10. Dowdy GSJr, Waldron GW, Brown WG. Surgical anatomy of the pancreatobiliary ductal system. Arch Surg. 1962;84:229.
11. Newman HF, Weinberg SB, Newman EB, Northrop JD. The papilla of Vater and distal portions of the common bile duct and duct of Wirsung. Surg Gynecol Obstet. 1958;106:687.
12. Stamm BH. Incidence and diagnostic significance of minor pathologic changes in the adult pancreas at autopsy: A systematic study of 112 autopsies in patients without known pancreatic disease. Hum Pathol. 1984;15:677.
13. Sterling JA. The common channel for bile and pancreatic ducts. Surg Gynecol Obstet. 1954;98:420.
14. Kleitsch WP. Anatomy of the pancreas: A study with special reference to the duct system. Arch Surg. 1955;71:795.
15. Skandalakis LJ, Rowe JSJr, Gray SW, Skandalakis JE. Surgical embryology and anatomy of the pancreas. Surg Clin North Am. 1993;73:661.
16. Hastier P, Buckley MJ, Dumas R, et al. A study of the effect of age on pancreatic duct morphology. Gastrointest Endosc. 1998;48:53.
17. Kreel L, Sandin B. Changes in pancreatic morphology associated with aging. Gut. 1973;14:962.
18. Ladas SD, Tassios PS, Giorgiotis K, et al. Pancreatic duct width: Its significance as a diagnostic criterion for pancreatic disease. Hepatogastroenterology. 1993;40:52.
19. Rottenberg N. Macroscopic and microscopic vasculature of the duodenal-biliary-pancreatic complex. Morphol Embryol. 1989;35:15.
20. O’Morchoe CCC. Lymphatic system of the pancreas. Microsc Res Tech. 1997;37:456.
21. Evans BP, Ochsner A. The gross anatomy of the lymphatics of the human pancreas. Surgery. 1954;36:177.
22. Bloom W, Fawcett DW. A Textbook of Histology, 11th ed. Philadelphia: WB Saunders; 1986.
23. Tompkins RK, Traverso LW. The exocrine cells. In: Keynes WM, Keith RG, editors. The Pancreas. New York: Appleton-Century-Crofts; 1981:23.
24. Bockman DE, Boydston WR, Parsa I. Architecture of human pancreas: Implications for early changes in pancreatic disease. Gastroenterology. 1983;85:55.
25. Shibata K, Kobayashi T, Matsuura N, et al. Production of three monoclonal antibodies specifically reactive respectively with the ductal, acinar, and islet cells of the human pancreas. Jpn J Clin Oncol. 1991;21:13.
26. Itzkowitz S, Kjeldsen T, Friera A, et al. Expression of Tn, sialosyl Tn, and T antigens in human pancreas. Gastroenterology. 1991;100:1691.
27. Soon-Shiong P, Tersaki PI, Lanza RP. Immunocytochemical identification of monoclonal antibodies with binding activity to acinar cells but not islets. Pancreas. 1991;6:318.
28. Ermak TH, Rothman SS. Zymogen granules of pancreas decrease in size in response to feeding. Cell Tissue Res. 1981;214:51.
29. Uchiyama Y, Saito K. A morphometric study of 24-hour variations in subcellular structures of the rat pancreatic acinar cell. Cell Tissue Res. 1982;226:609.
30. Bauduin H, Stock C, Vincent D, Grenier JF. Microfilamentous system and secretion of enzyme in the exocrine pancreas: Effect of cytochalasin B. J Cell Biol. 1975;66:165.
31. Metz J, Forssman WG, Ito S. Exocrine pancreas under experimental conditions. III: Membrane and cell junctions in isolated acinar cells. Cell Tissue Res. 1977;177:459.
32. De Lisle RC, Logsdon CD, Hootman SR, Williams JA. Monoclonal antibodies as probes for plasma membrane domains in the exocrine pancreas. J Histochem Cytochem. 1988;36:1043.
33. Nevalainen TJ. Effects of pilocarpine stimulation on rat pancreatic acinar cells: An electron microscopic study with morphometric analysis. Acta Pathol Microbiol Scand Suppl. 1970;210:1.
34. Bolender RP. Stereological analysis of the guinea pig pancreas. I: Analytical model and quantitative description of nonstimulated pancreatic acinar cells. J Cell Biol. 1974;61:269.
35. Amsterdam A, Jamieson JD. Studies on dispersed pancreatic exocrine cells. I: Dissociation technique and morphologic characteristics of separated cells. J Cell Biol. 1974;63:1037.
36. Palade GE. Intracellular aspects of the process of protein synthesis. Science. 1975;189:347.
37. Jamieson JD, Palade GE. Production of secretory proteins in animal cells. In: Brinkley BR, Porter KR, editors. International Cell Biology 1976-1977. New York: Rockefeller University Press, 1977.
38. Hogue-Angeletti R, Xu R-Y, Gonatas JO, et al. Identification of a novel protein (G210) specific to the Golgi apparatus. J Histochem Cytochem. 1989;37:1177.
39. Liebow C, Rothman SS. Distribution of zymogen granule size. Am J Physiol. 1973;225:258.
40. Nadelhaft I. Measurement of the size distribution of zymogen granules from rat pancreas. Biophys J. 1973;13:1014.
41. Greene LJ, Hirs CHW, Palade GE. On the protein composition of bovine pancreatic zymogen granules. J Biol Chem. 1963;238:2054.
42. Keller PJ, Cohen E. Enzymic composition of some cell fractions of bovine pancreas. J Biol Chem. 1961;236:1407.
43. Tartakoff A, Greene LJ, Palade GE. Studies on the guinea pig pancreas: Fractionation and partial characteristics of exocrine proteins. J Biol Chem. 1974;249:7420.
44. Scheele G, Bartelt D, Bieger W. Characterization of human exocrine proteins by two-dimensional isoelectric focusing/sodium dodecyl sulfate gel electrophoresis. Gastroenterology. 1981;80:461.
45. Kraehenbuhl JP, Racine L, Jamieson JD. Immunocytochemical localization of secretory proteins in bovine pancreatic exocrine cells. J Cell Biol. 1977;72:406.
46. Geuze JJ, Slot JW, Tokuyasu KT. Immunocytochemical localization of amylase and chymotrypsinogen in the exocrine pancreatic cell, with special attention to the Golgi complex. J Cell Biol. 1979;82:697.
47. Mroz EA, Lechene C. Pancreatic zymogen granules differ markedly in protein composition. Science. 1986;232:871.
48. Burwen SJ, Rothman SS. Zymogen granules: Osmotic properties, interaction with ions, and some structural implications. Am J Physiol. 1972;222:1177.
49. Rothman SS. The behavior of isolated zymogen granules: pH-dependent release and reassociation of protein. Biochim Biophys Acta. 1971;241:567.
50. Rothman SS. Association of bovine alpha-chymotrypsinogen and trypsinogen with rat zymogen granules. Am J Physiol. 1972;222:1299.
51. Ermak TH, Rothman SS. Internal organization of the zymogen granule: Formation of reticular structures in vitro. J Ultrastruct Res. 1978;64:98.
52. Hokin LE. Isolation of zymogen granules of dog pancreas and a study of their properties. Biochim Biophys Acta. 1955;18:379.
53. Fujita T. Insulo-acinar portal system in the horse pancreas. Arch Histol Jpn. 1973;35:161.
54. Fujita T, Murakami T. Microcirculation of monkey pancreas with special reference to the insulo-acinar portal system: A scanning electron microscope study of vascular casts. Arch Histol Jpn. 1973;35:255.
55. Bonner-Weir S, Orci L. New perspectives on the microvasculature of the islets of Langerhans in the rat. Diabetes. 1982;31:883.
56. Williams JA, Goldfine ID. The insulin-pancreatic acinar axis. Diabetes. 1985;34:980.
57. Lifson N, Kramlinger KG, Mayrand RR, Lender EJ. Blood flow to the rabbit pancreas with special reference to the islets of Langerhans. Gastroenterology. 1980;79:466.
58. Kramer MF, Tan HT. The peri-insular acini of the pancreas of the rat. Z Zellforsch. 1968;86:163.
59. Malaisse-Lagae F, Ravazzola M, Robbercht P, et al. Exocrine pancreas: Evidence for topographic partition of secretory function. Science. 1975;190:795.
60. Stefan Y, Orci L, Malaisse-Lagae F, et al. Quantitation of endocrine cell content in the pancreas of nondiabetic and diabetic humans. Diabetes. 1982;31:694.
61. Orci L. Macro- and micro-domains in the endocrine pancreas. Diabetes. 1982;31:538.
62. Boquist L. The endocrine cells. In: Keynes WM, Keith RG, editors. The Pancreas. New York: Appleton-Century-Crofts; 1981:31.
63. Arey LB. Developmental Anatomy: A Textbook and Laboratory Manual of Embryology, 7th ed. Philadelphia: WB Saunders; 1974.
64. Patten BM. Human Embryology, 3rd ed. New York: McGraw-Hill; 1968.
65. Kleitsch WP. Anatomy of the pancreas: A study with special reference to the duct system. Arch Surg. 1955;71:795.
66. Laitio M, Lev R, Orlic D. The developing human fetal pancreas: An ultrastructural and histochemical study with special reference to exocrine cells. J Anat. 1974;117:619.
67. Parsa I, Marsh WH, Fitzgerald PJ. Pancreas acinar cell differentiation. I: Morphologic and enzymatic comparisons of embryonic rat pancreas and pancreatic anlage grown in organ culture. Am J Pathol. 1969;57:457.
68. Pictet RL, Clark WR, Williams RH, Rutter WJ. An ultrastructural analysis of the developing embryonic pancreas. Dev Biol. 1972;29:436.
69. Ermak TH, Rothman SS. Increase in zymogen granule volume accounts for increase in volume density during prenatal development of pancreas. Anat Rec. 1983;207:487.
70. Uchiyama Y, Watanabe M. A morphometric study of developing pancreatic acinar cells of rats during prenatal life. Cell Tissue Res. 1984;237:117.
71. Rutter WJ, Kemp JD, Bradshaw WS, et al. Regulation of specific protein synthesis in cytodifferentiation. J Cell Physiol. 1968;72:1.
72. Sanders TG, Rutter WJ. The developmental regulation of amylolytic and proteolytic enzymes in the embryonic rat pancreas. J Biol Chem. 1974;249:3500.
73. Ermak TH, Rothman SS. Large decrease in zymogen granule size in the postnatal rat pancreas. J Ultrastruct Res. 1980;70:242.
74. Uchiyama Y, Watanabe M. Morphonometric and fine structural studies of rat pancreatic acinar cells during early postnatal life. Cell Tissue Res. 1984;237:123.
75. Descholdt-Lanckman M, Robberecht P, Camus J, et al. Hormonal and dietary adaptation of rat pancreatic hydrolases before and after weaning. Am J Physiol. 1974;226:39.
76. Robberecht P, Descholdt-Lanckman M, Camus J, et al. Rat pancreatic hydrolases from birth to weaning and dietary adaptation after weaning. Am J Anat. 1971;221:376.
77. Like AA, Orci L. Embryogenesis of the human pancreatic islets: A light and electron microscopic study. Diabetes. 1972;21:511.
78. Baxter-Grillo D, Blazquez E, Grillo TAI, et al. Functional development of the pancreatic islets. In: Cooperstein SJ, Watkins D, editors. The Islets of Langerhans. New York: Academic Press; 1981:35.
79. Stefan Y, Grasso S, Perrelet A, Orci L. A quantitative immunofluorescent study of the endocrine cell populations in the developing human pancreas. Diabetes. 1983;32:293.
80. McMahon AP, Ingham PW, Tabin CJ. Developmental roles and clinical significance of hedgehog signaling. Curr Top Dev Biol. 2003;53:1.
81. Ingham PW, McMahon AP. Hedgehog signaling in animal development: Paradigms and principles. Genes Dev. 2001;15:3059.
82. Kayed H, Kleff J, Keleg S, et al. Indian hedgehog signaling pathway: Expression and regulation in pancreatic cancer. Int J Cancer. 2004;110:668.
83. Kawahira H, Ma NH, Tzanakakis ES, et al. Combined activities of hedgehog signaling inhibitors regulate pancreas development. Development. 2003;130:4871.
84. Kim SK, Hebrok M. Intercellular signals regulating pancreas development and function. Genes Dev. 2001;15:111.
85. Hebrok M, Kim SK, St Jacques B, et al. Regulation of pancreas development by hedgehog signaling. Development. 2000;127:4905.
86. Ramalho-Santos M, Melton DA, McMahon AP. Hedgehog signals regulate multiple aspects of gastrointestinal development. Development. 2000;127:2763.
87. Kim SK, Melton DA. Pancreas development is promoted by cyclopamine, a hedgehog signaling inhibitor. Proc Natl Acad Sci U S A. 1998;95:13036.
88. Kayed H, Kleff J, Keleg S, et al. Indian hedgehog signaling pathway: Expression and regulation in pancreatic cancer. Int J Cancer. 2004;110:668.
89. Thayer SP, di Magliano MP, Heiser PW, et al. Hedgehog is an early and late mediator of pancreatic cancer tumorigenesis. Nature. 2003;425:851.
90. Curran T, Ng JM. Cancer: Hedgehog’s other great trick. Nature. 2008;455:293-4.
91. Yauch RL, Gould SE, Scales SJ, et al. A paracrine requirement for signalling in cancer. Nature. 2008;455:406-10.
92. Cano DA, Sekine S, Hebrok M. Primary cilia deletion in pancreatic epithelial cells results in cyst formation and pancreatitis. Gastroenterology. 2006;131:1856-69.
93. Nielsen S, Mollgård K, Clement CA, et al. Characterization of primary cilia and hedgehog signaling during development of the human pancreas and in human pancreatic duct cancer cell lines. Dev Dyn. 2008;237:2039-52.
94. Burlison JS, Long Q, Fujitani Y, et al. Pdx-1 and Ptf1a concurrently determine fate specification of pancreatic multi-potential progenitor cells. Dev Biol. 2008;316:74-86.
95. Fukuda A, Kawaguchi Y, Kodama S, et al. Loss of the major duodenal papilla results in brown pigment biliary stone formation in pdx1 null mice. Gastroenterology. 2006;130:855-67.
96. Jonsson J, Carlsson L, Edlund T, Edlund H. Insulin-promoter-factor 1 is required for pancreas development in mice. Nature. 1994;371:606-9.
97. Offield MF, Jetton TL, Labosky PA, et al. PDX-1 is required for pancreatic outgrowth and differentiation of the rostral duodenum. Development. 1996;122:983-95.
98. Johansson KA, Grapin-Botton A. Development and disease of the pancreas. Clin Genet. 2002;62:14.
99. Heiser PW, Hebrok M. Development and cancer: Lessons learned in the pancreas. Cell Cycle. 2004;3:270.
100. Jensen J, Pedersen EE, Galante P, et al. Control of endodermal endocrine development by Hes-1. Nature Genet. 2000;24:36.
101. Sumazaki R, Shiojiri N, Isoyoma S, et al. Conversion of biliary system to pancreatic tissue in Hes-1 deficient mice. Nature Genet. 2004;36:83.
102. Hald J, Hjorth JP, German MS, et al. Activated Notch 1 prevents differentiation of pancreatic acinar cells and attenuates endocrine development. Dev Biol. 2003;260:426.
103. Chevillotte G, Sahel J, Raillat A, Sarles H. Annular pancreas. Report of one case associated with acute pancreatitis and diagnosed by endoscopic retrograde pancreatography. Dig Dis Sci. 1984;29:75-7.
104. Glazer GM, Margulis AR. Annular pancreas: Etiology and diagnosis using endoscopic retrograde cholangiopancreatography. Radiology. 1979;133:303-6.
105. Fogel EL, Zyromski NJ, McHenry L, et al. Annular pancreas in the adult: Experience at a large pancreatobiliary endoscopy center. Gastrointest Endosc. 2006;63:308.
106. Ravitch MM, Woods ACJr. Annular pancreas. Ann Surg. 1950;132:1116-27.
107. Theodorides T. Annular pancreas. J Chir (Paris). 1964;87:445-62.
108. Lainakis N, Antypas S, Panagidis A, et al. Annular pancreas in two consecutive siblings: An extremely rare case. Eur J Pediatr Surg. 2005;15:364-8.
109. Kumar RB, Hulvat MC, Newman BM, Muraskas JK. Annular pancreas in identical twin newborns. J Pediatr Surg. 2006;41:E19-E21.
110. Jimenez JC, Emil S, Podnon Y, Nguyen N. Annular pancreas in children: A recent decade’s experience. J Pediatr Surg. 2004;39:1654-7.
111. Zyromski NN, Sandoval JA, Pitt HA, et al. Annular pancreas: Dramatic differences between children and adults. J Am Coll Surg. 2008;206:1019-25.
112. Ramalho-Santos M, Melton DA, McMahon AP. Hedgehog signals regulate multiple aspects of gastrointestinal development. Development. 2000;127:2763-72.
113. Hebrok M, Kim SK, St. Jacques B, et al. Regulation of pancreas development by hedgehog signaling. Development. 2000;127:4905-13.
114. Foo FJ, Gill U, Verbeke CS, et al. Ampullary carcinoma associated with an annular pancreas. JOP. 2007;8:50-4.
115. Kleitsch WP. Anatomy of the pancreas: A study with special reference to the duct system. Arch Surg. 1955;71:795-802.
116. Dawson W, Langman V. An anatomical-radiological study of the pancreatic duct pattern in man. Anat Rec. 1961;139:59-68.
117. Smanio T. Proposed nomenclature and classification of the human pancreatic ducts and duodenal papillae: study based on 200 postmortems. Int Surg. 1969;52:125.
118. Cotton PB. Congenital anomaly of pancreas divisum as cause of obstructive pain and pancreatitis. Gut. 1980;21:105-14.
119. Lans JI, Geenen JE, Johanson JF, Hogan WJ. Endoscopic therapy in patients with pancreas divisum and acute pancreatitis: A prospective, randomized, controlled clinical trial. Gastrointest Endosc. 1992;38:430-4.
120. Gerke H, Byrne MF, Stiffler HL, et al. Outcome of endoscopic minor papillotomy in patients with symptomatic pancreas divisum. JOP. 2004;5:122-31.
121. Dolan RV, ReMine WH, Dockerty MB. The fate of heterotopic pancreatic tissue. Arch Surg. 1974;109:762.
122. Chandra N, Campbell S, Gibson M, et al. Intussusception caused by a heterotopic pancreas. Case report and literature review. JOP. 2004;5:476-79.
123. Jeong HY, Yang HW, Seo SW, et al. Adenocarcinoma arising from an ectopic pancreas in the stomach. Endoscopy. 2002;34:1014-17.
124. Winter WE, Maclaren NK, Riley WJ, et al. Congenital pancreatic hypoplasia: A syndrome of exocrine and endocrine pancreatic insufficiency. J Pediatr. 1986;109:465-8.
125. Cano DA, Hebrok M, Zenker M. Pancreatic development and disease. Gastroenterology. 2007;132:745-62.
126. Jonsson J, Carlsson L, Edlund T, et al. Insulin-promoter-factor 1 is required for pancreas development in mice. Nature. 1994;371:606-9.
127. Kapa S, Gleeson FC, Vege SS. Dorsal pancreas agenesis and polysplenia/heterotaxy syndrome: A novel association with aortic coarctation and a review of the literature. JOP. 2007;8:433-7.
128. Boulanger SC, Borowitz DS, Fisher Jf, Brisseau GF. Congenital pancreatic cysts in children. J Pediatr Surg. 2003;38:1080-82.
129. Auringer ST, Ulmer JL, Sumner TE, Turner CS. Congenital cyst of the pancreas. J Pediatr Surg. 1993;28:1570.
130. Kimura K, Ohto M, Saiho H, et al. Association of gallbladder carcinoma and anomalous pancreaticobiliary ductal union. Gastroenterology. 1985;89:1258.
131. Guelrud MG, Carr-Locke DL, Fox VL. ERCP in Pediatric Practice: Diagnosis and Treatment. Oxford: Isis Medical Medica; 1997. p 22
132. Wang HP, Wu MS, Lin CC, et al. Pancreaticobiliary diseases associated with anomalous pancreaticobiliary ductal union. Gastrointest Endosc. 1998;48:184.
133. Guelrud M, Morera C, Rodriguez M, et al. Normal and anomalous pancreaticobiliary union in children and adolescents. Gastrointest Endosc. 1999;50:189.
134. Tokiwa K, Iwai N. Early mucosal changes of the gallbladder in patients with anomalous arrangement of the pancreaticobiliary duct. Gastroenterology. 1996;110:1614-18.
135. Samavedy R, Sherman S, Lehman G. Endoscopic therapy in anomalous pancreatobiliary duct junction. Gastrointest Endosc. 1999;50:623-7.
136. Matsushita M, Takakuwa H, Matsubayashi Y, et al. Management of anomalous pancreaticobiliary union. Endoscopy. 2005;37:682.
137. Cicek B, Parlak E, Koksal A, et al. Regarding the management of anomalous pancreaticobiliary union. Endoscopy. 2005;37:683.
138. Tashiro S, Imaizumi T, Ohkawa H, et al. Committee for registration of the Japanese study group on pancreaticobiliary maljunction: Retrospective and nationwide survey in Japan. J Hepatobiliary Pancreat Surg. 2003;10:345.