Chapter 15 Abnormalities of the Optic Nerve and Retina
Disorders of the optic nerve and retina are common causes of afferent visual loss in clinical neurology. The diagnosis of optic neuropathy should be considered when visual loss (affecting visual acuity, color vision, or visual field) is accompanied by abnormal optic disc appearance or a relative afferent pupillary defect (RAPD; see Chapter 17). The specific cause for an optic neuropathy often can be established on the basis of clinical history (i.e., character, progression of vision loss) and examination (i.e., pattern of visual field loss and optic disc appearance). Furthermore, optic neuropathies are classifiable by appearance of the optic disc: normal, swollen, or pale. Chapter 14 describes the various patterns of visual field loss and clinical history typically elicited in patients with specific optic nerve disorders. This chapter presents the differential diagnosis for optic neuropathies based on the optic disc appearance and discusses retinal disorders of particular interest in neurology. Chapter 36 discusses many of the entities described in this chapter in more detail.
Optic Nerve Anatomy and Physiology
Light stimulates retinal photoreceptors whose signal reaches a ganglion cell after being modulated by bipolar, horizontal, and amacrine cells (Fig. 15.1). Two main types of retinal ganglion cells exist: parasol cells (which project to the magnocellular layer and are specialized for motion perception and coarse stereopsis) and midget cells (which project to the parvocellular layer and are specialized for high spatial resolution, color vision, and fine stereopsis). Temporal retinal fibers form arcuate bundles around the fovea, respecting the midline horizontal raphe, and then enter the optic disc superiorly and inferiorly (Fig. 15.2). Optic nerve fibers exit the globe at the scleral canal, where they receive physical support from the lamina cribrosa and receive metabolic support from intertwining astrocytes. Once nerve fibers pass the lamina cribrosa, they are supported by oligodendrocytes and become myelinated. After exiting the orbit, the nerve enters the optic canal within the lesser sphenoid wing. In this space, the nerve is particularly vulnerable to trauma or compressive lesions (Balcer, 2001; Sarkies, 2004).
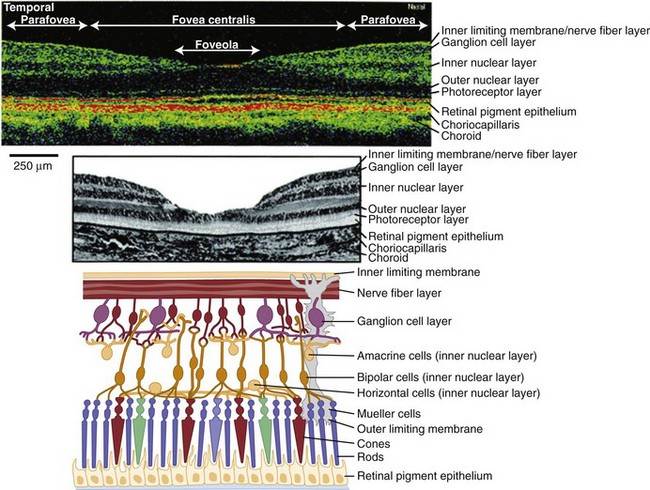
(Adapted and reprinted with permission from Jaffe and Caprioli [2004] and www.webvision.med.utah.edu.)
Effective axonal transport is essential for maintenance of the ganglion cell axon’s structure and function. Orthograde transport (away from the ganglion cell body) occurs at two speeds: 400 mm per day for proteins and neurotransmitters packaged in vesicles, and 1 to 4 mm per day for structural elements of the cytoskeleton. Interference of axonal transport, for example from elevated intracranial pressure (ICP), ultimately damages axons of the optic nerve and causes atrophy (Hayreh, 1977).
The ophthalmic artery, arising from the internal carotid artery, provides blood supply to the eye via multiple short posterior ciliary arteries and the central retinal artery (Hayreh and Zimmerman, 2007) (Fig. 15.3). The short posterior ciliary arteries provide blood supply to the optic nerve head and the subretinal choroid. Each posterior ciliary artery supplies a variable segmental territory of the optic nerve head, and because anastomoses in this blood supply are scant, it can suffer watershed ischemia during hypoperfusion. Furthermore, the segmental blood supply underlies the sectoral disc swelling or atrophy that results from interrupted flow of a posterior ciliary artery and subsequent optic nerve infarction (Balcer, 2001; Fontal et al., 2007).
The Swollen Optic Disc
Unilateral Optic Disc Swelling
Despite suggestive patterns in the appearance of the optic nerve, it often is not possible to distinguish NAION, optic neuritis, and compressive optic neuropathies on this basis alone. Typically these diagnoses also rely on data from the clinical history and the pattern of the visual field deficit (Fig. 15.4). Vision loss generally is slowly progressive in patients with compressive lesions; it is rapidly progressive with subsequent improvement in those with optic neuritis; and it is maximal at onset with minimal improvement in patients with NAION. Both optic neuritis and compressive lesions generally produce central visual loss, whereas NAION typically produces a nerve fiber bundle–type field defect (originating from the physiological blind spot and respecting the horizontal meridian, owing to the arrangement of retinal ganglion cell axons traveling to the optic disc). However, considerable overlap exists in the patterns of visual field loss caused by the different forms of optic neuropathy.
Optic Neuritis
Typical optic neuritis is an inflammatory optic neuropathy caused by demyelinating disease (Balcer, 2006) (Fig. 15.5). Visual loss in the affected eye typically occurs rapidly over several hours to a few days. Decreased color vision and contrast sensitivity are highly characteristic (Baier et al., 2005; Trobe et al., 1996). In addition, pain with eye movements precedes the vision loss in approximately 90% of cases (Optic Neuritis Study Group, 1991). The pain typically lasts 3 to 5 days; if it persists for longer than 7 days, optic neuritis should be considered less likely, and further workup should be pursued. Visual field defects commonly are present; they can be either diffuse or discrete scotomas and are nonspecific. Fundus examination reveals mild disc swelling in approximately one-third of affected eyes, which is considerably less prominent than the disc swelling associated with papilledema (Balcer, 2006; Beck, 1998) (Fig. 15.6). In the majority of patients, the fundus appearance is normal.
The prognosis for recovery of vision generally is good but is in relation to the severity of the initial deficit. Recovery typically begins within 1 month. The likelihood of progression of optic neuritis to multiple sclerosis (MS) is best predicted by brain magnetic resonance imaging (MRI) at the time of diagnosis. In the Optic Neuritis Treatment Trial, the risk of developing MS within 15 years was 72% among patients with one or more characteristic brain lesions, whereas it was 25% if the MRI was normal (Optic Neuritis Study Group, 2008). With features that are atypical for optic neuritis, however (e.g., painless visual loss, severe disc edema, disc or peripapillary hemorrhages, macular exudate), the risk of developing MS is significantly lower (Beck et al., 2003).
Following an episode of optic neuritis, the optic nerve often demonstrates pallor, suggesting that axonal loss has accompanied the episode of demyelination (Fig. 15.7). Optical coherence tomography (OCT) is a noninvasive imaging method that quantifies the atrophy of the nerve fiber layer (Balcer, 2006). It provides a reliable structural marker that complements clinical assessments of visual function.
Neuromyelitis optica (NMO), or Devic disease, is characterized by necrotizing demyelinating lesions of bilateral optic nerves and the spinal cord (Wingerchuk, 2006). It is believed to be a humorally mediated disease distinct from MS. The spinal lesion characteristic of NMO often extends contiguously over three or more vertebral segments. A serum antibody, neuromyelitis optica immunoglobulin G (NMO-IgG), which targets the autoantigen aquaporin 4 (AQP4), may be a useful marker in diagnosing the condition, although the exact specificity remains unknown (Jarius et al., 2008). Treatment with rituximab, a chemotherapeutic monoclonal antibody, may be of particular benefit in this group of patients (Wingerchuk, 2006).
Treating optic neuritis with high-dose intravenous (IV) corticosteroids reduces the risk of developing MS over the following 2 years (Beck et al., 1992). In the long term, however, this acute treatment is unlikely to affect the likelihood of progression to MS. In addition, IV corticosteroid treatment may hasten visual recovery, particularly for visual fields and contrast sensitivity, but does not significantly affect long-term visual outcomes. Because low-dose oral corticosteroids may be associated with an increased risk of recurrence of optic neuritis, this therapy should be avoided (Beck et al., 1992). In addition to IV corticosteroids, recent studies support the early use of immunomodulating treatments for high-risk patients to reduce the likelihood of progression to MS within 2 to 5 years (Balcer, 2006).
Ischemic Optic Neuropathy
GCA typically affects the extracranial medium- to large-caliber arteries, because they possess elastic lamina, which is the initial site of inflammation in this disorder (Salvarani et al., 2008). The condition is associated with polymyalgia rheumatica, consisting of proximal muscle ache, arthralgia, and stiffness, as well as with jaw claudication, fever, malaise, and scalp tenderness. The diagnosis is suggested by an elevated erythrocyte sedimentation rate and C-reactive protein and is confirmed by evidence of giant cells and endovascular inflammation on temporal artery biopsy. Acute vision loss is the presenting symptom in 7% to 60% of cases and is generally more severe than in NAION. In approximately 25% of cases, vision is limited to hand motion perception or worse (Balcer et al., 2003). In suspected cases, treatment with corticosteroids should not be delayed until a biopsy is obtained. Intraveous corticosteroids may help delay the progression of visual loss and decrease the likelihood of fellow eye involvement. The prognosis for recovery in the affected eye, however, is poor despite treatment (Hall and Balcer, 2004).
The optic disc in AAION typically has a chalky-white edematous appearance, and disc hemorrhages are likely to be present (Fig. 15.8). Coexisting retinal ischemia with cotton-wool spots is very typical for AAION. Fluorescein angiography reveals choroidal hypoperfusion (Fig. 15.9). Occasionally, GCA can be limited to the retro-orbital nerve and present without disc swelling; this situation is termed arteritic posterior ischemic optic neuropathy (PION).
Nonarteritic AION is the most common cause of unilateral optic nerve swelling in adults older than 50 and is commonly associated with vascular risk factors such as diabetes or hypertension (Fontal et al., 2007). Other risk factors include a crowded optic nerve head and nocturnal hypotension, possibly precipitated by antihypertensive therapy (Arnold, 2003; Mathews, 2005). Swelling of a crowded optic nerve within the scleral canal may provoke a cycle of further vascular compression, ischemia, and swelling.
Although the clinical profile of NAION may occasionally overlap with the findings of optic neuritis (Rizzo et al., 1991), typical features of NAION include nerve fiber hemorrhages, altitudinal visual field loss, moderate to severe disc edema, and the absence of pain (Figs. 15.10 and 15.11). Because the optic nerve head is supplied by an end-arterial system of short posterior ciliary arteries and the circle of Zinn-Haller, sectoral ischemic disc swelling is common. NAION may follow ocular surgery, because an associated increase in intraocular pressure may compromise optic nerve head perfusion (Fontal et al., 2007).
Many patients with NAION will have a stable deficit, although one minority may experience visual loss progressing over one month. Spontaneous improvement may occur in the first 6 months, although in many patients this reflects improved ability with eccentric fixation (Hayreh and Zimmerman, 2008). In 30% to 40% of patients, subsequent involvement of the fellow eye also occurs, and this rate is increased by the presence of vascular risk factors. When the second eye is affected in AION, optic atrophy has already developed in one eye, and acute disc edema occurs in the fellow eye; this clinical presentation is called the pseudo–Foster Kennedy syndrome. (A true Foster Kennedy syndrome is produced by optic atrophy due to compression, typically from an expanding tumor, and papilledema in the fellow eye secondary to increased ICP.) Occasionally, premonitory disc swelling in an asymptomatic eye will be noted, which may progress to frank visual loss or remit spontaneously (Hayreh and Zimmerman, 2007). Recurrence of NAION in an affected eye, however, is rare, possibly because optic nerve atrophy following the initial event decompresses the nerve. There does not appear to be a significantly higher rate of stroke in patients with nonarteritic ischemic optic neuropathy, suggesting that its pathophysiology may differ from simple vasoocclusion (Arnold and Levin, 2002).
Other Causes
Inflammatory conditions are an important cause of subacute optic neuropathy. Optic discs may appear swollen or normal, the latter indicating retrobulbar involvement. Optic nerve involvement is common in neurosarcoidosis, which can be accompanied by anterior uveitis or posterior segment vitritis (Prasad et al., 2008) (Fig. 15.12). Visual loss due to this condition is often steroid responsive. Optic neuropathy and retinal involvement may also occur with other inflammatory disorders, such as systemic lupus erythematosus and Sjögren disease (Fig. 15.13). Occasionally, optic nerve infiltration produces optic disc edema without affecting visual function, but more often there is a decrease in visual acuity and visual field loss.
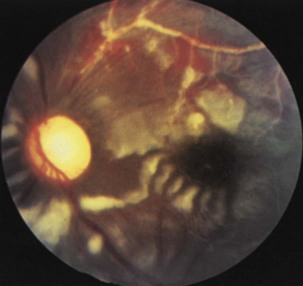
Fig. 15.13 Optic disc pallor and multiple cotton-wool spots in a patient with systemic lupus erythematosus.
Infectious conditions are another frequent cause of optic neuropathy (March and Lessell, 1996). Neuroretinitis, in which optic neuropathy coexists with characteristic peripapillary or macular exudates, should be distinguished from acute demyelinating optic neuritis (Fig. 15.14