History
An 82-year-old woman who had a myocardial infarction and a coronary artery bypass graft (CABG) in 1997 developed progressive dyspnea and limitation of exercise tolerance to 200 yards (New York Heart Association [NYHA] class III) in 2010. After the diagnosis of systolic heart failure, she was started on medical therapy, but developed bronchoconstriction with beta blockers and a cough on angiotensin-converting enzyme (ACE) inhibitors, and she could tolerate only low doses of an angiotensin receptor blocker. Her medical history included permanent atrial fibrillation and peripheral vascular disease.
Comments
The patient was symptomatic from systolic heart failure and intolerant to medical therapy.
Current Medications
The patient was taking clopidogrel 75 mg daily, furosemide 40 mg daily, losartan 50 mg daily, rosuvastatin 10 mg daily, and isosorbide mononitrate XL 60 mg daily.
Comments
The patient experienced bronchoconstriction while on beta blockers, developed a cough on ACE inhibitors, and was intolerant of doses of losartan higher than 50 mg daily.
Current Symptoms
The patient was in NYHA class III. Her exercise capacity was limited at 200 yards by dyspnea, and she had no angina.
Comments
Dyspnea is the main symptom. The patient rarely experienced intermittent claudication.
Physical Examination
Electrocardiogram
Findings
The electrocardiogram showed atrial fibrillation with a ventricular response of 97 bpm and a QRS duration of 134 ms (Figure 37-1). There was fragmentation of the QRS complex in leads II, III, aVF and V3, with no evidence of a bundle branch block.
Comments
The patient had a high ventricular rate at rest, in the background of intolerance to beta blockers. The QRS duration is in keeping with electrical dyssynchrony. The high ventricular rate raises the possibility of tachyarrhythmia-related left ventricular dysfunction.
Chest Radiograph
Findings
The chest radiograph revealed an increased cardiothoracic ratio, no evidence of pulmonary edema, and sternotomy wires (Figure 37-2).
Comments
The findings on chest radiography were in keeping with heart failure without pulmonary edema.
Echocardiogram
Findings
The echocardiogram showed global left ventricular hypokinesia, inferior akinesis, myocardial thinning at the apex, a pseudospherical left ventricle with severely impaired left ventricular function (LVEF of 29% using Simpson’s method), and biatrial dilation (Figure 37-3, A).
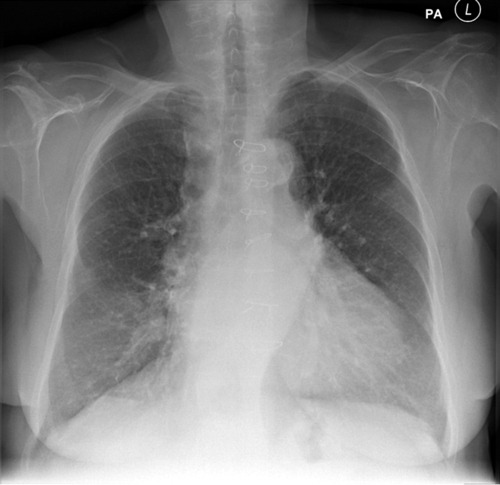
FIGURE 37-2 Pre-implant chest radiograph.
Comments
The findings on echocardiography were in keeping with ischemic cardiomyopathy.
Findings
The transaortic peak gradient on the echocardiogram was 17 mm Hg, and the mean gradient was 14 mm Hg (see Figure 37-3, B).
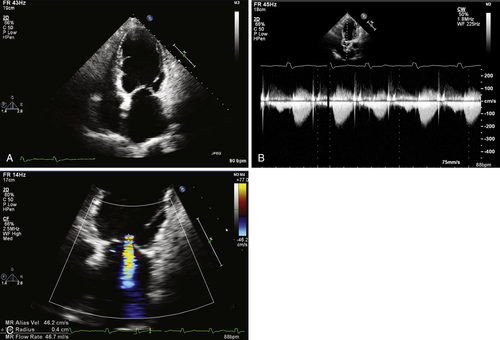
FIGURE 37-3 Pre-implant transthoracic echocardiogram showing an apical. A, Apical four-chamber view. B, Continuous wave Doppler image through aortic valve. C, Color Doppler image through mitral valve.
Comments
The gradient was consistent with mild aortic stenosis (see Figure 37-3, B). In the background of severely impaired left ventricular function, the severity of aortic stenosis may be underestimated.
Findings
The mitral regurgitant jet occupied 36% of the left atrial area on the four-chamber view. The proximal isovelocity hemispheric surface area radius was 0.4 cm and the effective regurgitant orifice was 0.2 cm2. The valvular leaflets were intact, and dilation of the mitral valve ring and biatrial dilation were present.
Comments
The findings are consistent with moderate mitral regurgitation. The presence of inferior hypokinesis and a previous myocardial infarction raises the possibility of ischemic mitral regurgitation resulting from involvement of the posterior papillary muscle (mitral valve apparatus). Functional dilatation of the mitral valve ring also contributes to the degree of regurgitation.
Coronary Sinus Venography
Right anterior oblique (RAO) and left anterior oblique (LAO) fluoroscopic coronary sinus venography was performed at the time of cardiac resynchronization therapy (CRT) device implantation, showing veins that might be considered options for left ventricular lead deployment (Figure 37-4, A).
Findings
Option 1 in Figure 37-4 is a small-caliber, posterolateral vein draining the mid-lateral segment. This vein has a stenosis in its proximal, tortuous portion (see Figure 37-4, insert, white arrow). Option 2 is a posterolateral vein draining the mid-lateral segment. Option 3 is a small-caliber, anterolateral vein draining the basal anterior segment.
Comments
Option 1 in Figure 37-4 appears to be a reasonable candidate vein for left ventricular lead deployment. A venoplasty could be performed in the proximal portion and the vein then straightened using a buddy wire technique. A small-caliber left ventricular lead could then be deployed distally, in the mid-lateral segment.
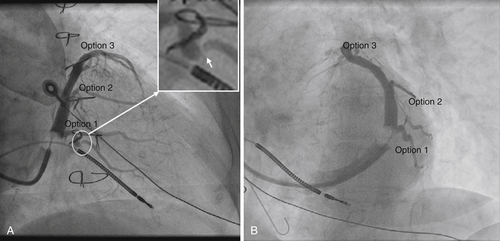
FIGURE 37-4 Coronary sinus venography at implantation. A, Right anterior oblique projection. B, Left anterior oblique projection.
Feature Tracking Cardiovascular Magnetic Resonance Imaging
Findings
Feature tracking of the basal slice shows that the latest contracting segments in the lateral free left ventricular wall are the basal posterior and the basal inferior segments (Figure 37-5, A, red arrows ). Peak circumferential strain in these segments, however, is less than 10%, suggesting myocardial scar. The basal lateral segment appears to not be scarred (peak circumferential strain, −
Buy Membership for Cardiovascular Category to continue reading. Learn more here