Emergency Imaging
Edited by George Jelinek
23.1 Emergency department ultrasound
James Rippey, Adrian Goudie and Andrew Haig
Background
Clinical ultrasound followed developments from the use of sonar, where the principle that sound waves could be used to locate objects was developed. Initially, ultrasound machines were large and cumbersome, but advances in technology have improved image quality while reducing machine size, so that today, small machines are able to produce high quality images. As a result of this improved technology, ultrasound is now available to clinicians and can be performed at the bedside of patients. There was early adoption of the technique by obstetricians and gynaecologists worldwide and later by other specialties in Europe and Japan. Ultrasound is now used by many different specialties in most countries.
Clinician performed ultrasound has a different approach than formal diagnostic ultrasound, such as that performed in radiology departments. Clinician performed ultrasound is generally limited in scope and targeted to answering a specific question (such as ‘is there an abdominal aortic aneurysm’), rather than providing a full assessment of an anatomical area (Table 23.1.1). In this regard, it is often viewed more as an extension of the clinical exam, than a technique that competes with other imaging techniques (including formal ultrasound).
Table 23.1.1
Current indications for emergency ultrasound
Trauma (haemoperitoneum, haemopericardium, pneumothorax)
Abdominal aortic aneurysm
Early pregnancy complications
Biliary disease
Renal stones and hydronephrosis
Echocardiography in trauma and shock
Lung ultrasound in acute dyspnoea
Proximal DVT exclusion
Procedural
Musculoskeletal
The Australasian College for Emergency Medicine supports the use of bedside ultrasound by emergency physicians [1], as does the American College of Emergency Physicians, the College of Emergency Medicine in the UK and the International Federation for Emergency Medicine, where it is seen as a core skill required of all trainees. It is expected that with increasing experience the range of conditions for which ultrasound is used in emergency departments (EDs) will increase.
Basic physics of ultrasound [2]
Sound waves are mechanical waves that transmit energy through the vibration of particles. Ultrasound waves are defined as those that are above the usual range of human hearing (20–20 000 Hz). Current diagnostic ultrasound machines are based upon the pulse–echo principle, using pulses of sound waves at frequencies of 2–15 MHz which are reflected back. Processing of these reflected echoes creates the ultrasound data and image.
The ultrasound transducer converts electrical impulses into pulses of sound (via the piezoelectric effect) which are then directed into the body. As the sound wave travels through tissue, it gradually loses energy, termed attenuation. The degree of attenuation differs for different tissues and is also dependent on the frequency of the pulse wave. Upon reaching a tissue interface, some of the energy is reflected back as an echo, due to the differences in acoustic impedance (gel or other coupling material is used to minimize reflection at the probe/skin surface). This reflected echo then travels back through tissue, undergoing further attenuation, until it reaches the transducer, which converts the energy back to an electrical impulse. This returning impulse is then amplified and processed. The time taken for the pulse wave to travel to the tissue interface and back is converted into distance by using the average speed for sound in tissue. The intensity of the returning wave determines the brightness of the displayed pixel. The returning pulses from the different reflecting surfaces along the path of the ultrasound beam generate a single line of the ultrasound image. The ultrasound beam is steered across the field to generate the multiple lines of information that then form the 2D image (termed B-mode, for brightness modulation). Alternatively, if the direction of the beam is kept constant and the changing surfaces are mapped over time then an M-mode image is generated.
The degree of attenuation is dependent on the frequency of the sound wave, so higher frequency pulses undergo greater attenuation. They also have shorter wavelengths, which improve the resolution of the ultrasound beam (the ability to distinguish two separate objects close together). This leads to one of the most important trade-offs in ultrasound, between resolution and penetration. To obtain high resolution, a high frequency probe can be chosen, but these will be unable to image deep structures.
To form the image, the ultrasound machine makes certain assumptions about the ultrasound beam and sound impulse. Deviations from these behaviours will result in image artefacts, i.e. when the image does not represent the tissue accurately. There are many artefacts, most of which reduce the information available from the image. The most clinically important artefacts, however, can also be used diagnostically:
Shadowing – when all of the energy of the ultrasound pulse is reflected or absorbed at a surface (such as air or bone). In this situation, there will be no returning pulses from the tissue distal to the object. This creates a black area on the screen, known as an acoustic shadow. The presence of a shadow behind a brightly reflective surface can thus be used to diagnose a region of calcification, such as a calculus (Fig. 23.1.1). Stones and bones generally give ‘clean’ or black shadows, while gas gives ‘dirty’ or grey shadows due to the superposition of both shadow and reverberation artefact (Fig. 23.1.2).
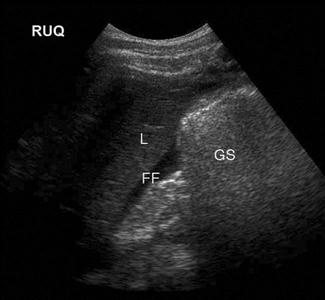
Enhancement – when an area of interest (such as fluid in a cyst) absorbs less energy than the surrounding tissue, the pulses that have travelled through that area will have more energy than equidistant pulses that did not, resulting in a bright region deep to the area of interest on the image (Fig. 23.1.3). Enhancement is used to confirm the fluid-filled nature of lesions.
Transducer
Different ultrasound transducers are available varying in shape, frequency and the size of the contact area (termed footprint). Transducers may have a small footprint to fit into small areas, such as between ribs, from which the beam spreads in a large arc (e.g. a sector transducer). Alternatively, they may be larger with a flat or slightly curved surface where contact can be maintained, such as a linear probe. Special transducers have been designed for use within body cavities, such as transoesophageal, endovaginal and endo-anal probes. These transducers offer the advantage of reduced distance between the transducer and area of interest, which allows higher frequencies to be used resulting in improved resolution. Very high frequency transducers have been used for intravascular and superficial ocular scanning. Appropriate choice of transducer is important in ensuring the optimal image is obtained.
The scope of emergency department ultrasound
Extended focused assessment by sonography for trauma (EFAST) [3–6]
Descriptions of the use of ultrasound by clinicians to evaluate trauma patients appeared in the European literature in the 1970s. Reports have subsequently appeared from countries around the world and the technique is now well established. Initially limited to the abdomen and pericardium (FAST), the examination is now routinely extended to include the chest (EFAST). With relatively brief training and experience, non-radiologists are able to diagnose haemoperitoneum, pericardial effusions, pleural effusions and pneumothorax with a high degree of sensitivity and specificity, although accuracy does improve with experience.
Clinical examination in abdominal trauma can be difficult and unreliable. Diagnostic peritoneal lavage (DPL), ultrasound (FAST) and computed tomography (CT) have been used to evaluate further this group of patients. In most cases, FAST has replaced diagnostic peritoneal lavage as it is non-invasive and does not interfere with subsequent interpretation of CT images. CT scanning is highly accurate for diagnosing both free fluid and solid organ injury and is slightly less accurate for hollow viscus and diaphragmatic injury.
Studies of ultrasound scanning in trauma have reported varying sensitivity. Much of this variation is due to differences in the gold standard used for comparison and definition of ‘true positive’. Haemoperitoneum (on further imaging, surgical or post-mortem examination), organ injury and clinical stability have all been used in different studies. It must be remembered that the primary role of a FAST scan is to detect free fluid in the peritoneal or pericardial spaces, for which it has high sensitivity and specificity. Solid organ or retroperitoneal haemorrhage may be detected but, even in expert hands, the accuracy is much lower (with as many as two-thirds of injuries being missed). FAST has been shown to be reliable and useful in both pregnant and paediatric patients.
Technique
FAST scanning evaluates four regions for the presence of free fluid: (1) pericardial; (2) perihepatic; (3) perisplenic; and (4) pelvic (Fig. 23.1.4). The chest is then scanned to examine the pleural spaces posterolaterally for fluid and anteriorly to exclude pneumothorax. The technique is rapid, generally being completed in under 5 minutes.
Free fluid appears as an echolucent area (i.e. black) which is generally linear or triangular in shape in the most dependent area of the peritoneal or pericardial space, although blood clots may be seen as echogenic (grey) collections (Figs 23.1.5–23.1.7). While fluid is most commonly seen in the perihepatic space, all spaces should be examined before the result can be considered negative. Small amounts of fluid (<500 mL) may not to be detected.
When scanning the chest, the presence of lung sliding or lung pulse is an indication that the visceral and parietal pleura are in contact, excluding a pneumothorax at that point. The presence of a moving transition point between areas of lung sliding and absent lung sliding (the ‘lung point’ sign) is diagnostic of pneumothorax.
Limitations and pitfalls
User dependent with learning curve.
Retroperitoneal haemorrhage may be missed.
Solid organ, hollow viscus or diaphragmatic injuries can occur without free fluid.
Small amounts of free fluid may not be detected.
Small amounts of pelvic fluid may be physiological in women.
Fluid-filled bowel can be misinterpreted as free fluid.
Pericardial fluid may decompress into the pleural cavity.
Loss of lung sliding may be due to causes other than pneumothorax.
Clinical implications and utility
The limitations of ultrasound in excluding all intra-abdominal injuries requiring laparotomy and the increasing use of conservative management of some injuries, even in the setting of intra-abdominal free fluid, has resulted in there being no universally accepted clinical algorithm based upon EFAST scan results. However, in this regard, EFAST scanning is no different to any other clinical, laboratory or imaging information of the trauma patient, the results of which are routinely used in combination to determine the management plan. Various algorithms incorporating EFAST scanning have been proposed, which generally incorporate haemodynamic stability and EFAST scan result, such as in Figure 23.1.8. Some algorithms incorporate a semiquantitative scoring system to estimate the amount of free fluid, with an increased volume of free fluid associated with greater need for therapeutic laparotomy. A positive abdominal EFAST scan is highly predictive of significant intra-abdominal injury and, based upon the clinical condition of the patient, generally indicates the need for CT or surgical exploration. A negative EFAST scan, stable haemodynamics and clinical observation have been shown to be highly accurate in excluding significant intra- abdominal injury. Some authors advocate serial EFAST examinations in stable patients, suggesting this can reduce the requirement for CT.
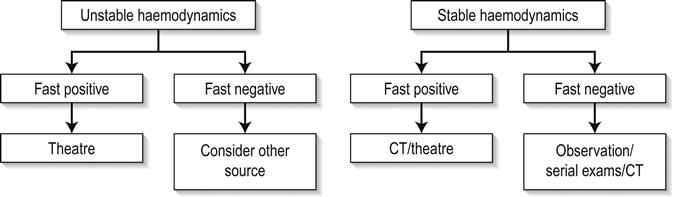
Similarly, for pneumothorax, the integration of EFAST findings with other clinical, laboratory and imaging findings will determine patient management. Conservative management of small pneumothoraces, even in the setting of positive pressure ventilation means that the ultrasound findings must be considered in the setting of the individual patient when management decisions are made.
In the Australasian setting, EFAST is generally accepted as fulfilling a complementary role to CT. Its portability and speed allow it to be used early in the evaluation of trauma patients (e.g. immediately after the primary survey) and this information is then incorporated with other clinical information to risk stratify the trauma patient to help to determine the requirement and timing for either laparotomy, thoracotomy or CT. Repeated examinations, particularly if the patient’s condition changes, can be valuable. Providing the limitations of the technique are not ignored, it can rapidly provide vital information to assist with patient management.
Abdominal aortic aneurysm [7,8]
Abdominal aortic aneurysms (AAA), defined as an aortic diameter>3.0 cm, are common, occurring in 1–9% of the population. Clinical assessment of the abdominal aorta is unreliable and may be especially difficult in the obese or unstable patient with abdominal pain. Clinical presentation of ruptured abdominal aortic aneurysm can be varied, with only 50% of patients demonstrating the classic presentation of hypotension, back pain and pulsatile mass. Other presentations may include abdominal, groin or flank pain, unexplained hypotension, syncope, haematuria or cardiac failure and AAA should be considered in any of these presentations.
Ultrasound is the primary mode of investigation of the abdominal aorta. Ultrasound performed by emergency clinicians has been shown to be rapid, highly sensitive and highly specific (>95%) in assessing aortic diameter. Ultrasound may occasionally detect rupture, but it is not reliable in excluding rupture. In addition to its utility in diagnosing AAA, ED ultrasound is very beneficial in rapidly excluding AAA in the wide variety of presentations listed above.
The risk of rupture of an AAA increases with the diameter. Although the risk of rupture if the aneurysm diameter is less than 4 cm is<0.5% per year and 1.5% per year for aneurysms 4.0–4.9 cm, rupture can still occur. Approximately 10% of ruptured aneurysms measure 5 cm or less.
Technique
The aorta should be identified anterior to the vertebral body and to the left of the inferior vena cava (IVC). It should be followed from the epigastric region to its bifurcation, just above the umbilicus, remembering that, in elderly patients, it may follow an ectactic course rather than following a strictly cranial–caudal course. It must be distinguished from both the superior mesenteric artery (SMA) (which runs anterior to the aorta) and the IVC (ensuring that the venous pulsation of the IVC is not mistaken for the arterial pulse of the aorta). Measurements should be taken both proximally and distally and, if an aneurysm is present, at the widest point. Measurements from both transverse and longitudinal planes should be taken. Measurements are taken from the outer wall to outer wall, including any mural thrombus (Fig. 23.1.9). If the renal arteries or SMA origin are identifiable then the relation to the aneurysm should be noted although, in the ED setting, this may not be possible. Any retroperitoneal haematoma or peritoneal free fluid should be noted.
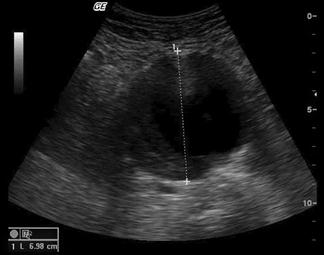
Limitations and pitfalls
Clinical implications and utility
In the patient with ruptured AAA who is haemodynamically unstable, ED ultrasound allows rapid and accurate diagnosis within the resuscitation area. Rapid diagnosis of these patients is essential to achieve successful treatment. In the stable patient, whose presentation may be atypical, ED ultrasound provides a rapid means of excluding the diagnosis (e.g. in the elderly patient who presents with ‘renal colic’). If an AAA is detected in these patients, then further imaging will often be required to determine if the AAA is an incidental finding or the cause of the patient’s symptoms. If the AAA is an incidental finding then formal follow up should be arranged.
Early pregnancy [9,10]
Ultrasound is the primary imaging modality for early pregnancy and its complications [11]. In the emergency department setting, it is most commonly used for the pregnant patient with pain or bleeding. In addition to transabdominal scanning (TAS), transvaginal scanning (TVS) can be performed with patient consent using a specifically designed probe which places the transducer close to the pelvic organs and utilizes higher frequencies to produce images of much greater detail than TAS. It does not require a full bladder and should not be a painful procedure. TAS still has an important role, as it allows a broader field of view that allows better assessment of large amounts of free intraperitoneal fluid and may diagnose other causes of pain. Emergency physician performed ultrasound for early pregnancy complications has been shown to be safe and reduce the time patients spend in emergency departments.
Technique
TAS is performed initially, preferably when the patient has a full bladder, as the pelvic organs will be better visualized. The uterus is identified and examined in both longitudinal and transverse planes (recognizing that the longitudinal axis of the uterus may not necessarily be in a strictly sagittal plane). The endometrial thickness is noted and any fluid collections or gestational sac noted. The adnexa are examined to identify the ovaries and any masses. The pelvis is scanned for free fluid. The upper abdomen can be examined to estimate the volume of free fluid if seen. The kidneys can also be examined to identify any alternate diagnoses.
TVS is performed after the procedure is explained and consent is obtained. A chaperone should be present if the sonographer is male. The patient is asked to empty their bladder and the pelvis is elevated slightly off the bed using a foam wedge or similar. The probe is covered with a sterile sheath (e.g. condom) with gel placed inside and outside the sheath. The probe is gently inserted into the vagina and advanced. The uterus and adnexa are then examined in both longitudinal and transverse planes as in TAS. After the scan is complete the probe must be cleaned and disinfected.
Limitations and pitfalls
Clinical implications and utility
The primary aim of ultrasound in evaluating early pregnancy complications in the emergency department is to locate the gestational sac. Additional information should then be sought for the presence of free fluid, adnexal masses, embryonic size and embryonic cardiac activity (viability). The earliest ultrasound evidence of pregnancy is a small anechoic fluid collection surrounded by an echogenic ring, which can be seen on TVS at approximately 4.5 weeks. A pseudogestational sac (due to fluid within the endometrial cavity), however, can have very similar appearances. Definite signs that the sac is a true gestational sac appear at 5.5 weeks when the yolk sac can be visualized, or later when the embryo can be identified. A heartbeat may be visualized from 6.0 to 6.5 weeks onward. TAS will show the same features but 1–2 weeks later.
Pregnancies that are not identified by ultrasound are termed ‘pregnancy of unknown location’ (PUL). Most will either fail (miscarry or resolve spontaneously) or progress to normal pregnancy. However, 9–43% will eventually be identified as ectopic pregnancies (lower rates are seen in centres with more expert scanning ability as they have higher rates of definitively diagnosing ectopic pregnancy). Quantitative human chorionic gonadotrophin (HCG) levels have been used to determine when a gestational sac should be identifiable by ultrasound, termed the ‘discriminatory zone’. For TVS, this is usually 1500 IU and for TAS 4500 IU (varying between institutions and depending on expertise and equipment). Even though a normal pregnancy may not be expected to be seen in patients with β-HCG levels below these levels, ultrasound should still be performed as it may still show diagnostic findings. In particular, ectopic pregnancies often have lower β-HCG levels than normal pregnancies of corresponding gestation and may be seen, as can the presence or absence of free fluid, which is valuable for risk stratification.
All patients with pregnancy of unknown location require close follow up with serial HCG and repeat ultrasound.
If an intrauterine pregnancy is confirmed, the risk of ectopic pregnancy is very low in spontaneously conceived pregnancies. Heterotopic pregnancy is where both an intrauterine and extrauterine pregnancy coexist and occurs in up to 1:7000 pregnancies in spontaneous conceived pregnancies, but over 1:100 pregnancies in the setting of fertility treatment. Failure to visualize an intrauterine pregnancy may be due to early dates in a normal pregnancy, failed intrauterine pregnancy (including complete miscarriage) or ectopic pregnancy. Other ultrasound findings in ectopic pregnancy include non-specific findings, such as pelvic blood and adnexal mass (Table 23.1.2) [12]. Visualization of a gestational sac (with yolk sac or embryo) outside the uterus is diagnostic, but seen only in 8–26% of ectopic pregnancies (Fig. 23.1.10).
Table 23.1.2
Ultrasound findings of ectopic pregnancy
Ultrasound finding | Accuracy (%) |
Absent IUP | 5 |
Any free fluid (no IUP) | 50 |
Mod–large free fluid (no IUP) | 60–85 |
Adnexal mass (no IUP) | 75 |
Adnexal mass+free fluid (no IUP) | 97 |
Ectopic pregnancy seen | 100 |
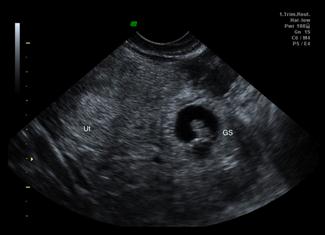
Unusual forms of ectopic pregnancy include interstitial, cervical and scar ectopics. In these cases, a gestational sac may be seen, but not within the true uterine cavity. It is recommended that pregnancies that appear low or eccentric should be reviewed by expert sonographers.
Distinguishing between miscarriage and ectopic pregnancy when no adnexal mass has been identified can be difficult on ultrasound. However, if the clinical symptoms have settled, no free fluid is identified on ultrasound and no adnexal masses have been identified, then it is safe to observe or discharge the patient for formal ultrasound review the following day and subsequent follow up with repeat ultrasound and quantitative HCG (see Chapter 19.2).
If an intrauterine pregnancy is confirmed, the gestational age can be estimated by measuring the size of the embryo. Most machines will automatically calculate gestational age based upon this measurement. Cardiac activity can usually be identified by TVS once the embryo is approximately 5 mm (9 mm by TAS). Absent cardiac activity when the embryo is 7 mm or above suggests embryonic demise. Absent yolk sac or embryo on TVS when the gestational sac is 25 mm suggests an empty sac miscarriage (also referred to as a blighted ovum) [13]. Other sonographic signs of poor prognosis for continued pregnancy exist, but they are generally beyond the scope of emergency ultrasound.
Right upper quadrant/gallbladder [14]
Upper abdominal pain due to biliary disease is a common presenting complaint to emergency departments and includes biliary colic, choledocholithiasis, cholecystitis and ascending cholangitis. Many of the patients suspected of having acute cholecystitis will have alternate diseases and clinical examination is neither sufficiently sensitive nor specific for these patients. Ultrasound is the primary imaging modality for these patients, where it is used to detect the presence of gallstones (see Fig. 23.1.1), other sonographic signs of cholecystitis and bile duct obstruction. It is superior to both scintigraphy and CT for these patients.
Ultrasound has a high sensitivity and specificity for the identification of stones when performed by either radiology or ED staff. Some stones may, however, be missed and false-positive results also occur. The diagnosis of cholecystitis relies upon associated findings including sonographic Murphy’s sign, gallbladder wall thickening, gallbladder distension and pericholecystic fluid (Fig. 23.1.11). Gallbladder wall thickening and pericholecystic fluid are both non-specific findings and may be seen in other hepatic or generalized diseases as well as in acalculous cholecystitis. Fasting can cause gallbladder distension. The common bile duct, if visualized, should be measured and examined for stones, although this is technically more difficult and may be beyond the scope of a focused gallbladder examination.
Technique
The gallbladder (GB) is usually identified by scanning under the costal margin in a longitudinal plane. Positioning the patient in the left lateral decubitus position and/or deep inspiration may assist. The gallbladder should be scanned throughout its length in both longitudinal and transverse planes. The sonographic Murphy’s sign is assessed by pressing with the ultrasound probe over the gallbladder. Wall thickness should be measured and is normally less than 3 mm. Gallstones will appear as bright, echogenic masses with posterior acoustic shadowing. Stones tend to be mobile unless impacted in the gallbladder neck or cystic duct. Stones impacted in these positions are technically more difficult to detect and may be missed if not painstakingly searched for. Sludge and polyps will also appear echogenic but will not shadow. Whenever possible, the common bile duct (CBD) should be visualized, measured and followed; when dilated, distal CBD obstruction should be considered.
Limitations and pitfalls
Clinical implications and utility
In a patient with abdominal pain, the finding of gallstones with a positive sonographic Murphy’s sign is strongly predictive of cholecystitis. The more sonographic signs of cholecystitis that are seen, the more likely the diagnosis. However, asymptomatic gallstones are common and may therefore represent an incidental finding, especially if the sonographic Murphy’s sign is absent. In elderly, diabetic or critically ill patients, 5–10% of cholecystitis can be acalculous. In those patients thought to have biliary colic or cholecystitis, a negative ultrasound should prompt a search for alternative diagnoses or consideration of further imaging, either formal ultrasound or, if an alternate diagnosis is believed likely, CT.
Renal ultrasound
The primary focus of renal ultrasound in the emergency setting is the detection of hydronephrosis in the presence of acute renal failure or renal colic [15]. As experience increases, users can often detect renal calculi, particularly when located at the vesicoureteric junction (VUJ). The presence of a ureteric jet excludes complete ureteric obstruction on that side.
Technique
The kidneys are paired retroperitoneal organs lying on either side of the spine between T12 and L4. They have a convex lateral border and a concave medial border and hilum. The normal adult kidney is 9–12 cm in length, 2.5–4 cm thick and 4–6 cm wide. The kidney itself is composed of two distinct areas, the renal parenchyma and the renal sinus.
The adult kidney is scanned using a curvilinear 3.5–5 MHz transducer and a renal preset that provides the best contrast resolution and grey map for imaging the kidneys. The patient may be supine, although the kidneys are usually best seen with the patient in a lateral decubitus position. A combination of subcostal and intercostal approaches is often necessary to fully evaluate the kidneys. The kidneys should be imaged in at least two planes, including the sagittal or coronal plane and the transverse plane (Figs 23.1.12 and 23.1.13). On ultrasound, the kidney can be identified by its elliptical shape with a thin echogenic capsule. Normal renal parenchyma has slightly decreased or equal echogenicity relative to the hepatic or splenic parenchyma, although this is age dependent with it being comparatively hyperechoic in the elderly. The central renal sinus is particularly echobright due to the fat and fibrous tissue content. The renal pelvis and infundibulum are usually collapsed and not seen except in the setting of hydronephrosis when they become filled with urine, appearing anechoic. The bladder should be full and examined in both the sagittal and transverse planes to complete the study. It should be noted that an excessively full bladder may cause mild dilatation of the pelvicalyceal system, however, this will return to normal following micturition.
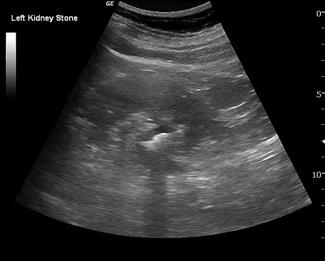
Ureteric jets occur when either ureter contracts propelling urine into the bladder. This occurs every 10–20 seconds in the euvolaemic patient with normal renal function and excludes complete obstruction on that side. The presence of a jet, however, does not exclude the possibility of a non-obstructive renal or ureteric calculus.
Hydronephrosis is the dilatation of the renal pelvis and calyces and may be secondary to an anatomical obstruction or may be functional in nature (such as with ureteric reflux). Obstructive hydronephrosis may be intrinsic or extrinsic. Depending on the level of obstruction, it may be unilateral or bilateral with or without associated hydroureter. When hydronephrosis is identified, the cause for the obstruction should be sought. Common intrinsic obstructive causes seen in the emergency department include obstructive or partially obstructive renal or ureteric calculi. Bladder outlet obstruction due to prostatic hypertrophy is another common cause of hydronephrosis. Extrinsic or invasive masses in the pelvis obstructing either the ureters or bladder outflow should also be considered.
Hydronephrosis may be described as mild, moderate or severe depending on the extent of dilatation of the renal collecting system:
Limitations and pitfalls
Clinical implications and utility
Ultrasound is less sensitive than plain films or CT in detecting renal calculi. Small stones may often be obscured by the echogenic renal sinus and be hard to detect if they have a weak posterior acoustic shadow. Having said this, stones in the kidney that are greater than 5 mm in size have been shown to be detected in experienced hands with 100% sensitivity sonographically [16]. Renal stones appear as bright, echogenic foci with distal acoustic shadowing and sometimes twinkle artefact when interrogated with colour Doppler. Ureteric calculi are difficult to visualize as they are often obscured by bowel gas in their retroperitoneal position. A normal appearing kidney and the failure to visualize a calculus therefore does not exclude a ureteric calculus that is non-obstructing or where hydronephrosis has not yet developed.
Deep vein thrombosis (DVT)
The primary focus of emergency department ultrasound in the assessment of DVT is in the diagnosis or exclusion of a proximal lower limb DVT.
The clinical assessment of DVT is unreliable and inaccurate [17,18]. Positive findings on sonographic examination of only 11% have been reported for patients referred for suspected acute DVT on the basis of clinical features [19].
Technique
Ultrasound is the imaging modality of choice for assessing for DVT. The technique relies primarily on B-mode imaging with intermittent venous compression, with the main diagnostic criteria used to exclude a DVT being complete collapse of the vein with apposition of the anterior and posterior walls of the vessel.
A broadband linear array transducer with a centre frequency of about 5 MHz is used to examine the femoral, popliteal and sometimes the calf veins. In larger patients, the curved linear array transducer with a centre frequency of 3.5 MHz (as used for abdominal studies) may be substituted. The curved linear array transducer is also used to examine the iliac veins. The machine should be configured to use the lower limb venous preset and the use of harmonic imaging may improve the contrast resolution between the vessel and surrounding tissue. Transducer compression of the interrogated vessel should be in the transverse imaging plane. Starting at the level of the groin, with the patient in a supine position, the common femoral vein is identified lying medial to the common femoral artery and the vein is compressed to demonstrate collapsibility extending distally in a stepwise fashion with the vein compressed every 2–3 cm (Fig. 23.1.14). Where thrombus is present in the vein, pressure with the transducer will not result in its collapse. The popliteal vein is best examined with the patient in a lateral or prone position with the knee slightly flexed. Colour and spectral Doppler may be used to supplement the findings of intermittent compression. Emergency physicians who had undergone standardized training to identify clot in the femoral or popliteal veins have shown an accuracy comparable to formal vascular studies [20].
Limitations and pitfalls
Clinical implications and utility
The accuracy of compression ultrasonography is highest in symptomatic patients, with studies comparing venography with compression ultrasound demonstrating an average sensitivity of 95% and specificity of 98% [21]. For proximal lower limb DVT, this technique has demonstrated sensitivity of up to 100% [22]. The use of colour and spectral Doppler to assess for vessel filling defects and flow patterns has not been shown to increase significantly the sensitivity for proximal DVT detection in the lower limb [22–25]. It has also been suggested that an abbreviated technique, using only two compression points (the saphenofemoral junction and the lower popliteal vein) has adequate sensitivity, provided repeat examination is performed in 5–7 days [24,26,27]. The accuracy of ultrasound in detecting isolated calf DVT, especially when applied to bedside emergency ultrasound, is low with success rates as low as 40% reported [28].
Thus, the aim of focused emergency department ultrasound in the assessment of DVT is generally to confirm or exclude the presence of clot in the proximal deep veins of the lower limb. A negative compression ultrasound study of the proximal lower limb significantly reduces the likelihood of DVT and discharge from the emergency department without anticoagulation, with outpatient follow up for a definitive study can be considered [21,29–31].
Emergency echocardiography
Focused use of echocardiography in the emergency department represents one of the most valuable uses of ultrasound in emergency medicine. Applications include its use in cardiac arrest, undifferentiated hypotension, suspected pericardial effusion and tamponade, chest pain, pulmonary embolus and ultrasound guided procedures. The use of cardiac ultrasound in emergency medicine is likely to increase significantly as more emergency physicians learn the technique and look to apply it increasingly in the clinical environment.
Echocardiography provides direct structural and functional information on cardiac structures only inferred by clinical examination, which has been shown to have limited accuracy, and with greater sensitivity and specificity than indirect tests, such as ECG and chest radiography [32–34].
Technique
Modern general ultrasound machines can provide good quality transthoracic echocardiography capability. A broadband phased array transducer with a centre frequency of 2 MHz and a small footprint to improve access between the ribs should be used. Ideally, the patient should be positioned on their left side.
The full, standard echocardiographic examination includes parasternal long and short axis views obtained at the left sternal edge in the 2nd to 4th rib spaces, the apical 4-, 5-, 3- and 2- chamber views that are obtained at the cardiac apex and the subcostal views obtained from a subxiphoid position. The standard examination would involve 2-D assessment of cardiac structure and function using B-mode, supplemented by the use of colour and spectral Doppler to assess valvular function and measure chamber pressures using the windows described above.
Where an abbreviated, emergency bedside echocardiographic examination is being used to screen for the presence of tamponade, right ventricular dysfunction, left ventricular dysfunction and hypovolaemia alone, simply using B-mode and attaining the parasternal short and long axis views, the apical 4-chamber view and the subcostal view is generally enough.
In cardiac arrest, the subcostal view is used with the patient in the supine position. All preparations are made while CPR continues. During the 10-second pulse check, a loop is recorded and reviewed once CPR recommences. It is imperative that the time without CPR is minimized and echocardiography should not interfere with this.
Emergency physicians have been shown to assess accurately left ventricular function in the hypotensive patient [35].
Limitations and pitfalls
Clinical indications and utility
In cardiac arrest, the aim of echocardiography is to assess left ventricular activity as well as to exclude the presence of potentially reversible causes, particularly tamponade. In the setting of cardiac arrest, cardiac standstill on initial presenting echocardiographic assessment has important prognostic implications, irrespective of presenting electrical rhythm. Blaivas et al. demonstrated that no patients out of 136 presenting with cardiac standstill on initial echocardiographic assessment survived to leave the ED irrespective of presenting rhythm, findings supported by Salen et al. in a study of 102 patients presenting in cardiac arrest [37,38]. Having said this, several case reports have recently been written describing patients who developed return of spontaneous circulation (ROSC) after having echo-documented cardiac standstill. As is always the case, the user must integrate ultrasound findings into the clinical picture to make a final clinical decision. Cardiac standstill in the newly collapsed patient, the hypothermic patient, the young patient or the toxicological overdose should not be used as the sole criterion to cease resuscitative efforts.
Echocardiography is very useful in determining the cause of undifferentiated hypotension and shock. The primary aim of focused emergency echocardiography in this setting is to assess for tamponade, for right ventricular (RV) dysfunction (that in the correct clinical setting would infer massive pulmonary embolism), for left ventricular systolic dysfunction and for hypovolaemia.
A pericardial effusion is seen as an anechoic collection of fluid between the visceral and parietal pericardium (Fig. 23.1.15), although an inflammatory pericardial effusion or haemopericardium may exhibit internal echoes. In differentiating between a pericardial effusion and a pleural effusion, a pericardial effusion tapers towards and anterior to the descending aorta and may extend a short distance between the aorta and left atrium, conversely, a pleural effusion will accumulate and extend behind the descending aorta. When a pericardial effusion is identified, its location and size should be documented and any evidence of tamponade looked for. The size of an effusion can be described as small, moderate or large. A small effusion is≤1 cm in thickness and may be localized. A moderately sized effusion is between 1 and 2 cm and is generally circumferential unless loculated. A large effusion is described as being>2 cm. In a group of 515 patients at high risk for pericardial effusions (103 of whom had pericardial effusions), emergency physicians were able to detect an effusion with an overall sensitivity of 96%, specificity of 98% and accuracy of 97% [36].
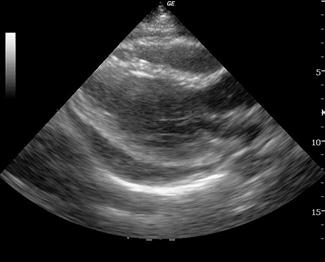
The risk of tamponade is more a function of the rate of accumulation than total volume of pericardial effusion. There are a number of different echocardiographic features used to define tamponade; however, its precise echocardiographic diagnosis remains complex and controversial. The most frequently used echocardiographic finding to support a diagnosis of tamponade is collapse of the right heart chambers during mid-to-late diastole and, specifically, right ventricular diastolic collapse. In the emergency department setting, pericardial tamponade should remain a clinical diagnosis. In a patient with signs and symptoms consistent with tamponade, the focus of emergency echocardiography is the identification of pericardial effusion. Its presence is then interpreted and acted upon in the clinical context.
Transthoracic echocardiography lacks sensitivity for diagnosing pulmonary embolism (PE). Echocardiography missed 16 of 39 patients presenting to an ED with PE, diagnosed by other modalities in a prospective observational study [39]. However, there are echocardiographic features associated with PE that, when identified and put into clinical context, can be highly suggestive or diagnostic. These include right ventricular dysfunction or dilatation, paradoxical septal motion, acute tricuspid regurgitation and the presence of clot in the right heart. While echocardiography may be a poor tool for diagnosing PE, it may be useful in assessing RV dysfunction caused by PE and may have a role in risk stratifying patients and influencing the decision to use thrombolytic therapy. RV dysfunction is associated with a significantly higher mortality [40] and thrombolysis may be considered in this setting, although the specific criteria for the use of thrombolytic therapy to treat PE remains controversial.
When the cause of shock is primarily due to pump failure, then echocardiography demonstrates a poorly functioning, often dilated left ventricle (LV) and/or atrium. Differentiating severe global left ventricular dysfunction (as occurs is cardiogenic shock) from normal LV function or the hyperdynamic LV of hypovolaemia is readily done. More precise calculations of ejection fraction and cardiac output or regional wall motion abnormality are generally beyond the scope of the emergency physician.
In shock due to hypovolaemia, echocardiography demonstrates a small left ventricular end-systolic volume with hyperdynamic left ventricular motion. The inferior vena cava also tends to be small or collapsed and demonstrates increased respiratory variation. In the spontaneously ventilating patient, inspiratory collapse of over 50%, particularly when the IVC is small, infers a high likelihood of fluid responsiveness.
The use of echocardiography for the acute assessment of chest pain is extremely useful but requires significant expertise and is generally beyond the scope of the ED user. Transthoracic echo can detect the regional wall motion abnormalities associated with cardiac ischaemia and, in expert hands, the sensitivity and specificity are relatively high. Echo too may detect the RV dilatation and dysfunction associated with PE, the pericardial effusion that may be associated with pericarditis, proximal aortic dilatation and even an intimal flap or aortic regurgitation from dissection but, for these conditions, has much lower sensitivity. Ultrasound can also detect numerous chest wall and pulmonary pathologies presenting with chest pain that may mimic cardiac pain and thoracic ultrasound is described in the next section.
Lung ultrasound
Lung ultrasound is being increasingly used by critical care clinicians and respiratory physicians to assess the patient with undifferentiated shortness of breath. It is also used to answer a diverse range of specific clinical questions, such as is there a pleural effusion, is there a pneumothorax or is there pulmonary oedema? Finally, it is used to guide pleural procedures, such as effusion drainage or biopsy.
Suggested algorithmic approaches, such as Lichtenstein’s ‘BLUE protocol’ for the patient with acute respiratory failure, claim a diagnostic accuracy of 90.5% [41]. This protocol assesses for cardiogenic pulmonary oedema, pneumonia, decompensated chronic obstructive pulmonary disease (COPD), asthma, pulmonary embolism and pneumothorax.
It should be recalled that neither bone nor air allows the passage of ultrasound. Because of this, one cannot assess directly behind a rib where an acoustic shadow occurs, nor deep to an air interface, such as the normal pleural surface. This means that thoracic ultrasound can focus on the bony thoracic cage where fractures of ribs and the sternum can be readily detected or on the pleural space where effusions, pleural masses or free air (as in pneumothorax) can be detected and, finally on the lung itself. In aerated lung, assessment can only be made of the visceral pleural surface (and a tiny rim of lung tissue directly adjacent to this). If the lung is not aerated, as occurs with solid tumours, consolidation, collapse or infarction, the solid area of lung deeper to the surface can be explored with ultrasound.
Sonographic assessment of aerated lung relies on two things. First, movement of the lung, with the two pleural surfaces sliding against one another during ventilation and, secondly, on artefact created by reverberation of ultrasound. This reverberation occurs at the pleural surface and again within minute collections of interstitial fluid or fibrosis. Normal ventilating lung is therefore characterized by ‘lung sliding’ where the very slightly irregular visceral pleural surface can be seen moving to-and-fro past the parietal pleura with inspiration and expiration. Even in normal lung, tiny foci of interstitial or alveolar fluid or fibrosis create short path reverberation artefacts, which appear as bright vertical lines deep to the pleural surface. When these are short they are called comet tails and when they are long they have been termed lung rockets or ‘B-lines’. When there is an increasing amount of interstitial or alveolar fluid as seen in pulmonary oedema, pneumonitis, acute respiratory distress syndrome (ARDS), lymphangitis carcinomatosis and pulmonary fibrosis the number and prominence of these vertical ‘B-lines’ increases dramatically. More subtle sonographic changes in the pleural surface may allow differentiation between these subgroups, however, often it is clinical correlation that makes the picture clearer.
With pneumothorax lung sliding is lost, as are the vertical comet tail and B-line artefacts. The contact or lung point can sometimes be found where the two pleural surfaces meet and allow some degree of assessment of pneumothorax size. In addition, with pneumothorax, the free air beneath the parietal pleural surface creates a smooth mirror-like effect. Long path reverberation artefacts, known as ‘A-lines’, occur where horizontal repetitions or reflections of the pleural surface are seen below the actual pleural surface. Lung sliding can also be absent in COPD, bullae or conditions where the lung surface is ‘sticky’ from inflammation of any cause.
Technique
The pleural surface is interrogated using either the curvilinear abdominal or the high frequency linear transducer. Several different methods for assessment have been described and depend on clinical suspicion, which must be used to guide and then interpret the scan. Integration of lung ultrasound and emergency echocardiography is the best approach to assessing the patient with undifferentiated acute dyspnoea.
In the absence of pleural adhesions or loculations, pneumothorax collects in the most apical portion of the thoracic cage and should be examined for there. If the patient is a supine trauma patient, assessment anteriorly along the midclavicular line from clavicle to diaphragm, with the probe in longitudinal is effective.
If considering pleural effusion or haemothorax, examining the bases and costophrenic angles from the front, side and back, usually with the patient sitting up, is best. To assess the lung parenchyma, maximizing the view of the pleura, lining the probe up in the line of the intercostal spaces and so avoiding ribs, is ideal.
For a non-trauma patient who presents in acute respiratory distress, sitting up, the author tends to examine the chest methodically:
Limitations and pitfalls
Clinical implications and utility
ED ultrasound allows the rapid assessment of patients in acute respiratory distress at the bedside. The integration of ultrasound findings with the findings of the clinical assessment and appropriate clinical judgement can vastly expedite and improve management of these patients.
In addition to the diagnostic utility of thoracic and lung ultrasound, having access to ultrasound for procedural guidance is extremely useful. Ultrasound can be used to assess an area of opacity seen on chest X-ray, to determine whether it is solid or liquid. If a pleural effusion is confirmed, it can be further characterized as being simple or loculated and as to whether there is debris floating within it. Aspiration or tube placement can then be done after calculating the thickness of the chest wall, the depth of the effusion and the best direction from which to approach. The procedure can be done in real-time or after the patient is positioned, the skin is marked and trajectory planned.
While the benefits of lung ultrasound are clear, there is a diverse range of pathology that one can encounter and often the differences in sonographic appearance between pathologies are subtle. Appropriate education and experience are essential as is the correlation of clinical assessment with the ultrasound assessment.
Ultrasound-guided vascular access
Vascular access, both venous and arterial and central and peripheral is commonly performed in the emergency department. Widespread availability of machines and increasing familiarity and expertise among the emergency medicine community in using ultrasound to guide all forms of vascular access has had major positive implications to patients in recent years.
Traditionally, central venous access has been secured using the ‘landmark technique’, where surface anatomical features are used to predict the location of the internal jugular, subclavian and femoral veins. However, access using this technique has been associated with a 20% failure rate and a 10% complication rate, including inadvertent arterial puncture, excessive bleeding, vessel laceration, pneumothorax and haemothorax [42,43]. Improved success rates and decreased complication rates have been described using ultrasound-guided central venous access, including reduction in needle puncture time, increased overall success, reduction in carotid puncture, reduction in pneumothorax and a reduction in catheter-related infection [44,45]. National guidelines from the UK [46] and the USA [47] support the use of ultrasound guidance for central venous catheter (CVC) placement.
Ultrasound guidance is also useful in aiding peripheral vascular access. The basilic, brachial and cephalic veins are frequently not visible clinically, but are readily cannulated using ultrasound guidance. Basilic vein cannulation has been shown to be very successful in the emergency department setting in patients in whom other peripheral access was difficult [48].
Technique
A medium to high frequency broad bandwidth linear array transducer is used with a centre frequency of 7.5–10 MHz. Specific presets to optimize the needle’s visibility have been developed, but a musculoskeletal preset is usually adequate. The procedure is most easily performed with the ultrasound screen, the patient and operator all in line, with orientation checked and optimized. A sterile transducer cover and ultrasound gel are essential. A longer cannula is also particularly useful as the vessels being targeted are generally deep and increased length is required to reach them adequately.
Two techniques have been described, the static and dynamic techniques. The static technique may be used for very superficial or very large vessels, but is generally considered inferior to the dynamic technique. The static technique is used to locate the vessel, measure its dimensions, confirm relationships of surrounding structures and determine depth below the skin. The vessel is then centred on the screen and the skin marked at the centre of the transducer, corresponding to the vessel’s subcutaneous position. This mark is then used for the puncture site without ultrasound visualization of the needle as it enters the vessel.
The dynamic technique uses real-time ultrasound guidance visualizing the needle tip as it enters the vessel. Higher success rates have been demonstrated with the dynamic technique than with the static technique [49].
Real-time ultrasound guided cannulation using both in plane and out of plane transducer orientation relative to the vessel have been described. The out of plane orientation is easier to obtain and provides information related to adjacent structures, however, the needle tip is less clearly seen. The in plane orientation is more difficult to achieve but provides information related to vessel orientation and slope and provides visualization of the needle in its entirety as it passes through the tissues and enters the vessel.
Clinical implications and utility
In many centres, emergency staff (both medical and nursing) are gaining familiarity and expertise with ultrasound-guided vascular access of all sorts. The increased use of ultrasound to place peripheral cannulae and PICC lines has meant less trauma for our patients and, in many cases, less need for central line placement.
Miscellaneous applications
Scrotal ultrasound
Patients may present to the emergency department with scrotal or testicular pain, a scrotal mass or following scrotal trauma. Acute scrotal pain in the absence of trauma may be due to testicular torsion or epididymo-orchitis. Scrotal swelling may be due to hydrocoele, hernia or testicular mass. Scrotal trauma may be associated with testicular rupture, haematoma and testicular ischaemia. Ultrasound is the imaging modality of choice for assessing for testicular pathology and injury.
The scrotum is examined using a high- resolution linear array transducer with the patient in a supine position with the scrotum supported by a towel between the patient’s legs.
In testicular torsion, the testis rotates on its axis leading to twisting of the spermatic cord with compromise of both venous drainage and arterial supply. To diagnose torsion, it is important to demonstrate normal flow within the normal testis and absent flow in the affected side [50]. However, it should be noted that flow may be very difficult to identify in normal paediatric testes and with intermittent torsion– detorsion, blood flow may appear normal or even increased in the affected testis. Partial torsion can occur with normal colour flow on ultrasound.
Epididymo-orchitis is the most common cause of scrotal pain in postpubertal men. Sonographically, the epididymis is characteristically thickened. Increased blood flow is demonstrated with colour Doppler in the epididymis or testis, or both. A reactive hydrocoele is common.
Ocular ultrasound
Ocular ultrasound has been used by ophthalmologists as an adjunct to examination for over 40 years. In the emergency setting, it is most useful to examine the eye in those patients where swelling prevents direct visualization of the eye and for disorders for which direct ophthalmoscopy has poor accuracy (particularly when performed by emergency doctors). Using a generous amount of gel, a high-frequency linear probe is gently applied to the closed eyelid and the globe examined in two planes from side to side. The posterior chamber should appear round and echo free. The optic nerve can be identified posteriorly. A retinal detachment appears as a relatively thick and echogenic membrane that may show colour flow on Doppler. In rhegmatogenous retinal detachments, the memberane undulates with eye movement (older detachments tend to move less with increasing fibrosis). Retinal detachments do not cross the optic nerve. Smaller areas of a thick elevated membrane that do not undulate may be seen with other types of detachments or other pathologies, but all of these will generally be beyond the scope of the emergency physician and require an ophthalmologist to distinguish. A vitreous detachment appears as a much thinner, avascular membrane that may only be seen when perpendicular to the beam, which also moves with globe movement. Vitreous haemorrhages appear as either dots or larger echogenic regions which move more quickly than retinal detachments. Studies have shown good accuracy when employed by emergency physicians to diagnose retinal detachment [51].
Ultrasound has also been used to diagnose globe rupture, foreign bodies and lens dislocation. Some groups have described its use in the diagnosis of intracranial hypertension, by looking at the optic nerve sheath diameter. However, while individual groups have found good accuracy, differences in technique and wide variation in the quoted normal ranges have limited the general applicability of this technique.
Appendicitis
Misdiagnosis of appendicitis on clinical assessment is associated with a negative appendectomy rate of 15%, with rates as high as 40–50% reported in some series [52]. Delays in intervention can result in appendiceal perforation with associated increased morbidity and mortality [53]. The aim in assessing a patient with clinically suspected appendicitis is to identify adequately the appendix to confirm or refute the diagnosis, identify complications, such as perforation, or to identify other causes of the patient’s presentation. The appendix is identified as a blind-ending, tubular, aperistaltic structure arising from the posteromedial caecum 1–2 cm distal to the ileocaecal junction. The patient should be examined in a supine position using a high-frequency linear array transducer to optimize image resolution. The normal appendix is compressible with a wall thickness equal to or less than 3 mm [54]. Increased wall thickness (outerwall to outerwall) of greater than 6 mm with loss of compressibility (Fig. 23.1.16), loss of definition of the mucosa, submucosa and muscularis propria and the visualization of an appendicolith support a diagnosis of appendicitis. Additionally, the detection of peri-appendiceal inflammatory changes in the presence of an abnormal appendix increases the likelihood of appendicitis [55]. Failure to identify the appendix is common and does not exclude appendicitis.
Musculoskeletal applications
There are numerous musculoskeletal applications for diagnostic ultrasound in emergency medicine. These include foreign body identification, evaluation of suspected tendon tears (Fig. 23.1.17), muscle tears and haematomas, joint effusions (Fig. 23.1.18) and fractures. Most musculoskeletal imaging is done using a broadband, high-resolution, linear array transducer with centre frequency of about 10 MHz.
Ultrasound-guided procedures
Ultrasound is a useful modality for identifying fluid collections and guiding diagnostic or therapeutic aspiration, including thoracocentesis, paracentesis and arthrocentesis. It is also increasingly used by both anaesthetists and emergency physicians to guide nerve blocks.
Training and credentialling [1]
In Australasia, both the Australasian College for Emergency Medicine (ACEM) and the Australasian Society for Ultrasound in Medicine (ASUM) make recommendations and provide credentialling pathways in emergency ultrasound. ASUM provides the nationally recognized qualifications the Diploma in Diagnostic Ultrasound (DDU) and the Certificate in Clinician Performed Ultrasound (CCPU).
In 1999, ACEM proposed a policy supporting the use of ultrasound by emergency physicians for at least the detection of traumatic haemoperitoneum, abdominal aortic aneurysm, pericardial fluid, ectopic pregnancy, renal and biliary disease. The subsequently published policy document supported a credentialling process for training in FAST and AAA studies and included recommendations on training requirements. These requirements mandated a minimum of 25 FAST exams with at least five positive scans for intraperitoneal, pleural or pericardial fluid and 15 AAA exams of which five should demonstrate an aneurysm for credentialling purposes. All of these training exams should be confirmed by another study or direct supervision of a suitably qualified physician or sonographer. The requirements also included attendance at an ultrasound workshop that would cover the basic information for an emergency physician to perform and interpret FAST and AAA studies. Guidelines for the Minimum Criteria for Ultrasound Workshop were published by ACEM in 2000. These policies were reviewed in 2011, and requirements for credentialling in Basic Echo in Life Support were added.
ASUM is the recognized national training, qualifying and credentialling body in medical ultrasound. ASUM offers two qualifications to medical practitioners performing ultrasound: the Certificate in Clinician Performed Ultrasound (CCPU) and the Diploma in Diagnostic Ultrasound (DDU). Both of these qualifications are endorsed by ACEM for the purposes of training and credentialling in emergency ultrasound and describe a scope of practice that extends beyond those described by the College. Further details can be obtained from the ASUM website.
In 2005, the Royal College of Radiologists in the UK, in consultation with the clinical colleges, published a document entitled ‘Ultrasound Training Recommendations for Medical & Surgical Specialties’. This document defines three levels of competency with suggested training and practice requirements for each level and has been endorsed by the College of Emergency Medicine (UK).
The 2001 American College of Emergency Physicians (ACEP) Policy Statement, Emergency Ultrasound Guidelines, reviewed the previous criteria for achievement of competency to perform focused clinical ultrasound. These recommendations included a 16-hour introductory course and a minimum of 25 ultrasound examinations for each defined primary modality.
Although the minimum training requirements for emergency physicians to become proficient in focused emergency ultrasound remains unclear, the recommendations from ACEP, ACEM, ASUM (for the CCPU) and the training recommendations of the Royal College of Radiologists (UK) for clinical ultrasound are similar and have been promulgated in a consensus document by the International Federation for Emergency Medicine.
The increasing technological sophistication, portability and affordability of ultrasound machines has led to an increasing demand for ultrasound as a diagnostic tool to be devolved to the clinician managing the patient. This is no more so than in emergency medicine where ultrasound has the potential of establishing a broad range of applications and indications that extend beyond EFAST and AAA detection as is described by the training curricula and guidelines above. The challenge now lies in developing adequate training and supervision networks to allow these skills to be learnt and maintained.
23.2 CT scanning in emergency medicine
Steven J Dunjey and Swithin Song
Introduction
Computed tomography (CT) was developed in 1971 by Godfrey Hounsfield and Allan Cormack and was rapidly adopted into medical practice. By the early 1980s, CT scanning was in general clinical use in the USA and, within a generation, most large emergency departments have acquired their own dedicated machines. Emergency medicine has enthusiastically embraced the utilization of this modality, which provides rapid answers to many of our diagnostic questions. It has revolutionized the approach to patients with traumatic injury, neurological emergencies, including head injuries, subarachnoid hemorrhage and strokes, abdominal pain and, more recently, chest pain. It is assuming the pre-eminent position in the investigation of patients with possible pulmonary embolism. It is cost effective, fast, less sensitive to patient movement than magnetic resonance imaging and (unless contrast is used) non-invasive. It is, however, a modality that presents some real risks to patients and clinicians need to be prudent in their use of CT. It is also an area of considerable development and, as CT scanners become faster and more accurate, more uses are being found for this modality.
Development science
CT scan machines essentially consist of a gantry around a patient, with an X-ray source on one side of the gantry and detectors on the opposite side moving in synchrony with the source. Early scanners imaged one slice at a time (‘step and shoot’), with the table stationary while a static image was acquired. This produced a series of parallel slice images (tomographic images) of a region of the body. The beam produced by the source can be adjusted, producing widths from 1 to 20 mm. Traditionally, images were produced which displayed the volume of data as axial slices (transverse slices, perpendicular to the long axis of the body), but current scanners are able to display the collected information as coronal or sagittal slices which improves diagnostic yield (Figs 23.2.1–23.2.3).
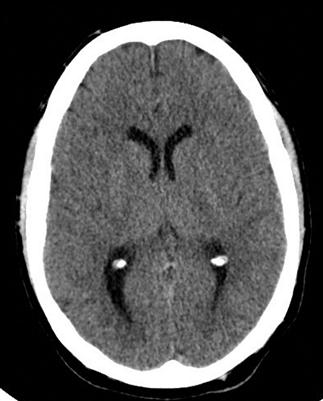
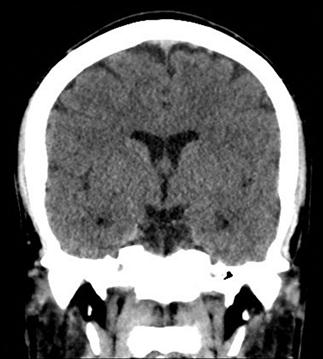
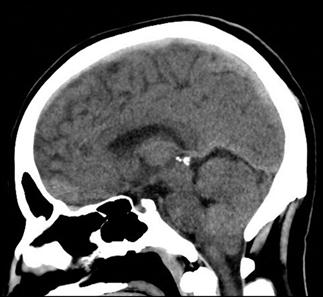
Helical (spiral) CT scanners move the patient rapidly and continuously through a circular gantry opening which is equipped with a source and multiple detectors, which are continuously rotating and provide continued volumetric acquisition. The source describes a helical trajectory relative to the patient.
There have been considerable advances in computer technology and, in combination with the development of multislice helical CT, there has been significant improvement in the resolution and diagnostic utility of CT scanners. Helical scanners are also capable of producing a 3-dimensional image of an internal organ by digital post-processing.
Windows
The objects displayed in a scan can be differentiated from adjacent organs by their differential attenuation of the X-ray beam based on their individual density. The density of the tissues in the scan produced is measured in Hounsfield Units (HU). Water has a density of 0 and tissues that are denser than water have values greater than 0, while less dense tissues have negative values. The accepted convention is for high-density structures to be displayed in white shades and low-density structures to be displayed in darker shades. The denser a structure, the whiter the shade displayed. The scale extends up to about+4000 for very dense metals, but cancellous bone is about+700 and dense bone about+3000. Blood is in the range of+35 to+45 and muscle about+40. At the other extreme of the scale, air is−1000, lung−700 and fat−84 (Figs 23.2.4 and 23.2.5).
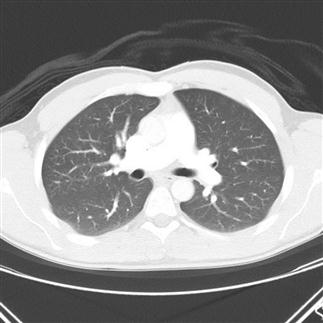
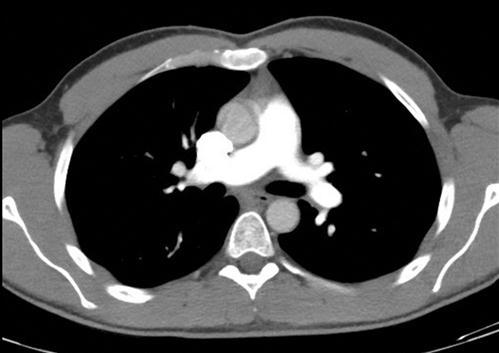
Humans can only perceive a limited number of grey shades and so to highlight the tissues of interest, the full range of density values is not displayed. Instead, the display shows a narrow portion of the full range to allow clear differentiation of one tissue from another and pathological tissue from healthy tissue.
For example, bone windows are a preset that will shift the grey scale displayed to center on the range of densities which are typical of bone and allow detection of subtle abnormalities, such as small fractures. As a consequence of focusing the display on such high-density structures, there is a marked decline in the ability to assess soft tissues on bone windows.
Display convention
The accepted convention for displaying images is for the right side of the patient to be on the left side of the image, which is best appreciated by imagining yourself standing at the feet of the CT gantry, with the patient supine.
Artefacts
There are still a number of imaging artefacts that affect the quality of the images generated and hence the diagnostic value of the scan [1]. These artefacts arise from a number of sources, classified as physics-based artefacts, patient based, scanner based and multisection based [3]. Most emergency physicians are familiar with patient-based issues, such as patient movement (Fig. 23.2.6) or metallic objects in or around the patient (Figs 23.2.7 and 23.2.8).
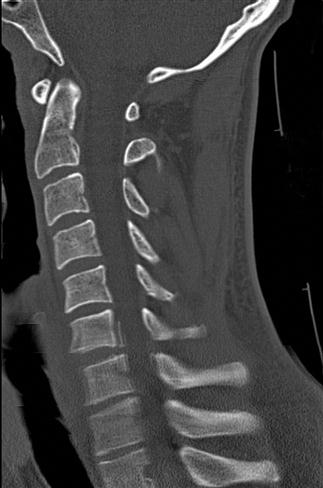
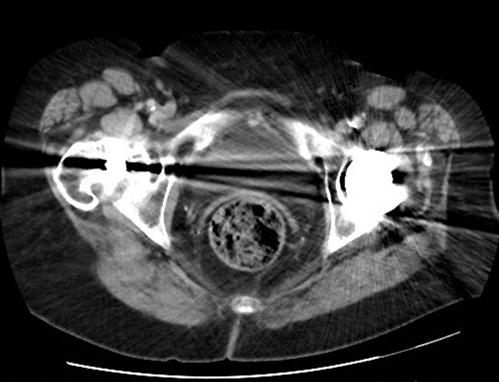
Physics-based problems include beam hardening (resulting from the absorption of low-energy photons after passage through an object, leaving only high-energy photons and a higher energy beam) which can produce the streaks and dark bands that are sometimes seen. Undersampling is another physics-based problem, in which the distance between CT samples is large enough to create misregistration of information about small objects or sharp edges. Partial volume averaging is another common problem, in which the average of densities in a single CT is averaged rather than displaying separate individual densities. That this occurs should not be a surprise, because every CT slice displayed is a 2D representation of a finite 3D thickness of tissue [4]. Ring artefacts, a scanner-based problem, occur due to miscalibration or failure of one or more detector elements in a CT scanner (Fig. 23.2.9).
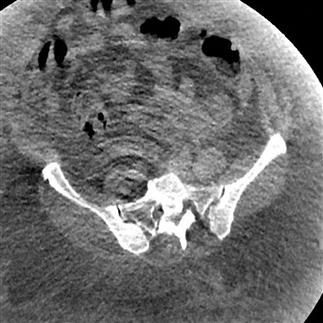
Current uses and indications
While the overall usage of CT scanning in medicine is dramatically increasing, evidence suggests a higher rate of increase in emergency medicine than elsewhere. A 2011 study showed that, in the USA between 1995 and 2007, the number of CT studies performed increased from 2.7 million to 16.2 million – nearly a sixfold increase [5].
By the end of the study period, the presenting complaints topping the list of those undergoing CT were abdominal pain, headache and chest pain. The percentage of patient visits associated with CT for all complaints increased most substantially among those who underwent CT for flank, abdominal or chest pain. Other high-use areas include shortness of breath, trauma and headache and use is more marked in the elderly population [6].
Indications for emergency department CT scans increase every year, especially as the manifest deficiencies of plain X-rays become clearer. Examples include CT supplanting the traditional role of plain films in assessing head injury, cervical spine injury and renal colic. This era, when long-held beliefs about the accuracy of clinical examination are being challenged, acts as a force encouraging increasing reliance on good diagnostic tests. In the last decade, there has been a dramatic increase in the number of CT scanners such that even moderate- sized country hospitals now have scanners and most large urban emergency departments have their own dedicated scanners. During the same period, scanners have become faster with improved diagnostic yield. All of these factors drive the increase in scans performed and there is the promise of further developments. CT scans are likely to be used increasingly for chest pain, not just for suspected pulmonary embolism, but for suspected acute coronary syndrome, coronary artery disease and aortic dissection as well. Surgeons now wish to avoid unnecessary operations and are increasingly unwilling to take patients for exploratory laparotomies without a CT scan to provide a definitive or tentative diagnosis prior to surgical exploration.
Problems
There are some significant problems that continue to present challenges for physicians requesting CT studies.
Weight constraints
Most scanners have a weight limit, somewhere in the range of 160–200 kg depending on the unit used. Some manufacturers are developing bariatric scanners with much higher weight capabilities, but they are not in common usage at this time.
Unstable patients
A CT suite is usually limited in its provision of resuscitative care. Even with oxygen and suction outlets and full monitoring, these spaces are primarily designed for imaging not resuscitation. Most are some distance from the resuscitation area of the emergency department (ED) and from operating theatres and it represents a challenge for a team to deal with a markedly unstable patient in CT. Although current CT scanners can perform in a short time interval, the process of manoeuvring patients into the room, off the trolley and changing over tubing and monitoring has changed little over the years. These factors are responsible for the bulk of time in the scanner and scanners that can perform a whole body scan in minutes have made surprisingly little difference to the time in the CT scan suite. Patients still die in the relative isolation of these suites and it is still important for physicians to ensure that their patients are as stable as possible prior to leaving the resuscitation area. Patients who cannot be stabilized because of the severity of their injuries should go to the operating theatre rather than be imaged.
Allergy to CT contrast media
There is significant lack of understanding in the medical community regarding reactions to contrast media. Contrast molecules are so small that that they are not capable of acting as antigens and, although they can create an allergic type reaction, it is probably not IgE mediated. Reactions range from minor skin reactions up to more severe anaphylactoid reactions (bronchospasm, angio-oedema, hypotension). IV contrast reactions are more common in atopic individuals and those with previous allergic reactions, but the most important risk factor is a previous reaction to contrast [7]. Determining the incidence of such reactions is difficult because of underreporting and because concomitant illness can produce similar symptoms in some patients. The American College of Radiologists manual on contrast media suggests rates of 0.2–0.7% overall, with the rate of serious reactions of about 1 or 2 per 10 000 [8].
Iodine is an essential element and it is not possible to be allergic to it. Although shellfish are a rich source of iodine, allergy to shellfish is due to the proteins found in the muscle of the shellfish and so the widely held belief that shellfish allergy precludes the use of contrast agents is not based on fact [7].
For patients who are at risk of a reaction, it is wise to have medication to manage a severe response easily to hand. The usefulness of prophylactic steroids for emergency patients is dubious, partly because of the time constraints imposed by the acuity of the illness and partly because of questions surrounding its efficacy. One recommendation suggests:
‘Do not delay emergent studies for steroid premedication. Only lengthy 12-h premedication protocols have shown any effect on reaction rates and this small benefit was manifested primarily by decreasing minor reactions. No steroid protocol has shown a significant benefit in decreasing severe or fatal reactions [9].
Contrast-induced nephropathy
Radiocontrast-induced nephropathy is a feared complication for those receiving IV contrast media. It has been estimated to have a mortality rate of up to 36% in hospital and a 19% 2-year survival, as well as prolonged hospitalization [10–12]. Contrast media have also been estimated to be used in approximately 50% of scans currently performed and CT scan usage is increasing at an exponential rate [13].
Under normal physiological conditions, nearly all of the contrast medium is eliminated through the kidneys. The resulting concentration in the renal tubular system is up to 100 times the concentration in plasma and approaches≤30% of the concentration of the injected solution [14]. With contrast concentrations of this magnitude, it is not surprising that this could be a cause of acute kidney injury.
Recent evidence suggests that some long-held beliefs about contrast-induced nephropathy (CIN) should be challenged. One of the turning points in studies in this area has been the adoption of a more uniform way of diagnosing and describing the illness (accepted basic criteria require a per cent change in the baseline creatinine of≥25% and/or an absolute elevation of creatinine from baseline values of 0.5 mg/dL).
The great majority of previous reports on CIN have been from observations in the cardiac catheterization laboratory. Studies focusing on contrast-enhanced CT show an overall incidence of CIN, with the current generation of non-ionic contrast media, to be in the range of about 5%. In comparison, the rates of serious outcomes generally, and CIN specifically, are much higher for cardiac catheterization. Very recent work examining contrast media away from cardiac catheterization has questioned whether the media induce CIN at all [15]. Traditionally, risks for developing CIN included renal insufficiency, age (older than 55), hypovolaemia, diabetes of longstanding and patients taking metformin among others. On the basis of recent evidence, it appears that baseline renal insufficiency is the only well-supported independent risk factor [13].
Unfortunately, there is no universally agreed threshold of serum creatinine elevation (or degree of renal dysfunction) beyond which intravascular iodinated contrast medium should not be administered.
There have been many agents used as attempted prophylaxis against development of CIN, including N-acetylcysteine (NAC), vasodilators, such as fenoldepam, calcium channel blockers, theophylline, but the only well-accepted measure for at-risk patients is adequate hydration. This position is supported by the American College of Radiology, but the ideal volume and rate of administration is not known. Isotonic fluids, such as normal saline, are preferred. Most guidelines suggest 6–12 hours of infusion prior to the procedure and for up to 4 hours afterwards [16]. Clearly these recommendations are impossible to implement for emergent patients.
Radiation
CT scanning is a source of ionizing radiation, which is capable of overcoming the binding energy of electrons and is able to knock them out of orbit, creating ions. Although X-rays can ionize DNA directly, it is the production of hydroxyl radicals from ionization of water molecules that produces strand breaks and base damage. Although there is some capacity to repair damaged DNA, unresolved damaged DNA can induce cancer. At the doses delivered in normal scanning, there is a small risk of radiation-induced carcinogenesis. This contention is supported by the historical evidence supplied by Japanese survivors of the atomic bombs dropped in 1945. The group who received low doses of radiation in the range 5–150 mSv (mean 40 mSv which approximates the organ dose from a typical CT involving two to three scans in an adult) showed a significant increase in the risk of cancer [17].
Spiral (or helical) CT is rapidly becoming the dominant type of scanner used and, under typical use (with a pitch of 1 or greater), the radiation dose is comparable to conventional CT. Slice thickness, the number of cuts obtained and the pitch affect radiation exposure. Pitch is a ratio defined as the distance the couch travels during one rotation of the radiation source divided by the section thickness [18].
In pregnancy
Radiation damages DNA and a fetus with rapidly dividing and differentiating cells is more susceptible to these effects than adults. Large doses of radiation can lead to growth retardation, birth defects, cancers, mental retardation and even fetal death, but modern diagnostic studies are well below the threshold that would cause such catastrophic effects [19]. Exposure to less than 5 rads has not been associated with deleterious effects on a fetus [17].
In context, an abdominal X-ray exposes a fetus to about 100 mrad, a lumbar spine X-ray to 50–150 mrad, a CT of pelvis to 250 mrad and a CT of abdomen or lumbar spine to 3.5 rad.
Apart from the risks of radiation, there are often questions about the safety of contrast media. It is generally considered safe to give contrast media in all trimesters of pregnancy, although there are theoretical risks of thyroid depression in the fetus/neonate because contrast media molecules are small enough to cross the placental barrier and to be excreted in breast milk. Some centres direct pregnant or lactating women to discard breast milk for up to 24 hours after contrast administration, although this is likely not necessary [19].
In childhood
There has been a marked increase in the use of diagnostic CT scanning in the paediatric population, driven in part by the decrease in time to perform a scan, which eliminates the need for an anaesthetized child to keep them stationary [18].
The largest growth has been in the diagnosis of acute appendicitis, in which CT is cost effective and accurate, although ultrasound represents a viable and safer option in most cases [20].
The increased risk of carcinogenesis is even more marked in the paediatric population for two reasons. They are more radiosensitive and they also have more years of life in which to develop a radiation-induced cancer.
Overuse
Widespread acceptance of the use of CT scanning, the development of new indications, its speed and accuracy in diagnosis, liability issues and the improved access to scanning are among a long list of reasons why the number of scans generated by emergency departments is exploding [21].
While this change is mirrored throughout medical practice, the potential for inappropriate overuse of CT in emergency medicine is an area of concern. Unnecessary scans expose patients to radiation (particularly if there are consecutive or multiple scans done in a short period) and are financially expensive (particularly if compared with modalities like plain X-ray or ultrasound).
Some estimates have suggested a sevenfold increase in total medical radiation exposure from the 1980s to 2006 for the population of the USA. While CT scanning in that period only accounted for 17% of X-ray imaging, they were responsible for 49% of the total estimated dose [22].
In the same population, emergency medicine generates about one-third of the CT scans performed. The benefits for patients in EDs are not disputed and the American College of Radiology sponsored workshop made the following comment:
CT has proven its critical value in the detection and diagnosis of trauma patients and those with chest pain. Diagnosis and management of fatal diseases such as aortic dissection, pulmonary emboli, and others have been revolutionized with CT imaging. The mystery of the ‘blackbox’ of the abdomen has been revealed, improving the diagnosis of conditions such as bowel obstruction, bowel necrosis, ureteral stones, appendicitis, pancreatitis, diverticulitis, pyelonephritis, and so on. CT scans also became the principal modality for evaluating intracranial problems and, to an ever increasing extent, the diagnosis of cancers [20].
Some studies suggest that there are significant numbers of inappropriate tests ordered from emergency departments. Studdert et al. found that 70% of the emergency physicians responding to their questionnaire admitted to ordering imaging that they did not feel was clinically indicated because of the threat of medical liability [23].
Emergency physicians respond by pointing out that, although the CT radiation dose is significant (10–20 millisieverts (mSv), which is associated with a lifetime risk of fatal cancer of about one per 2000 scans), more than one in 2000 patients will have potentially life-saving information provided by a CT. To many frontline doctors, the long-term risks are theoretical and poorly quantified compared with the risk of missing significant pathology in the here-and-now [24].
One area of medicine in which CT scanning has revolutionized care is trauma management. Rapid, high-quality scanning has unequivocally led to better outcomes in many patients but, even here, questions are being asked. The pan-scan has been enthusiastically embraced and quickly become a standard of care before any chance of rigorous scientific evaluation [25].
An Australian study reported the results of a large cohort study comparing radiation exposure to trauma patients before and after the introduction of a pan-scan (whole body scan for trauma) diagnostic algorithm employing a 64-slice scanner [26]. Their findings were challenging, suggesting that patients undergoing a pan-scan, according to the calculated risk ratio, were 1.7 (95% confidence interval [CI] 1.3–2.2) times more likely to receive a radiation dose exceeding 20 mSv compared with a conventional CT work-up, but did not significantly benefit from the procedure in terms of the incidence of missed injuries (0.6% vs 0.9%).
Only one-fifth of the patients in the study fulfilled the criteria for major trauma, the major justification for a pan-scan. The authors went on to suggest that the pan-scan may be 26 times more likely to harm the patient in the long term than assist them in the acute setting.
CT scanning should not be requested in a frivolous fashion, without regard for potential long-term health risks. The speed of the investigation and the quality of the diagnostic information should always be balanced against a knowledge that the scan may harm the patient.
Clinical decision rules
Emergency physicians are responsible for ensuring that they make sensible and appropriate decisions for their patients and that their choice of CT as a modality takes account of the risks to the patient. It is unfortunately true that there has been such a proliferation of guidelines that sometimes clinicians are hard pressed to know which one to follow. There are, for example, no less than three widely accepted and well-validated decision rules for determining the need for CT in head injury (Canadian Head CT rule/New Orleans Criteria/NEXUS II). The American College of Radiologists has developed a comprehensive list of appropriateness criteria that relate possible investigations to the presenting complaint, covering the full gamut of clinical presentations and made in conjunction with appropriate input from clinicians [27].
Diagnostic accuracy of emergency physicians
Emergency physicians are often required to interpret a CT scan. Early studies did not suggest this was done well. Many studies have examined the ability of emergency physicians (EPs) and registrars (EM residents in North America) to interpret head CT scans. In the specific clinical setting of stroke, compared to the gold standard of interpretation by neuroradiologists, EPs performed relatively poorly in recognition of both haemorrhage and early ischaemic changes (accuracy 60%), but neurologists and general radiologists only achieved a result of 80% [28–30].
A study examining EPs’ abilities to assess head CT for trauma concluded that, without extra training, EPs should not be interpreting such scans [30]. While there is debate about whether the non-concordance between EPs and radiologists leads to poor clinical outcomes, there are numbers of studies that demonstrate the improvement that is possible with focused teaching [31].
There is no doubt that improvement in training must occur because, despite the advent of teleradiology, there will still be occasions when the situation dictates that an EP perform the initial interpretation of a CT scan.
Future directions
Low-dose CT scanning is an attempt to produce high-quality scans at lower doses of radiation. It is achieved by a variety of techniques, including dose modulation, noise management software (to filter out unnecessary data), lower peak voltage settings and switching off the tube at some points of its rotation and iterative dose reduction techniques. As an area of rapid development, the trade off in image quality versus radiation dose is becoming less of an issue.
23.3 Magnetic resonance imaging in emergency medicine
James Rippey
Introduction
Magnetic resonance imaging (MRI), like each of the other imaging modalities, has its unique strengths and weaknesses. Emergency diagnostic imaging algorithms are complex and vary according to the clinical condition, the question being asked, patient factors, local availability and expertise. In Australasia, there are rapidly increasing numbers of MRI machines, together with appropriately trained staff. Many diagnostic algorithms are being revised to include MRI as a realistic imaging alternative. While the indications for urgent MRI are increasing, the major indication for emergency MRI remains suspected acute spinal cord pathology.
The main strength of MRI lies in its ability to image soft tissues at extremely high resolution, both spatially and with unparalleled levels of soft tissue contrast. It also has the ability to create two-dimensional slices in any plane; these multiplanar capabilities allow comparison of adjacent tissues from any angle.
Different MRI techniques allow different anatomical and pathological features to be demonstrated. MRI is particularly good at imaging the structure and pathology of brain, spinal cord and nerves, muscle, tendons and ligaments, cartilage, bone marrow and solid abdominal organs. The advent of magnetic resonance angiography (MRA) makes it an alternative to computed tomography angiography (CTA), particularly in those with contraindications to CT contrast media or those more vulnerable to ionizing radiation. Finally, the ability to perform ECG gated imaging has enabled unsurpassed dynamic non-invasive cardiac imaging.
MRI has imaging limitations in cortical bone and air-filled spaces (particularly lung) and thus tends not to be the imaging choice for assessing these tissues.
The lack of ionizing radiation makes MRI an attractive alternative to CT, particularly in younger patients, especially children and women of childbearing age. MRI’s apparent safety in pregnancy is another advantage.
Currently, the main limitations of MRI lie in its lack of availability, expertise and associated high costs. From the emergency perspective, even when availability is not an issue, a major disadvantage is the time it takes to complete a scan. The patient is in an inaccessible, confined space, usually with limited monitoring for the duration of the 30–60 minute scan. MRI is therefore unsuitable for the unstable patient. If a patient is stable and intubated, specialized anaesthetic and monitoring equipment and expertise in using it, is required to ensure patient safety.
Patients with metallic foreign bodies or electronic implants are often unable to have MRI, which can also limit its use.
Technical issues
Put very simply, the steps of an MRI involve putting the patient into a powerful magnet, sending in a radiowave, turning the radiowave off, the patient then emits a signal which is received and used for reconstruction of the image.
The components
The traditional MRI suite is centred around the MRI scanner, with its mobile patient table that moves the patient in and out of the MRI tunnel (Fig. 23.3.1). Current machines have a bore (internal diameter of the tunnel) of up to 70 cm and utilize short bore architecture that allows the tunnel to be approximately half the length of that required in the previous generation of MRI scanners. The machine houses a superconductor magnet, the strength of which is measured in Tesla. Current machines operate at 1.5 or 3 Tesla. A 3 Tesla magnet creates a magnetic field around the patient 60 000 times the strength of the earth’s magnetic field. As well as the magnet, there are radiofrequency transmitter and receiver coils that send and receive radiofrequency pulses. These briefly disturb the magnetic field and ultimately create the MRI image. Another three sets of gradient coils provide additional linear electromagnetic fields important for spatial information – determining the origin of the signal in the 3-dimensional space. It is these coils banging against their anchoring devices that cause the loud noises associated with MRI.
The high magnetic field generated by MRI means metallic objects within range can become projectile missiles and great care has to be taken to ensure metal objects are well secured or do not enter the room. The magnet can also interfere with electronic equipment, such as computers, monitors and medical equipment like pacemakers and these must be kept away. Finally, the receiver coils are highly sensitive and are designed to detect very minor fluctuations in returning radiowaves (which are a form of electromagnetic radiation). External radiowaves can interfere with the waves received by the coils and this noise will create artefacts interfering with image production. To minimize this, the entire MRI room is secured inside a Faraday cage, whose external conducting surface blocks or markedly attenuates any outside potentially interfering radiowaves. The MRI control room and computer terminals with operating console are located immediately adjacent to, but outside the MRI room, in a similar fashion to the CT control room.
Creating an image
MRI depends on the alignment of hydrogen nuclei or positively charged protons within organic compounds in the body. Hydrogen nuclei act like tiny bar magnets. Under the influence of the external MRI magnet, mobile hydrogen ions align and spin in the orientation of the MRI magnet’s field, creating a magnet of the patient’s body. As well as aligning and spinning on their own axis, protons also rotate or ‘precess’ as would a spinning top with a slight wobble, around a central axis.
Pulses of electromagnetic energy, called radiofrequency or RF pulses, are then sent into the area being imaged. This briefly disturbs the orientation and precession of the aligned protons. A transient reduction in the longitudinal magnetic field results and a new magnetic vector in the transverse direction, called transversal magnetization, is created. Once the RF pulse is stopped, the protons relax back to their initial aligned state and the longitudinal and transverse magnetic vectors return to their original state. The realignment rate depends on tissue characteristics and water content. As the magnetic vectors realign, electric currents are induced and the MRI signal and signal intensity created. The receiver coils receive these minute pulses of newly created electromagnetic radiation and these are interpreted to create the ultimate image.
Different MRI imaging techniques
Numerous different MRI imaging sequences and techniques have been developed to create the optimal images for varying body tissues and pathology. The following is not an exhaustive list.
T1- and T2-imaging
These are the most common MRI images with which we are familiar.
T1-weighted images (anatomical) create high definition anatomical images with optimal tissue contrast resolution. In these images, fat is white and water is black. The resultant image gives detailed representation of the internal structure of soft tissue organs. T1 is a time constant that refers to the time it takes for the changes in longitudinal magnetization induced by the RF pulse, to return toward the original state. Measuring this tends to define structural tissue proteins and fats optimally.
T2-weighted images (pathological) highlight pathological processes where there is increased water content within tissues (Fig. 23.3.2). Most pathological processes involve an element of tissue oedema and whether it be trauma, infection, infarction or neoplasia, these images highlight water. Water is seen as white in these images. T2 is a time constant that refers to the time it takes for the changes in transversal magnetization induced by the RF pulse, to return toward their initial state. Measuring this tends to highlight water optimally.
Other MRI techniques each aimed at highlighting other anatomical or pathological features are shown in Figure 23.3.3. The left image (A) is a FLAIR (fluid attenuated inversion recovery) sequence that nulls fluid and can highlight periventricular demyelination; the central image (B) is a T2 gradient image which detects haemoglobin and its breakdown products; the right hand image (C) is a diffusion ADC image detecting cell injury in early stroke.
Angiography and gadolinium
Magnetic resonance angiography (MRA) can be done with or without contrast media (Fig. 23.3.4 shows non-contrast MRI). Flow itself alters the MR signal simply by moving the protons that have been exposed to the RF pulse. This can leave what is called a flow void phenomenon and, using this, the machine can create an angiographic image.
Where a contrast agent is used, the paramagnetic rare earth, gadolinium (Gd), is the agent of choice. Its use creates excellent angiographic images. In addition, gadolinium does not cross the normal blood–brain barrier. If, however, this is disrupted, as can occur in many pathological processes, gadolinium improves lesion detection and diagnostic accuracy. Gadolinium is not an iodinated contrast medium and is generally very well tolerated.
The sagittal images of the lumbar spine shown in Figure 23.3.5 demonstrate a tumour involving the L1, causing some cord compression. The left image is T1, the centre T2 and the right a T1 fat-saturated post-gadolinium image where the tumour with its abnormal vasculature is most obvious.
Diffusion and perfusion weighted imaging
MRI imaging changes occur early after stroke and can be detected prior to any visible change on CT. Diffusion weighted imaging assesses water diffusion across cell membranes. There is no water movement across cell membranes when cells are damaged. Diffusion imaging is used to define areas of newly infarcted cerebral tissue. These changes can occur as early as 10 minutes after infarction. Perfusion imaging aims to detect the potentially salvageable cerebrovascular accident ‘penumbra’ surrounding the non-viable ischaemic core, with a view to decision making regarding thrombolysis and revascularization.
Cardiac imaging
Cardiac imaging is done by using ECG gating and imaging the heart over several cardiac cycles. Sequentially timed images taken from separate cardiac cycles are stitched together to create an animation representative of a single cardiac cycle. The result is a very high resolution, dynamic study of heart function and blood flow within the heart (Fig. 23.3.6).
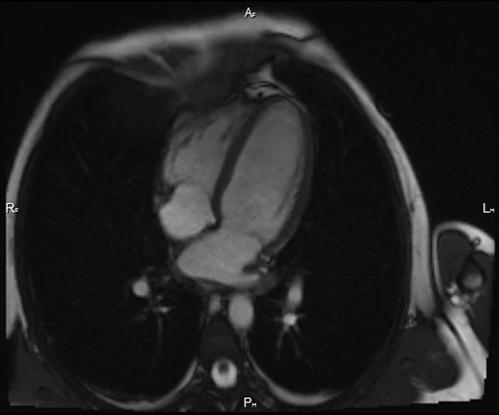
Monitoring patients in the MRI
The patient in the MRI suite is particularly vulnerable. Their physical movement is restricted while in the MRI tunnel, they are inaccessible to immediate assistance and there are particular challenges with traditional patient monitoring in the MRI environment. This makes MRI unsuitable for the unstable patient and makes those requiring sedation or general anaesthesia a far greater challenge.
As a minimum requirement, visual camera and verbal monitoring are mandated for all MRI machines, ensuring some degree of immediate communication between radiographer and patient.
If any sedation is required, it is essential the patient have oxygen saturation monitoring. There are MRI compatible units, with no ferromagnetic components, often fibreoptic, suited to this purpose and available in most units.
Patients who are under general anaesthetic require highly specialized anaesthetic staff and equipment. This form of remote anaesthesia requires unique training and practice. MRI compatible and prepared equipment is required. This includes specially prepared ventilators and monitors. Attention to detail is required regarding all the equipment involved. Even inappropriate ECG dots and leads can heat and cause injury.
Indications for MRI
In most parts of the world, access to MRI, with its great cost and requirement for highly specialized radiography and radiology staff, is extremely limited. Australasia falls into the category where truly emergent MRI requests can usually be met by most tertiary hospitals. Arranging the scan in a public hospital generally requires consultant-to-consultant discussion and involvement of the inpatient specialty team.
Where there are reasonable imaging alternatives to MRI, diagnostic imaging guidelines have been designed to create effective alternative pathways.
Suspected acute spinal cord and cauda equina pathology
There is little argument that emergency MRI is the investigation of choice when it comes to imaging the spinal cord, spinal nerves, intervertebral discs and ligaments of the spine. Where there are long tract signs and suspicion of an acute spinal cord-threatening lesion, most would proceed directly to MRI. In the setting of trauma, a CT to define bony injury is usually performed prior to MRI.
MRI clearly defines any pathological process affecting the cord, CSF space and surrounding soft tissues. In the emergency setting, trauma with cord injury (Fig. 23.3.7) or contusion may occur and MRI gives additional information regarding spinal ligamentous injury. Other common cord-threatening pathology includes malignancy, which may originate in the vertebrae, most commonly the bodies, and extend into the spinal canal or may invade the canal through the spinal foramina. Malignant deposits, particularly metastases, may also originate within the spinal canal, involving the cord or dura. Infective processes, such as discitis and epidural abcess, are not infrequent causes of acute cord compression and are more common in IV drug users, the immunosuppressed and those who have had spinal procedures. Vascular phenomena, such as epidural haematoma, AV malformation with bleed, aneurysms and spinal cord infarction can all be defined by MRI. Degenerative conditions, such as disc prolapse (Fig. 23.3.8) and spinal canal stenosis from any cause may also threaten the cord.
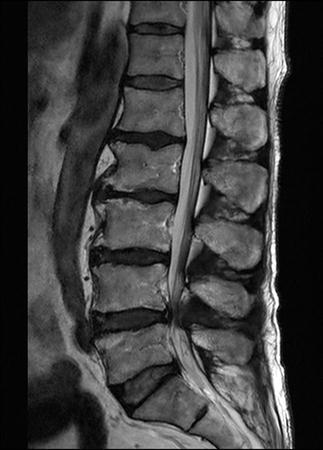
Rapidly defining the cause of the spinal cord lesion enables surgical planning or, if a malignant process, consideration and planning for radiotherapy.
Stroke
MRI is an excellent modality for defining any brain pathology and stroke is no exception. As well as confirming the presence of stroke and excluding the presence of haemorrhage, MRI can detail the extent of brain injury, the vascular supply, any ischaemic penumbra and can assess for dissection or other predisposing vascular causes.
Unfortunately, even if immediately available, performing an MRI and then preparing and reporting the images often puts the patient outside the window of benefit for thrombolysis. CT is far more accessible and provides adequate information for the majority of cases and this is currently the accepted gold standard.
The exception is the posterior circulation and suspected brainstem infarction where MRI is superior to CT and generally required before interventional attempts at revascularization.
Headache
CT scan is the first choice for investigation of headache. MRI is performed where consideration for intervention for brain tumours, acoustic neuromas and pituitary tumours is being made. Where patients are young or cannot have iodinated contrast and sinus venous thrombosis is being considered, MR venography would be performed over CT venography; however, in most people, CT is adequate to define sinus venous thrombosis and other sinister causes of headache.
Angiography
MR angiography and CT angiography are considered similar in their utility for imaging blood vessels. Dissection is well imaged with either modality and factors that would swing one in favour of MRI include a young patient age (MRI avoids radiation) and allergy to CT contrast.
Occult fracture detection
In the Australasian setting, it is unusual to attain an MRI to assess for occult fracture. Hip and scaphoid fractures are the classic examples where early detection and intervention can benefit the patient. CT scan has lower sensitivity than MRI for detecting occult hip fractures, but it remains reasonable. Where concern remains, a bone scan will give the answer. Similarly, for scaphoid fractures, CT or bone scan or immobilization and delayed repeat plain films at 10 days are reasonable alternatives.
Soft-tissue musculoskeletal injury
MRI is increasingly being used to assess for soft-tissue injury when complex injuries are suspected. Requests generally come from the orthopaedic team involved in the patient’s care. MRI can image injuries to muscles, tendons, ligaments, joint capsule and cartilaginous surfaces extremely well (Fig. 23.3.9). Acute shoulder and knee injuries are most commonly assessed with MRI, but complex elbow, wrist, foot and ankle injuries can also be assessed by MRI.
Where there is complex fracture/dislocation and the relationship of adjacent bones and bone fragments need defining, CT is more appropriate.
Magnetic resonance cholangiopancreatogram (MRCP)
MRI can image the liver and biliary tree well. Ultrasound is generally the first imaging modality used to investigate for biliary pathology. Where concern remains and ultrasound imaging has not been definitive, MRCP can help. This is most common with distal biliary obstruction where ultrasound has not been able to image the extreme distal common bile duct. An effective alternative is endoscopic retrograde cholangiopancreatogram (ERCP) and endoscopic ultrasound. The advantage of ERCP is that therapeutic interventions, such as stone retrieval or stenting, can be carried out at the same time.
Appendicitis in pregnancy
Ultrasound is the first indicated investigation. MRI may sometimes be used where there is diagnostic uncertainty.
Contraindications, precautions and limitations
There are numerous contraindications to MRI and pre-MRI safety checklists can be found at www.mrisafety.com. MRI technicians should be made aware of any implanted metallic, prosthetic, electronic or drug delivery device. The pre-MRI questionnaire also covers past medical history, particularly renal dysfunction and allergies.
Ferrous and metallic materials
The high magnetic field generated by MRI can move and heat metallic materials. While most joint and heart valve prostheses are now MRI compatible, older prostheses, implanted metallic medical devices, aneurysm clips and metallic shrapnel, especially intraocular metallic foreign bodies, are contraindications to MRI.
Metallic drug transdermal drug infusion patches often contain metal and can heat.
Some tattoos and permanent makeup contain iron oxide and can heat in the MRI environment, although this is rare.
Electronic implants
The intense magnetic field can affect electronic and magnetic equipment. Cardiac pacemakers, implantable cardioverter defibrillators, implanted nerve stimulators, cochlear implants and other electronic implants can be affected. Some are now MRI-compatible, however, most are a contraindication to MRI. The website www.mrisafety.com has lists of thousands of implants and devices that have been tested in MRI machines and is recommended by RANZCR as a resource for obtaining information on patient’s implanted devices.
Noise
The MRI is very loud, with constant banging heard. Ear protection is recommended.
Pregnancy
There has been no demonstrated adverse effect from MRI or gadolinium-based contrast media to the mother or embryo/fetus. The evidence in this setting, however, is limited and, with regard to MRI exposure, the ALARA principle (as low as reasonably acceptable) is followed. MRI should only be pursued where potential benefits outweigh the risks. Generally, it is considered prudent to avoid MRI in the first trimester.
Gadolinium
Gadolinium can rarely cause nephrogenic systemic fibrosis (NSF). This disabling disease is more likely in patients with underlying renal disease. It is recommended that patients over 60, those with hypertension or diabetes, a history of renal disease (including transplant or a single kidney), those within a month of a liver transplant or those with an acute deterioration in renal function have renal function tested prior to MRI. If their renal function is not normal, further discussion and consideration of the risk benefit ratio of gadolinium should be made in conjunction with the MRI radiologists.
Weight and size limits
Different MRI machines can tolerate different patient weight and size limits. Some tolerate patients up to 250 kg. Occasionally, it is the patient diameter or shape rather than absolute weight that limits entry to the MRI tunnel.
Claustrophobia
The MRI tunnel frequently causes claustrophobia. Non-pharmaceutical management may include patient education, allowing a patient companion to accompany the patient, continuous verbal contact, headphones with audio, use of prone and or feet first positioning, use of a blindfold, fan, bright lights, aromas or other relaxation techniques or watching videos or movies via mirrors.
Despite these measures, sedation is sometimes required and should be done only with oxygen and saturation monitoring and a single dedicated and trained person responsible to supervise and monitor the sedation.
Conclusion
The increasing availability of MRI within Australasia, together with improving imaging times and a diverse range of MRI techniques, mean MRI is becoming the imaging modality of choice for an increasing number of indications.
Currently, it is generally used in conjunction with other imaging modalities, particularly if they have been unsuccessful, in imaging soft-tissue structures and pathology.
The required isolation of the patient for the duration of the scan and difficulty in monitoring and managing anaesthetized patients in the MRI, mean it is not the place for unstable critically unwell patients.
Acknowledgements
The author declares no conflict of interest.
Thanks to the MRI unit at Sir Charles Gairdner Hospital for their advice on emergency MRI and the images, particularly Dr Rohan Van den Driesen, Dr Andrew Thompson and Ms Anne Windsor.