CHAPTER 56 Pancreatic Secretion
As has been reviewed in Chapter 55, the pancreas is an exocrine organ as well as an endocrine organ. This chapter is devoted to the exocrine pancreas. The exocrine pancreas has been of considerable interest to physiologists and other scientists for quite some time; in fact, the first demonstration of a hormone action was in the pancreas around the turn of the 20th century.1 The pancreas has been the major organ used to demonstrate the mechanisms of synthesis and transport for exportable proteins2 as well as the signaling pathways involved in regulated protein secretion.3 This chapter presents a concise description of the current understanding of pancreatic physiology.
FUNCTIONAL ANATOMY
The functional unit of the exocrine pancreas is composed of an acinus and its draining ductule (Fig. 56-1).4 The ductal epithelium extends to the lumen of the acinus with the centroacinar cell situated between the ductal epithelium and the acinus. The function of centroacinar is not established but may play a role in providing progenitor cells for pancreatic cell lineages. The ductule drains into interlobular (intercalated) ducts, which in turn drain into the main pancreatic ductal system.
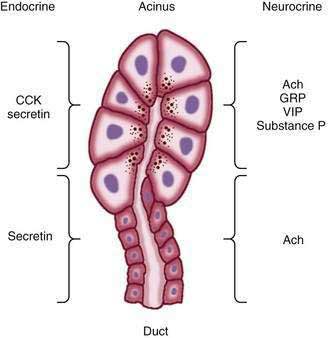
Figure 56-1. Regulation of exocrine pancreatic secretion during the intestinal phase of digestion. Exocrine secretions are regulated by endocrine and neurocrine pathways. In addition, the endocrine and neurocrine mediators regulate secretion from the acinus and the duct differ. For the acinus, cholecystokinin (CCK), secretin, acetylcholine (Ach), gastrin-releasing peptide (GRP), vasoactive intestinal polypeptide (VIP), and substance P regulate secretion. (Signaling mechanisms [second messengers] are shown in Fig. 56-6.) Secretin and Ach are the major regulators of pancreatic bicarbonate secretion from the duct. (Transporters involved in ductal secretion are illustrated in Fig. 56-4.)
(Adapted from Gorelick F, Pandol SJ, Topazian M. Pancreatic physiology, pathophysiology, acute and chronic pancreatitis. Gastrointestinal Teaching Project, American Gastroenterological Association, 2003.)
The acinus (from the Latin term meaning “berry in a cluster”) can be spherical or tubular, as shown in Figure 56-1, or can have some other irregular form.4 The acinar cells are specialized to synthesize, store, and secrete digestive enzymes. On the basolateral membrane are receptors for hormones and neurotransmitters that stimulate secretion of the enzymes.3 The basal aspect of the cell contains the nucleus as well as abundant rough endoplasmic reticulum for protein synthesis (Fig. 56-2, left). The apical region of the cell contains zymogen granules, the store of digestive enzymes. The apical surface of the acinar cell also possesses microvilli. Within the microvilli and in the cytoplasm underlying the apical plasma membrane is a filamentous actin meshwork that is involved in exocytosis of the contents of the zymogen granules.5,6 Secretion is into the lumen of the acinus. Tight junctions between acinar cells form a band around the apical aspects of the cells and act as a barrier to prevent passage of large molecules such as the digestive enzymes.7 The junctional complexes also provide for the paracellular passage of water and ions.
Another intercellular connection between acinar cells is the gap junction. This specialized area of the plasma membrane between adjacent cells acts as a pore to allow small molecules (molecular weight 500 to 1000 Da) to pass between cells. The gap junction allows chemical and electrical communication between cells.3 For example, calcium signaling is coordinated between the cells of an acinus with effects on digestive enzyme secretion.8,9
The duct epithelium consists of cells that are cuboidal to pyramidal and contain the abundant mitochondria necessary for energy products needed for ion transport (see Fig. 56-2, right). The duct cells as well as the centroacinar cells contain carbonic anhydrase, which is important for their ability to secrete bicarbonate.10
COMPOSITION OF EXOCRINE SECRETIONS
INORGANIC CONSTITUENTS
The principal inorganic components of exocrine pancreatic secretions are water, sodium, potassium, chloride, and bicarbonate (Fig. 56-3). The purposes of the water and ion secretions are to deliver digestive enzymes to the intestinal lumen and to help neutralize gastric acid emptied into the duodenum. Pancreatic juice secreted during stimulation with secretin is clear, colorless, alkaline, and isotonic with plasma. The flow rate increases from an average rate of 0.2 or 0.3 mL/minute in the resting (interdigestive) state to 4.0 mL/minute during postprandial stimulation. The total daily volume of secretion is 2.5 L. The osmolality of pancreatic juice is independent of flow rate. However, when the pancreas is stimulated by secretin (the major mediator of the greater volume output), bicarbonate and chloride concentrations change reciprocally (see Fig. 56-3).
Secretin stimulates secretion by binding to its receptor on the acinar cell and activating adenylate cyclase and increasing cyclic adenosine monophosphate (cAMP) in the ductal cell; acetylcholine does so by binding to its receptor and raising intracellular calcium concentrations. The initial events involve cAMP-dependent and Ca2+-dependent chloride (Cl−) channel activation on the luminal membrane as well as K+ channel activation on the basolateral membrane (Fig. 56-4).10–12 The cAMP-dependent Cl− channel is the cystic fibrosis transmembrane conductance regulator (CFTR).10–13 The activation of both channels by cAMP leads to Cl− secretion into the lumen. The higher chloride concentration in the lumen is coupled to a Cl−-HCO3− antiport, resulting in an exchange of Cl− for HCO3− in the lumen. Newer evidence also suggests a HCO3− channel on the apical surface involved in HCO3− secretion and that this HCO3− conductance is likely CFTR.11,12 On the basolateral surface of the duct cell are a Na+,H+ antiport and a Na+,HCO3− cotransport, as well as ATPases (Na+,K+-ATPase, H+-ATPase) and K+ channels. In combination, these transporters facilitate HCO3− secretion at the apical surface as well as maintain intracellular pH.14 Na+ and water are secreted into the ductal system to counter the electrical and osmotic forces resulting from the HCO3− secretion.
ORGANIC CONSTITUENTS15–17
The human pancreas has a large capacity for synthesizing protein (mostly digestive enzymes). Table 56-1 lists the major proteolytic, amylolytic, lipolytic, and nuclease digestive enzymes. Some of the enzymes are present in more than one form (e.g., cationic trypsinogen, anionic trypsinogen, and mesotrypsinogen). Enzymes that could digest the pancreas are stored in the pancreas and secreted into the pancreatic duct as inactive precursor forms. As illustrated in Figure 56-5, activation of these enzymes takes place in the intestinal lumen, where a brush-border glycoprotein peptidase, enterokinase, activates trypsinogen by removing (by hydrolysis) an N-terminal hexapeptide fragment of the molecule (Val-Asp-Asp-Asp-Asp-Lys).17 The active form, trypsin, then catalyzes the activation of the other inactive proenzymes.
Proenzymes* | Cationic trypsinogen |
Anionic trypsinogen | |
Mesotrypsinogen | |
Chymotrypsinogen (A, B) | |
Kallireinogen | |
Procarboxypeptidase A (1, 2) | |
Procarboxypeptidase B (1, 2) | |
Prophospholipase | |
Proelastase | |
Enzymes | Amylase |
Carboxylesterase | |
Sterol esterase | |
Lipase | |
DNase | |
RNase |
* Proenzymes listed are stored in the pancreas and secreted into the duodenal lumen as inactive proenzyme forms. If these enzymes were active in the pancreas, they would digest the pancreatic gland. Other enzymes, such as amylase and lipase, are stored and secreted in their active forms.
Adapted from Gorelick F, Pandol SJ, Topazian M. Pancreatic physiology, pathophysiology, acute and chronic pancreatitis. Gastrointestinal Teaching Project, American Gastroenterological Association, 2003.
In addition to the digestive enzymes, the acinar cell secretes a trypsin inhibitor, pancreatic secretory trypsin inhibitor (PSTI). This 56–amino acid peptide inactivates trypsin by forming a relatively stable complex with the enzyme near its catalytic site.18 The function of the inhibitor is to inactivate trypsins that are formed autocatalytically in the pancreas or pancreatic juice, thus preventing disorders such as pancreatitis.19
FUNCTIONS OF THE MAJOR DIGESTIVE ENZYMES
AMYLASE
Human amylase is secreted by both the pancreas and salivary glands. These enzymes digest starch and glycogen in the diet. Human salivary and pancreatic amylases have identical enzyme activities. However, they differ in molecular weight, carbohydrate content, and electrophoretic mobility.20 Salivary amylase initiates digestion in the mouth and may account for a significant portion of starch and glycogen digestion because it is transported with the meal into the stomach and small intestine, where it continues to have activity. In the stomach, the amylase activity is protected from secreted gastric acid by buffering from the meal and by the protected alkaline environment of salivary and gastric mucus. The action of both salivary and pancreatic amylase is to hydrolyze 1,4-glycoside linkages at every other junction between carbon 1 and oxygen. The products of amylase digestion are maltose and maltotriose (two- and three-α-1,4-linked molecules, respectively) and α-dextrins containing 1,6-glycosidic linkages, because 1,6-glycosidic linkages in starch cannot be hydrolyzed by amylase. The brush-border enzymes complete hydrolysis of the products of amylase digestion to glucose. The final product, glucose, is transported across the intestinal absorptive epithelial cell by a Na+-coupled transport (see Chapter 100).21,22
LIPASES
The pancreas secretes three lipases: lipase (or triglyceride lipase), phospholipase A2, and carboxylesterase (see Table 56-1). The most important source of these lipases, in contrast to amylase, is the pancreas. Salivary (lingual) and gastric lipases also contribute to fat digestion but in a minor fashion (see Chapter 49).
Pancreatic lipase hydrolyzes a triglyceride molecule to two fatty acid molecules released from carbons 1 and 3 and a monoglyceride with a fatty acid esterified to glycerol at carbon 2.16,23 Lipase binds to the oil-water interface of the triglyceride oil droplet, where it acts to hydrolyze the triglyceride. Bile acids as well as colipase are important for the full activity of lipase. Bile acids aid in the emulsification of triglyceride to enlarge the surface area for lipase to act on, and they form micelles with fatty acids and monoglyceride, which in turn remove these products from the oil-water interface. Colipase is believed to form a complex with lipase and bile salts. This ternary complex anchors lipase and allows it to act in a more hydrophilic environment on the hydrophobic surface of the oil droplet.
Phospholipase A2 catalyzes the hydrolysis of the fatty acid ester linkage at carbon 2 of phosphatidylcholine.16 This cleavage leads to the formation of free fatty acid and lysophosphatidylcholine.
Carboxylesterase has a broad specificity and will cleave cholesterol esters, lipid-soluble vitamin esters, triglycerides, diglycerides, and monoglycerides. Bile salts are also important for the full activity of this enzyme.24
PROTEASES
The combined actions of the pancreatic proteases and pepsin from the stomach result in the formation of oligopeptides and free amino acids. The oligopeptides are further digested by brush-border enzymes (see Chapter 100). Free amino acids and oligopeptides are transported across the intestinal mucosa by a group of Na+– and H+-coupled transporters.25 It is interesting that only certain amino acids (mostly essential amino acids) can be measured in the lumen during digestion, indicating that the combined action of the proteases is not random and that the products result from the combined specificities of the individual proteases. These amino acids have greater effects on stimulating pancreatic secretion, inhibiting gastric emptying, regulating small bowel motility, and causing satiety. Thus, the specific pattern of protease actions leads to physiologic regulation of several organs in the gastrointestinal tract.
DIGESTIVE ENZYME SYNTHESIS AND TRANSPORT
Synthesis of digestive enzymes takes place in the internal space of the rough endoplasmic reticulum (RER) (see Fig. 56-2, left).2,26 The mechanism for translation of the cell’s messenger RNA (mRNA) into exportable protein is explained by the signal hypothesis. The main feature of the hypothesis is that ribosomal subunits attach to mRNA and initiate synthesis of a hydrophobic “signal” sequence on the NH2-terminal of nascent proteins. This complex then attaches to the outer surface of the endoplasmic reticulum, and the signal sequence targets the protein being synthesized into the lumen of the RER.
Newly synthesized proteins can undergo modifications in the endoplasmic reticulum, including disulfide bridge formation, phosphorylation, sulfation, and glycosylation. Conformational changes resulting in tertiary and quaternary structures of the protein also take place in the endoplasmic reticulum. Processed proteins in the RER are transported to the Golgi complex where further post-translational modification (glycosylation) and concentration occur.27 Important conformational specificities are required for appropriate function and transport of proteins between compartments. These conformational specificities are facilitated by proteins called chaperones and foldases among others. When there is an increased demand on the RER for protein synthesis or when there is a cell stress that causes unfolding of proteins, the RER responds by increasing synthesis of chaperones and foldases, ceases synthesis of the proteins, and even degrades unfolded proteins using the mechanisms of the unfolded protein response (UPR).28 By keeping proteins in their appropriate conformation, the UPR maintains normal transport of proteins between compartments of the cell.
The Golgi complex also serves the important function of sorting and targeting newly synthesized proteins into various cell compartments. Digestive enzymes are transported to the zymogen granules.27 Lysosomal hydrolases are sorted to the lysosome.29 For this lysosomal pathway, mannose-6-phosphate groups are added to oligosaccharide chains on the protein during its presence in the cis-Golgi complex. The mannose-6-phosphate groups serve as a recognition site for a specific receptor. The interaction of the lysosomal enzyme mannose 6-phosphate with its receptor leads to formation of vesicles that transport this complex to the lysosome, delivering the enzyme. In the lysosome the enzyme dissociates from the receptor, which in turn cycles back to the Golgi complex.
Secretion of the digestive enzymes occurs by exocytosis. Exocytosis consists of movement of the secretory granule to the apical surface, the recognition of a plasma membrane site for fusion, and the fission of the granule membrane-plasma membrane site after fusion.2,30 Recent studies demonstrate roles for actin-myosin, SNARE (soluble N-ethylmaleimide–sensitive factor attachment protein [SNAP] receptor) proteins, and guanosine triphosphate (GTP)-binding proteins in these processes.30–38 Intracellular signals generated by agonist receptors as discussed following interact with these entities to mediate digestive enzyme secretion via exocytosis of zymogen granules.
REGULATION OF PROTEIN SYNTHESIS
The mechanisms involved in regulating expression of digestive enzymes in the exocrine pancreas have been partially elucidated. The investigations have addressed the following two questions: First, what accounts for the specific expression of digestive enzymes in the pancreas? Second, how do alterations in dietary nutrients change the synthesis of specific digestive enzymes? Genes for digestive enzymes such as amylase, chymotrypsin, and elastase contain enhancer regions in their 5′ flanking nucleotide sequences that regulate the transcription of their mRNAs, termed the pancreas consensus element (PCE).39,40 A transcription factor, pancreas transcription factor-1 (PTF-1), is present selectively in the exocrine pancreas, binds to this region, and is essential for expression of these digestive enzymes.41–44 Thus, PTF-1 represents at least one of the differentiation-regulated factors that accounts for digestive enzyme expression in the pancreas.
Numerous studies have demonstrated that the relative synthesis rates of specific digestive enzymes change as a function of dietary intake. For example, a carbohydrate-rich diet results in an increase in synthesis of amylase and a decrease in that of chymotrypsinogen45; a lipid-rich diet enhances lipase expression46; and an alcohol-rich diet decreases amylase expression.47 The mechanisms responsible for this adaptation are only partially understood. The regulation occurs at the level of gene transcription in many of these conditions.47 Several studies have also demonstrated that amylase gene expression is regulated by insulin and diet.45
CELLULAR REGULATION OF ENZYME SECRETION
With the use of radiolabeled ligands and specific antagonists, receptors for cholecystokinin (CCK), acetylcholine, gastrin-releasing peptide (GRP), substance P, vasoactive intestinal peptide (VIP), and secretin have been identified in preparations from several species.48–50 Furthermore, the molecular structure for each of these receptor types has been elucidated from cloning and sequencing. Each is a G-protein–coupled receptor with seven hydrophobic domains believed to be membrane-spanning segments. The receptors are on the basolateral plasma membrane of the acinar cell.
Receptors on acinar cells have been divided into two categories according to the mode of stimulus-secretion coupling (Fig. 56-6). In one category are VIP and secretin. The interaction of these agents with acinar cells leads to activation of adenylate cyclase and a rise in cellular cAMP, which in turn activates enzyme secretion through cAMP-dependent protein kinase A.3,48 In the other category are acetylcholine, GRP, substance P, and CCK. The actions of these agonists include stimulating cellular metabolism of membrane phosphoinositides and raising intracellular free calcium concentrations ([Ca2+]i) from mobilization of intracellular stores.3,48 Specifically, the agonist-receptor interaction leads to a phospholipase C-mediated hydrolysis of phosphatidylinositol 4,5-bisphosphate to 1,2-diacylglycerol and inositol 1,4,5-triphosphate (IP3). IP3, in turn, releases calcium from endoplasmic reticulum stores.51 The calcium release into the cytosol causes a rapid rise in the concentration of free calcium that is necessary for the secretory response.52 Calcium release into the cytosol is also mediated by ryanodine receptors and signals interacting with the ryanodine receptor, such as calcium and fatty acid-coenzyme A (CoA) esters.53 Other molecules involved in intracellular calcium release are cyclic adenosine diphosphate (ADP)–ribose and nicotinic acid adenine dinucleotide phosphate (NAADP).54
The mechanism by which increases in [Ca2+]i mediate secretion is not established but involves calmodulin-dependent protein kinases and actin-myosin interactions, SNARE proteins, and GTP-binding proteins as discussed earlier.3 The continued stimulation of enzyme secretion by these agents also depends on the influx of extracellular calcium.52 This influx is mediated by changes in nitric oxide and cyclic guanosine monophosphate (cGMP). Components of the influx channel have been determined.55
The intracellular mechanism of enzyme secretion may also be regulated by 1,2-diacylglycerol and protein kinase C3,56 as well as arachidonic acid.57 Specific phosphorylations and dephosphorylations of cellular proteins also occur with cAMP agonists and calcium-phosphoinositide agonists.3 The exact roles of these events in secretion are not established.
ORGAN PHYSIOLOGY
INTERDIGESTIVE SECRETION
The interdigestive pancreatic secretory pattern is cyclic and follows the pattern of the migrating myoelectric complex (MMC).58 The patterns recur every 60 to 120 minutes, bursts of enzyme secretion being temporally associated with the periods of increased motor activity in the stomach and duodenum (i.e., phases II and III). In addition to pancreatic enzyme secretion is increased secretion of bicarbonate and bile (secondary to partial gallbladder contraction) into the duodenum during phases II and III of the MMC (see Chapter 97). The underlying mechanism involves the cholinergic nervous system.59 Motilin and pancreatic polypeptide are also involved in regulation of the MMC.60,61 The pancreatic secretion during the interdigestive phase integral to the “housekeeping” function of the MMC.
DIGESTIVE SECRETION
The vagal nerves mediate the cephalic phase of the exocrine secretion. The extent of cephalic stimulation of exocrine pancreatic secretion in humans has been evaluated through measurement of exocrine secretions stimulated by sham feeding (chewing and spitting out the food). One study indicated that sham feeding stimulated pancreatic enzyme secretion at up to 50% of the maximal secretory rate, with no increase in bicarbonate secretion when gastric secretions were prevented from entering the duodenum.62 When gastric secretions were allowed entry into the duodenum, the rate of pancreatic enzyme secretion rose to about 90% of maximal, and bicarbonate was also secreted. These results suggest that cephalic stimulation specifically stimulates acinar secretion and that a low pH in the duodenum (from gastric acid) augments this secretion as well as causes bicarbonate secretion.
Results of investigations of the mechanism of neurotransmission during cephalic stimulation are controversial. Acetylcholine is certainly a major neurotransmitter involved because cholinergic antagonists greatly reduce and in some cases abolish sham feeding–stimulated pancreatic secretion in humans.63 Nerve endings containing the peptides VIP, GRP, CCK, and enkephalins have been identified in the pancreas. Data supporting the role of these peptides in the cephalic phase of secretion are strongest for VIP and GRP.64,65 Both are released into the pancreatic venous effluent with vagal stimulation in animals. Furthermore, as discussed, acinar cells have receptors for GRP and VIP that mediate enzyme secretion. The ductal epithelium also responds to VIP with the secretion of water and bicarbonate.64
The gastric phase of pancreatic secretion results from meal stimuli acting in the stomach. The major stimulus is gastric distention, which causes predominantly secretion of enzymes with little secretion of water and bicarbonate. Balloon distention of either the gastric fundus or the antrum results in a low-volume, enzyme-rich secretion by way of a gastropancreatic vagovagal reflex.66
The major mediator of hydrogen ion–stimulated bicarbonate and water secretion is secretin. Secretin is released from the duodenal mucosa by gastric acid, with a pH threshold of 4.5.67 The quantity of secretin released as well as the volume of pancreatic secretion depend on the load of titratable acid delivered to the duodenum.67,68 Immunoneutralization of secretin with specific antisecretin antibody decreases meal-stimulated pancreatic volume and bicarbonate secretion by as much as 80%.69 The antisecretin antibody also inhibits meal-stimulated enzyme secretion by as much as 50%, suggesting that secretin also has a role in enzyme secretion, possibly by potentiating the action of agonists such as acetylcholine.
If exogenous secretin is infused to reproduce the plasma concentrations of secretin during a meal, the pancreatic bicarbonate output is less than the bicarbonate output observed with a meal. The bicarbonate response to secretin also depends on cholinergic input because atropine partially inhibits the response stimulated by exogenous secretin.70 Thus, the complete meal-stimulated response results from a combination of mediators.
During the intestinal phase, secretion of digestive enzymes is mediated by intraluminal fatty acids more than eight carbons in length, monoglycerides of these fatty acids, peptides, amino acids, and, to a small extent, glucose. The most potent amino acids for stimulating secretion in humans are phenylalanine, valine, methionine, and tryptophan.71 The response to peptides and amino acids is related to the total load perfused into the intestine rather than the concentration.72
The mediators of the enzyme secretory response from intestinal stimuli are neural and humoral (Fig. 56-7). Truncal vagotomy and atropine markedly inhibit the enzyme (and bicarbonate) responses to low intestinal loads of amino acids and fatty acids as well as infusion of physiologic concentrations of CCK.73–75 These results indicate that vagovagal reflexes mediate enzyme secretion and augment bicarbonate secretion stimulated by secretin.
CCK is the major humoral mediator of meal-stimulated enzyme secretion during the intestinal phase. The circulating concentration of CCK is found to rise with a meal76 and the main circulating form is 58 amino acids in size (CCK-58).77 CCK is released from the upper small intestinal mucosa by digestion products of fat and protein and, to a lesser extent, by starch digestion products.76
Further experiments indicate that CCK activates afferent neurons in the duodenal mucosa.74,75 These afferent neurons activate a vagovagal reflex that causes pancreatic enzyme secretion, as illustrated in Figure 56-7.
The elements of the secretory mechanism during the intestinal phase of a meal previously described are activated when standard nutrients enter the duodenum. Investigations have been performed to determine whether administration of alternative nutrients or delivery of nutrients to the jejunum rather than the duodenum may result in less activation of the pancreatic secretory response. Such information is critical for strategies to provide nutrients to patients with pancreatic disorders such as acute or chronic pancreatitis. With acute pancreatitis, stimulation of the pancreas can exacerbate the severity of the disease; in chronic pancreatitis, stimulation of the pancreas can exacerbate pain (see Chapter 58 and 59).
The research in this area has delineated effects of different types of nutrients as well as location of administration on the pancreatic secretory response. Studies in normal human volunteers compared the effect of equicaloric amounts of long-chain triglycerides and medium-chain triglycerides, infused into the jejunum, on plasma CCK levels, pancreatic secretion, and gallbladder contraction.78 Medium-chain triglycerides led to none of these responses, but long-chain triglycerides stimulated each of the responses. Thus, with respect to jejunal administration, medium-chain triglycerides can be used to provide an energy source without significantly stimulating the pancreas.
Another study in healthy volunteers compared the effects of duodenal infusions of a complex liquid diet with those of an elemental diet with low fat content.79 Noteworthy was the finding of significantly less pancreatic secretion with the elemental diet than with the complex liquid diet. In the clinical setting, the results of these studies indicate that the type of nutrient administered to the intestinal lumen is the most important factor in regulating the pancreatic secretory response.
FEEDBACK REGULATION
In animals as well as humans, diversion of pancreatic juice from the intestine results in augmented pancreatic secretion.80 The augmented enzyme secretion is mediated by a rise in circulating CCK.81 Both the increase in CCK and enzyme secretion following diversion of pancreatic juice can be inhibited by replenishment of intraluminal trypsin and other digestive enzymes.82 It is hypothesized that during a meal, when trypsin is occupied by meal proteins, pancreatic secretion is enhanced because trypsin is not available to the surface of the intestinal epithelial cells to cause feedback inhibition. After the meal, trypsin is free and inhibits intestinal CCK release and pancreatic enzyme secretion. Intraluminal CCK-releasing factors that mediate this effect of trypsin have been described. One is a protein, called monitor peptide, that is secreted by the pancreas.83 Another is called luminal CCK-releasing factor (LCRF).84 Both monitor peptide, and LCRF causes CCK release from the enteroendocrine CCK cell (I cell) into the blood. These releasing factors are likely mediators of the physiologic feedback mechanism for enzyme secretion. It is believed that the effects of trypsin described earlier are due to degradation of the CCK-releasing factors by trypsin when trypsin is not bound to meal proteins.
A similar feedback mechanism involving a secretin-releasing factor (an intraluminal peptide) has been described that regulates release of secretin from the secretin-containing enteroendocrine cell of the intestine.85,86
PANCREATIC SECRETORY FUNCTION TESTS
Various tests have been devised to measure the secretory function of the pancreas in order to diagnose disorders such as chronic pancreatitis and pancreatic cancer (Table 56-2).87–100 The function tests fall into two general categories: direct and indirect. Direct tests of pancreatic secretory function involve collection of pancreatic secretions after intravenous administration of a secretagogue or a combination of secretagogues. Indirect tests of pancreatic secretory function include the measurement of pancreatic enzymes in duodenal samples after nutrient ingestion; the measurement of products of digestive enzyme action on ingested substrates; the measurement of pancreatic enzymes in the stool; and the measurement of the plasma concentration of hormones or other markers that are altered in pancreatic insufficiency states.
Which pancreatic function test should be used depends on the clinical question and the characteristics and availability of the test. The exocrine pancreas has a very large functional reserve. Maldigestion and malabsorption do not occur until the functional capacity as measured by CCK-stimulated digestive enzyme secretion is reduced to 5% to 10% of normal.71,87 Thus, many tests relying on the conversion of an ingested substrate by digestive enzymes to a measurable product will be insensitive in pancreatic disease unless moderate to severe pancreatic insufficiency is present. Therefore, the measurement of duodenal digestive enzymes after the intravenous administration of pancreatic secretagogues provides the greatest sensitivity and specificity. The major drawbacks to the direct tests are the requirements for duodenal intubation and the fact that very few centers are proficient in performing the studies properly. Improved imaging techniques for diagnosing pancreatic disease have greatly decreased the use of the tests. On certain occasions, however, pancreatic function tests are necessary for diagnosing pancreatic disease. More recently, a direct test using endoscopy and rapid collection has been described.87,92–95 The pancreatic function tests are described here to allow the reader to appreciate the relative diagnostic usefulness of each test.
DIRECT TESTS
Direct tests provide a gold standard for measurement of pancreatic function. Stimulation of secretion has been described most commonly with secretin, CCK, or the two combined. The combination provides the complete information about acinar and ductular cell secretions. In fact, it is accepted that the direct tests are more sensitive than current imaging techniques in the diagnosis of early pancreatic disease.87 In the classic description, the stomach and duodenum are intubated. The gastric intubation is required to remove gastric secretions that would interfere with the ability to measure water and bicarbonate secretion from the pancreas. Low pH may also alter pancreatic enzyme activity. The duodenal tube is used for infusion of a nonabsorbable marker and collection of pancreatic secretions. The use of a nonabsorbable marker such as cobalamin or polyethylene glycol (PEG) allows the quantitation of secretions without the need for complete aspiration of secretions.88
The direct function tests are based on the principle that maximal water, bicarbonate, and enzyme secretion are related to the functional mass of the pancreas.89 Historically, the secretin test (intravenous administration of secretin, with volume and bicarbonate measurement) first provided information about the function of the pancreas in various clinical settings. Administration of CCK and the measurement of digestive enzyme secretion also have been used successfully to demonstrate pancreatic insufficiency. Because the combination of secretin and CCK administration provides stimulation of both functional units of the exocrine pancreas, this combination is most commonly used.88–91 CCK is best delivered by constant intravenous infusion. The dose for synthetic CCK-octapeptide (carboxy-terminal octapeptide of CCK; Squibb, Princeton, NJ) is 40 ng/kg/hr. The dose of synthetic secretin (SecroFlo, Repligen, Needham, Mass) is 0.2 µg/kg injected over 1 minute. Measurements, corrected for percentage recovery of a nonabsorbable marker, are made for fluid and for bicarbonate and protein concentrations, and the activity of digestive enzymes during collections over 15-minute periods for 1 hour. Amylase, trypsin, chymotrypsin, and lipase are the digestive enzymes most commonly measured.88,90
In a study of 236 subjects undergoing continuous infusion of CCK-octapeptide (40 ng/kg/hr) and purified natural secretin (0.25 clinical unit [CU]/kg/hr), measurements of chymotrypsin concentration and peak bicarbonate output were used to distinguish subjects with chronic pancreatitis from those without organic disease.91 The test was 83% sensitive and 89% specific. False-positive results may occur in patients with celiac disease and diabetes mellitus.
An adaptation of the direct secretory test to upper endoscopy also has been described.92–95 At the time of endoscopy, either secretin, CCK, or the combination is administered intravenously, and pancreatic secretions are collected via the endoscope positioned in the duodenum.
INDIRECT TESTS
Lundh Test Meal96
The subject ingests a 300-mL liquid test meal composed of dried milk, vegetable oil, and dextrose (6% fat, 5% protein, and 15% carbohydrate). After ingestion of this meal, samples are aspirated from the intubated duodenum at intervals for measurement of digestive enzyme concentration. Usually only trypsin activity is measured; however, the additional determination of lipase or amylase may improve test sensitivity. The test is not valid in patients with mucosal disease (e.g., celiac disease) or altered gastroduodenal anatomy (e.g., following a vagotomy and drainage procedure or a Billroth II gastrectomy). Comparisons of the Lundh test meal with the secretin-CCK test show that the latter is more sensitive in detecting mild forms of pancreatic disease, whereas the tests are comparable in more advanced disease.88
Measurement of Fecal Fat
Steatorrhea occurs when stimulated lipase output drops to less than 5% to 10% of normal.71 Thus, measurement of fat in the stools collected for 72 hours in a subject ingesting a diet adequate in fat intake (70 to 100 g/day) is considered an effective means of diagnosing steatorrhea. Normally 7% or less of ingested fat appears in the stool. A simple qualitative microscopic examination of a single stool for oil is almost as sensitive as quantitative measurements for fat.88 Because steatorrhea occurs only with advanced pancreatic disease, measurement of fecal fat is not useful in the diagnosis of mild or moderate disease, nor is steatorrhea specific for pancreatic disease (see Chapter 101).
Measurement of Fecal Chymotrypsin and Elastase 1
Chymotrypsin measurements in the stool have been used as indirect tests of pancreatic function for many years,97 especially to establish pancreatic insufficiency in patients with cystic fibrosis.88 These measurements are about 85% sensitive in advanced pancreatic dysfunction and relatively insensitive in mild to moderate disease.
Pancreatic elastase 1 can be measured in the stool using a monoclonal antibody against human elastase 1. This test, like other indirect tests, is not sensitive in detecting mild to moderate pancreatic disease.98,99
Other Tests
The bentiromide test is an indirect tubeless test in which the synthetic peptide N-benzoyl-l-tyrosyl-p-aminobenzoic acid (NBT-PABA) is specifically cleaved by chymotrypsin to NBT (N-benzoyl-l-tyrosyl) and para-aminobenzoic acid (PABA). PABA is then absorbed in the intestine, conjugated in the liver, and excreted in the urine.88 The PABA metabolite can be measured in either serum or urine. Prior gastric surgery, small bowel disease, liver disease, and renal insufficiency may interfere with the measurements. So may the use of several drugs (acetaminophen, phenacetin, chloramphenicol, benzocaine, lidocaine, procaine, sulfonamides, sulfonylureas, and thiazides) and prior ingestion of certain foods (prunes and cranberries).
A broad range of sensitivities has been reported for the NBT-PABA test.88 In patients with severe pancreatic insufficiency and malabsorption, the sensitivity is 80% to 90%. In those with mild to moderate impairment, test sensitivity is as low as 40%. In order to improve test specificity, several modifications of the NBT-PABA test have been devised. Administering free PABA on a separate day or giving carbon-14 (14C)–labeled PABA simultaneously with NBT-PABA may identify patients in whom abnormal NBT-PABA test results are caused by mucosal disease of the small bowel.88
Another test similar to the NBT-PABA test is the fluorescein dilaurate (pancreolauryl) test. The principle underlying this test is the same as that for the NBT-PABA test. Fluorescein dilaurate is an ester, poorly soluble in water, that is hydrolyzed by pancreatic carboxylesterase into lauric acid and free water-soluble fluorescein. The fluorescein is readily absorbed into the intestine, partly conjugated in the liver, and excreted in the urine. Fluorescein dilaurate is given in the middle of a breakfast meal.88 Urine is collected for 10 hours after breakfast, and the fluorescein excreted in the urine is measured. Like the PABA metabolite, fluorescein can also be measured in the serum. To evaluate the subject’s absorption, conjugation, and excretion, the test is repeated two to three days later with free fluorescein. The recovery rate on both days is expressed as a ratio. Like the NBT-PABA test, the pancreolauryl test is highly sensitive and specific for advanced pancreatic disease and less so for mild or moderate disease.
Several other indirect tubeless tests have been created in an effort to improve sensitivity for identifying milder forms of exocrine pancreatic dysfunction. They include triglyceride and cholesteryl breath tests; H2 and CO2 breath tests; the dual-label Schilling test; and plasma measurements of pancreatic polypeptide and amino acids.88,100 However, none of these tests has been shown to have greater sensitivity than the indirect tubeless tests described previously. In addition, many of them require radioactive isotopes or expensive equipment, making their usefulness less desirable.
O’Keefe SJ, Lee RB, Anderson FP, et al. Physiological effects of enteral and parenteral feeding on pancreaticobiliary secretion in humans. Am J Physiol Gastrointest Liver Physiol. 2003;284:G27-36. (Ref 79.)
Petersen OH, Tepikin AV. Polarized calcium signaling in exocrine gland cells. Annu Rev Physiol. 2008;70:273-99. (Ref 54.)
Stevens T, Conwell DL, Zuccaro GJr, et al. A prospective crossover study comparing secretin-stimulated endoscopic and Dreiling tube pancreatic function testing in patients evaluated for chronic pancreatitis. Gastrointest Endosc. 2008;67:458-66. (Ref 94.)
Steward MC, Ishiguro H, Case RM. Mechanisms of bicarbonate secretion in the pancreatic duct. Annu Rev Physiol. 2005;67:377-409. (Ref 10.)
Whitcomb DC, Gorry MC, Preston RA, et al. Hereditary pancreatitis is caused by a mutation in the cationic trypsinogen gene. Nat Genet. 1996;14:141-5. (Ref 19.)
Whitcomb DC, Lowe ME. Human pancreatic digestive enzymes. Dig Dis Sci. 2007;52:1-17. (Ref 17.)
Williams JA. Intracellular signaling mechanisms activated by cholecystokinin-regulating synthesis and secretion of digestive enzymes in pancreatic acinar cells. Annu Rev Physiol. 2001;63:77-97. (Ref 3.)
1. Bayliss WM, Starling EH. The mechanisms of pancreatic secretion. J Physiol. 1902;28:325-53.
2. Palade G. Intracellular aspects of the process of protein synthesis. Science. 1975;189:347-58.
3. Williams JA. Intracellular signaling mechanisms activated by cholecystokinin-regulating synthesis and secretion of digestive enzymes in pancreatic acinar cells. Annu Rev Physiol. 2001;63:77-97.
4. Motta PM, Macchiarelli G, Nottola SA, Correr S. Histology of the exocrine pancreas. Microsc Res Tech. 1997;37:384-98.
5. O’Konski MS, Pandol SJ. Effects of caerulein on the apical cytoskeleton of the pancreatic acinar cell. J Clin Invest. 1990;86:1649-57.
6. Muallem S, Kwiatkowska K, Xu X, Yin HL. Actin filament disassembly is a sufficient final trigger for exocytosis in nonexcitable cells. J Cell Biol. 1995;128:589-98.
7. Fallon MB, Gorelick FS, Anderson JM, et al. Effect of cerulein hyperstimulation on the paracellular barrier of rat exocrine pancreas. Gastroenterology. 1995;108:1863-72.
8. Stauffer PL, Zhao H, Luby-Phelps K, et al. Gap junction communication modulates [Ca2+]i oscillations and enzyme secretion in pancreatic acini. J Biol Chem. 1993;268:19769-75.
9. Yule DI, Stuenkel E, Williams JA. Intercellular calcium waves in rat pancreatic acini: Mechanism of transmission. Am J Physiol. 1996;271:C1285-94.
10. Steward MC, Ishiguro H, Case RM. Mechanisms of bicarbonate secretion in the pancreatic duct. Annu Rev Physiol. 2005;67:377-409.
11. Sohma Y, Gray MA, Imai Y, Argent BE. HCO3-transport in a mathematical model of the pancreatic ductal epithelium. J Membr Biol. 2000;176:77-100.
12. Whitcomb DC, Ermentrout GB. A mathematical model of the pancreatic duct cell generating high bicarbonate concentrations in pancreatic juice. Pancreas. 2004;29:e30-40.
13. Marino CR, Matovcik LM, Gorelick FS, Cohn JA. Localization of the cystic fibrosis transmembrane conductance regulator in pancreas. J Clin Invest. 1991;88:712-16.
14. Stuenkel EL, Machen TE, Williams JA. pH regulatory mechanisms in rat pancreatic ductal cells. Am J Physiol. 1988;254:G925-30.
15. Scheele G, Bartelt D, Bieger W. Characterization of human exocrine pancreatic proteins by two-dimensional isoelectric focusing/sodium dodecyl sulfate gel electrophoresis. Gastroenterology. 1981;80:461-73.
16. Rinderknecht H. Pancreatic secretory enzymes. In: Go V, Gardner J, DiMango E, et al, editors. The exocrine pancreas: Biology, pathobiology and disease. 2nd ed. New York: Raven; 1993:219-251.
17. Whitcomb DC, Lowe ME. Human pancreatic digestive enzymes. Dig Dis Sci. 2007;52:1-17.
18. Pubols MH, Bartelt DC, Greene LJ. Trypsin inhibitor from human pancreas and pancreatic juice. J Biol Chem. 1974;249:2235-42.
19. Whitcomb DC, Gorry MC, Preston RA, et al. Hereditary pancreatitis is caused by a mutation in the cationic trypsinogen gene. Nat Genet. 1996;14:141-5.
20. Meites S, Rogols S. Amylase isoenzymes. CRC Crit Rev Clin Lab Sci. 1971;2:103-38.
21. Kimmich GA. Membrane potentials and the mechanism of intestinal Na(+)-dependent sugar transport. J Membr Biol. 1990;114:1-27.
22. Wright EM, Martín MG, Turk E. Intestinal absorption in health and disease-sugars. Best Pract Res Clin Gastroenterol. 2003;17:943-56.
23. Hofmann AF, Borgstrom B. Hydrolysis of long-chain monoglycerides in micellar solution by pancreatic lipase. Biochim Biophys Acta. 1963;70:317-31.
24. Tsujita T, Okuda H. Effect of bile salts on the interfacial inactivation of pancreatic carboxylester lipase. J Lipid Res. 1990;31:831-8.
25. Kilberg MS, Stevens BR, Novak DA. Recent advances in mammalian amino acid transport. Annu Rev Nutr. 1993;13:137-65.
26. Nicchitta CV. A platform for compartmentalized protein synthesis: Protein translation and translocation in the ER. Curr Opin Cell Biol. 2002;14:412-16.
27. Farquhar MG, Palade GE. The Golgi apparatus: 100 years of progress and controversy. Trends Cell Biol. 1998;8:2-10.
28. Kubisch CH, Sans MD, Arumugam T, et al. Early activation of endoplasmic reticulum stress is associated with arginine-induced acute pancreatitis. Am J Physiol Gastrointest Liver Physiol. 2006;291:G238-45.
29. Hille-Rehfeld A. Mannose 6-phosphate receptors in sorting and transport of lysosomal enzymes. Biochim Biophys Acta. 1995;1241:177-94.
30. Nemoto T, Kojima T, Oshima A, et al. Stabilization of exocytosis by dynamic F-actin coating of zymogen granules in pancreatic acini. J Biol Chem. 2004;279:37544-50.
31. Poucell-Hatton S, Perkins PS, Deerinck TJ, et al. Myosin I is associated with zymogen granule membranes in the rat pancreatic acinar cell. Gastroenterology. 1997;113:649-58.
32. Valentijn JA, Valentijn K, Pastore LM, Jamieson JD. Actin coating of secretory granules during regulated exocytosis correlates with the release of rab3D. Proc Natl Acad Sci U S A. 2000;97:1091-5.
33. Hansen NJ, Antonin W, Edwardson JM. Identification of SNAREs involved in regulated exocytosis in the pancreatic acinar cell. J Biol Chem. 1999;274:22871-6.
34. Ohnishi H, Mine T, Shibata H, et al. Involvement of Rab4 in regulated exocytosis of rat pancreatic acini. Gastroenterology. 1999;116:943-52.
35. Chen X, Li C, Izumi T, Ernst SA, et al. Rab27b localizes to zymogen granules and regulates pancreatic acinar exocytosis. Biochem Biophys Res Commun. 2004;323:1157-62.
36. Le Page SL, Bi Y, Williams JA. CCK-A receptor activates RhoA through G alpha 12/13 in NIH3T3 cells. Am J Physiol Cell Physiol. 2003;285:C1197-206.
37. Gaisano HY, Lutz MP, Leser J, et al. Supramaximal cholecystokinin displaces Munc18c from the pancreatic acinar basal surface, redirecting apical exocytosis to the basal membrane. J Clin Invest. 2001;108:1597-611.
38. Cosen-Binker LI, Binker MG, Wang CC, et al. VAMP8 is the v-SNARE that mediates basolateral exocytosis in a mouse model of alcoholic pancreatitis. J Clin Invest. 2008;118:2535-51.
39. Boulet AM, Erwin CR, Rutter WJ. Cell-specific enhancers in the rat exocrine pancreas. Proc Natl Acad Sci U S A. 1986;83:3599-603.
40. Stevenson BJ, Hagenbuchle O, Wellauer PK. Sequence organisation and transcriptional regulation of the mouse elastase II and trypsin genes. Nucleic Acids Res. 1986;14:8307-30.
41. Cockell M, Stevenson BJ, Strubin M, et al. Identification of a cell-specific DNA-binding activity that interacts with a transcriptional activator of genes expressed in the acinar pancreas. Mol Cell Biol. 1989;9:2464-75.
42. Howard G, Keller PR, Johnson TM, Meisler MH. Binding of a pancreatic nuclear protein is correlated with amylase enhancer activity. Nucleic Acids Res. 1989;17:8185-95.
43. Rose SD, Kruse F, Swift GH, et al. A single element of the elastase I enhancer is sufficient to direct transcription selectively to the pancreas and gut. Mol Cell Biol. 1994;14:2048-57.
44. Kruse F, Rose SD, Swift GH, et al. Cooperation between elements of an organ-specific transcriptional enhancer in animals. Mol Cell Biol. 1995;15:4385-94.
45. Brannon PM. Adaptation of the exocrine pancreas to diet. Annu Rev Nutr. 1990;10:85-105.
46. Birk RZ, Brannon PM. Regulation of pancreatic lipase by dietary medium chain triglycerides in the weanling rat. Pediatr Res. 2004;55:921-6.
47. Perkins PS, Rutherford RE, Pandol SJ. Effect of chronic ethanol feeding on digestive enzyme synthesis and mRNA content in rat pancreas. Pancreas. 1995;10:14-21.
48. Williams JA. Regulation of pancreatic acinar cell function. Curr Opin Gastroenterol. 2006;22:498-504.
49. Wank SA, Harkins R, Jensen RT, et al. Purification, molecular cloning, and functional expression of the cholecystokinin receptor from rat pancreas. Proc Natl Acad Sci U S A. 1992;89:3125-9.
50. Murphy JA, Criddle DN, Sherwood M, et al. Direct activation of cytosolic Ca(2+) signaling and enzyme secretion by cholecystokinin in human pancreatic acinar cells. Gastroenterology. 2008;135:632-41.
51. Muallem S, Schoeffield M, Pandol S, Sachs G. Inositol triphosphate modification of ion transport in rough endoplasmic reticulum. Proc Natl Acad Sci U S A. 1985;82:4433-7.
52. Pandol SJ, Schoeffield MS, Sachs G, Muallem S. Role of free cytosolic calcium in secretagogue-stimulated amylase release from dispersed acini from guinea pig pancreas. J Biol Chem. 1985;260:10081-6.
53. Fitzsimmons TJ, McRoberts JA, Tachiki KH, Pandol SJ. Acyl-coenzyme A causes Ca2+ release in pancreatic acinar cells. J Biol Chem. 1997;272:31435-40.
54. Petersen OH, Tepikin AV. Polarized calcium signaling in exocrine gland cells. Annu Rev Physiol. 2008;70:273-99.
55. Sutton R, Petersen OH, Pandol SJ. Pancreatitis and calcium signaling: Report of an international workshop. Pancreas. 2008;36:e1-14.
56. Pandol SJ, Schoeffield MS. 1,2-Diacylglycerol, protein kinase C, and pancreatic enzyme secretion. J Biol Chem. 1986;261:4438-44.
57. Pandol SJ, Hsu YL, Kondratenko NF, et al. Dual pathways for agonist-stimulated arachidonic acid release in pancreatic acini: Roles in secretion. Am J Physiol. 1991;260:G423-33.
58. DiMagno EP, Hendricks JC, Go VL, Dozois RR. Relationships among canine fasting pancreatic and biliary secretions, pancreatic duct pressure, and duodenal phase III motor activity: Boldyreff revisited. Dig Dis Sci. 1979;24:689-93.
59. Zimmerman DW, Sarr MG, Smith CD, et al. Cyclic interdigestive pancreatic exocrine secretion: Is it mediated by neural or hormonal mechanisms? Gastroenterology. 1992;102:1378-84.
60. Itoh Z. Motilin and clinical application. Peptides. 1997;18:593-608.
61. Fox JA. Control of gastrointestinal motility by peptides: Old peptides, new tricks-new peptides, old tricks. Gastroenterol Clin North Am. 1989;18:163-77.
62. Anagnostides A, Chadwick VS, Selden AC, Maton PN. Sham feeding and pancreatic secretion: Evidence for direct vagal stimulation of enzyme output. Gastroenterology. 1984;87:109-14.
63. Katschinski M, Dahmen G, Reinshagen M, et al. Cephalic stimulation of gastrointestinal secretory and motor responses in humans. Gastroenterology. 1993;103:383-91.
64. Holst JJ, Schaffalitzky de Muckadell OB, Fahrenkrug J. Nervous control of pancreatic exocrine secretion in pigs. Acta Physiol Scand. 1979;105:33-40.
65. Holst JJ, Knuhtsen S, Skak-Nielsen T. The role of gastrin-releasing peptide in pancreatic exocrine secretion. Ann N Y Acad Sci. 1988;547:234-41.
66. Kreiss C, Schwizer W, Erlacher U, et al. Role of antrum in regulation of pancreaticobiliary secretion in humans. Am J Physiol. 1996;270:G844-51.
67. Chey WY, Konturek SJ. Plasma secretion and pancreatic secretion in response to liver extract meal with varied pH and exogenous secretin in the dog. J Physiol. 1982;324:263-72.
68. Meyer JH, Way LW, Grossman MI. Pancreatic bicarbonate response to various acids in duodenum of the dog. Am J Physiol. 1970;219:964-70.
69. Chey WY, Kim MS, Lee KY, Chang TM. Effect of rabbit antisecretin serum on postprandial pancreatic secretion in dogs. Gastroenterology. 1979;77:1268-75.
70. You CH, Rominger JM, Chey WY. Effects of atropine on the action and release of secretin in humans. Am J Physiol. 1982;242:G608-11.
71. Go VL, Hofmann AF, Summerskill WH. Pancreozymin bioassay in man based on pancreatic enzyme secretion: Potency of specific amino acids and other digestive products. J Clin Invest. 1970;49:1558-64.
72. Meyer JH, Kelly GA, Spingola LJ, Jones RS. Canine gut receptors mediating pancreatic responses to luminal L-amino acids. Am J Physiol. 1976;231:669-77.
73. Singer MV, Solomon TE, Grossman MI. Effect of atropine on secretion from intact and transplanted pancreas in dog. Am J Physiol. 1980;238:G18-22.
74. Owyang C. Physiological mechanisms of cholecystokinin action on pancreatic secretion. Am J Physiol. 1996;271:G1-7.
75. Li Y, Hao Y, Owyang C. High-affinity CCK-A receptors on the vagus nerve mediate CCK-stimulated pancreatic secretion in rats. Am J Physiol. 1997;273:G679-85.
76. Liddle RA, Goldfine ID, Rosen MS, et al. Cholecystokinin bioactivity in human plasma: Molecular forms, responses to feeding, and relationship to gallbladder contraction. J Clin Invest. 1985;75:1144-52.
77. Reeve JRJr, Green GM, Chew P, et al. CCK-58 is the only detectable endocrine form of cholecystokinin in rat. Am J Physiol Gastrointest Liver Physiol. 2003;285:G255-65.
78. Symersky T, Vu MK, Frolich M, et al. The effect of equicaloric medium-chain and long-chain triglycerides on pancreas enzyme secretion. Clin Physiol Funct Imaging. 2002;22:307-11.
79. O’Keefe SJ, Lee RB, Anderson FP, et al. Physiological effects of enteral and parenteral feeding on pancreaticobiliary secretion in humans. Am J Physiol Gastrointest Liver Physiol. 2003;284:G27-36.
80. Green GM, Lyman RL. Feedback regulation of pancreatic enzyme secretion as a mechanism for trypsin inhibitor-induced hypersecretion in rats. Proc Soc Exp Biol Med. 1972;140:6-12.
81. Louie DS, May D, Miller P, Owyang C. Cholecystokinin mediates feedback regulation of pancreatic enzyme secretion in rats. Am J Physiol. 1986;250:G252-9.
82. Walkowiak J, Witmanowski H, Strzykala K, et al. Inhibition of endogenous pancreatic enzyme secretion by oral pancreatic enzyme treatment. Eur J Clin Invest. 2003;33:65-9.
83. Liddle RA. Regulation of cholecystokinin secretion by intraluminal releasing factors. Am J Physiol. 1995;269:G319-27.
84. Spannagel AW, Green GM, Guan D, et al. Purification and characterization of a luminal cholecystokinin-releasing factor from rat intestinal secretion. Proc Natl Acad Sci U S A. 1996;93:4415-20.
85. Li JP, Chang TM, Wagner D, Chey WY. Pancreatic phospholipase A2 from the small intestine is a secretin-releasing factor in rats. Am J Physiol Gastrointest Liver Physiol. 2001;281:G526-32.
86. Li P, Chang TM, Chey WY. Neuronal regulation of the release and action of secretin-releasing peptide and secretin. Am J Physiol. 1995;269:G305-12.
87. DiMagno M, DiMagno E. Chronic pancreatitis. Current Opin Gastroenterol. 2006;22:487-97.
88. Niederau C, Grendell JH. Diagnosis of chronic pancreatitis. Gastroenterology. 1985;88:1973-95.
89. Hansky J, Tiscornia OM, Dreiling DA, Janowitz HD. Relationship between maximal secretory output and weight of the pancreas in the dog. Proc Soc Exp Biol Med. 1963;114:654-6.
90. Boyd EJ, Wormsley KG. Laboratory tests in the diagnosis of the chronic pancreatic diseases. Part 1: Secretagogues used in tests of pancreatic secretion. Int J Pancreatol. 1987;2:137-48.
91. Heiji HA, Obertop H, Schmitz PIM, et al. Evaluation of the secretin-cholecystokinin test for chronic pancreatitis by discriminant analysis. Scand J Gastroenterol. 1986;21:35-40.
92. Raimondo M, Imoto M, DiMagno EP. Rapid endoscopic secretin stimulation test and discrimination of chronic pancreatitis and pancreatic cancer from disease controls. Clin Gastroenterol Hepatol. 2003;1:397-403.
93. Conwell DL, Zuccaro G, Morrow JB, et al. Analysis of duodenal drainage fluid after cholecystokinin (CCK) stimulation in healthy volunteers. Pancreas. 2002;25:350-4.
94. Stevens T, Conwell DL, Zuccaro GJr, et al. A prospective crossover study comparing secretin-stimulated endoscopic and Dreiling tube pancreatic function testing in patients evaluated for chronic pancreatitis. Gastrointest Endosc. 2008;67:458-66.
95. Conwell DL, Zuccaro GJr, Vargo JJ, et al. An endoscopic pancreatic function test with synthetic porcine secretin for the evaluation of chronic abdominal pain and suspected chronic pancreatitis. Gastrointest Endosc. 2003;57:37-40.
96. Lundh G. Pancreatic exocrine function in neoplastic and inflammatory disease: A simple and reliable new test. Gastroenterology. 1962;42:275-80.
97. Lankisch PG. Function tests in the diagnosis of chronic pancreatitis: Critical evaluation. Int J Pancreatol. 1993;14:9-20.
98. Hardt PD, Marzeion AM, Schnell-Kretschmer H, et al. Fecal elastase 1 measurement compared with endoscopic retrograde cholangiopancreatography for the diagnosis of chronic pancreatitis. Pancreas. 2002;25:e6-9.
99. Lankisch PG. Now that fecal elastase is available in the United States, should clinicians start using it? Curr Gastroenterol Rep. 2004;6:126-31.
100. Newcomer AD, Hofmann AF, DiMagno EP, et al. Triolein breath test: A sensitive and specific test for fat malabsorption. Gastroenterology. 1979;76:6-13.