Chapter 32 Wilderness Cardiology
This chapter reviews basic cardiovascular physiologic adaptations to the wilderness environment. Although basic cardiovascular responses to exercise are central to all wilderness activities, a detailed discussion of exercise physiology is beyond the scope of this chapter; the reader is referred elsewhere, including Chapter 98, for this information.30 In addition, we review cardiovascular conditions of relevance to wilderness travelers and physicians, with emphasis on basic management of common cardiovascular emergencies in the wilderness setting.
Cardiovascular System Responses to Specific Wilderness Environments
Hyperbaria (Underwater Diving)
Diving places a unique combination of physical stressors on the cardiovascular system. The physiologic stress of diving arises from the combination of immersion underwater and sustained moderate-intensity exercise. Immersion significantly reduces the effect of gravity on the diver’s body and therefore on the lower extremity venous blood pool when the diver is upright.19 This results in central redistribution of blood, with increases in intrathoracic blood volume and right atrial and ventricular filling pressures.20 The direction and magnitude of the fluid shift induced by water immersion depend on the position of the diver in the water. If the diver is upright, then the extensively researched physiology of upright water immersion applies. However, if the diver is horizontal or head down, entirely different hydrostatic gradients are induced, and these may be dynamic as the diver changes position in the water. For upright divers, as dictated by the Frank-Starling phenomenon, the increase in cardiac preload leads to immediate increases in stroke volume, cardiac output, and myocardial work. Patients with cardiomyopathy with or without prior congestive heart failure may be especially sensitive to cardiac volume overload and may become acutely symptomatic with these sudden shifts in volume status.
The diving reflex is a unique autonomic reflex response to water immersion that has direct relevance to the safety of this popular sport. It was first described in 1870 by the French physiologist Paul Bert, when he observed that heart rate slowed dramatically during forced water submersion. The full diving reflex response involves transient slowing of the heart rate (typically by 20 to 30 beats/min) with subsequent reduction in cardiac output and peripheral vasoconstriction to maintain systemic blood pressure.6 The reflex is mediated by increased vagal nerve activity. Heart rates lower than 10 beats/min have been measured in accomplished breath-hold divers.18 Although typically well tolerated, such intense bradycardia may result in supraventricular and ventricular escape arrhythmias in susceptible individuals.40
Individuals with underlying heart disease may tolerate diving poorly, as evidenced by data that document a significant percentage of diving fatalities attributable to cardiac causes. Depending on the age of the population, sudden cardiac death (SCD) and myocardial infarction account for 12% to 27% of diving fatalities.15 It is important to emphasize that this statistic does not mean that diving “caused” the fatality but rather that acute coronary events occur in middle-age divers who may not have access to usual emergency care if an event occurs during diving. Adequate screening for underlying cardiovascular disease is essential at a reasonable interval before any diving excursion, especially in patients with known disease. Individuals with significant cardiovascular disease, including incompletely revascularized CAD and cardiomyopathy/congestive heart failure, are typically at high risk for complications during diving and should be given restrictive recommendations. In addition, patients who have undergone coronary artery bypass grafting and have had chest tubes placed occasionally have scarring and air trapping that could place such individuals at risk for pneumothorax during compressed-air diving. At the very least, a chest radiograph should document no evidence for scarring before allowing such patients to dive; occasionally other tests for air trapping, such as chest computed tomography, could be indicated. Recommendations used to advise patients with cardiac disease who want to participate in competitive sports may be useful for diving and other wilderness activities as well.36 If patients have normal left ventricular function, are well revascularized, and have no provocable ischemia or arrhythmias, consideration could be given to individual patients to be allowed to dive. It must be emphasized that divers, should they become incapacitated during a dive, put not only themselves at risk, but also their diving partners. Therefore some recommendations may be more restrictive than just for individual assumption of risk.
Hypoxia (High-Altitude Activity)
The cardiovascular system’s response to hypoxia is a dynamic process that begins immediately on exposure and evolves over days, weeks, and years of prolonged exposure. Although the temporal nature of this response has inherent interindividual variability, it is useful to consider the response in two distinct phases: acute hypoxia and sustained hypoxia. Important changes in cardiovascular function during acute and sustained hypoxia are summarized in Figure 32-1.
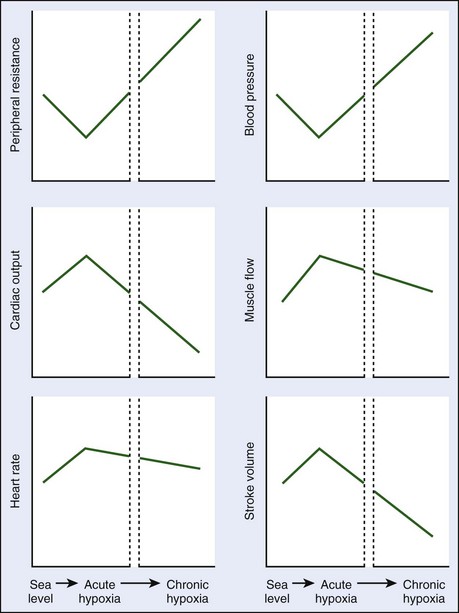
FIGURE 32-1 Changes in cardiovascular parameters during acute and sustained exposure to hypoxia.
(Modified from Baggish AL, Levine BD: The cardiovascular system at high altitude. In Horbein TF, Schoene RB: High altitude: An exploration of human adaptation, New York, [in press], Marcel Dekker, Inc.)
Acute hypoxia decreases alveolar and arterial oxygen content, leading to sympathetic nervous system activation.26,37 This activation is the key element of the acute hypoxic response of the cardiovascular system. Increased sympathetic activity (with or without vagal withdrawal) leads to increased heart rate and thus increased cardiac output. However, peripheral vasoconstriction, the expected vascular response to increased sympathetic tone, appears to be blunted by the release of local vasodilatory substances, constituting a phenomenon equivalent to the “functional sympatholysis” that occurs during sustained endurance exercise.23 Cardiac output increases while systemic vascular resistance and blood pressure decrease transiently. Acute hypoxia also causes dilation of the coronary arteries, although in patients with CAD who may have endothelial dysfunction, appropriate coronary dilation may not occur and may even be associated with paradoxical vasoconstriction.5
As hypoxia becomes sustained, arterial oxygen concentration (CaO2) increases secondary to hemoconcentration, ventilatory acclimatization, and increases in red cell mass. These changes lead to some abatement of the hyperdynamic state of the circulatory system. Ventricular contractile function remains normal, but reductions in plasma volume and ventricular filling lead to decreased stoke volume as predicted by the Frank-Starling mechanism.24 Persistent sympathetic hyperactivity leads to downregulation of cardiac β-receptors45 and increased vagal activity,10 which combine to promote a reduction in resting heart rate. However, resting heart rate remains elevated during sustained hypoxia when compared with sea-level conditions. These heart rate and stroke volume reductions lead to an overall fall in cardiac output, especially at maximal exercise. In the systemic circulation, blood pressure gradually rises as the peripheral mechanisms responsible for local vasodilation diminish. For patients with hypertension, this sympathetic activation may result in substantial increases in blood pressure during high-altitude excursions.
High-altitude activities, such as hiking, trekking, and mountaineering, also generally include sustained, low-intensity exercise for prolonged periods of time. The combination of reduced arterial oxygen content and perhaps paradoxical vasoconstriction during exercise (increased myocardial oxygen demand) results in provocation of myocardial ischemia with acute altitude exposure at work rates somewhat less than that provoked at sea level.32 However, with at least 5 days of acclimatization, responses near those noted at sea level can be restored. Mountain walking, even at the relatively moderate altitudes seen in the Alps, is associated with a slightly increased risk for SCD,11 although this risk seems greatest in persons who are least fit. Overall, there is one SCD per 780,000 hiking hours and one SCD per 1,630,000 skiing hours in the Alps,11 and one “cardiac event” per 957,000 hours of mountain activities,41 which increases substantially in individuals over the age of 50 to 60 years. This risk seems somewhat higher than the one death per 3,000,000 jogging hours reported by Thompson and colleagues.43 For patients with known CAD, if they are adequately revascularized, exercise at high altitude appears reasonably safe.17,42
Hyperthermia
The healthy human body is capable of tolerating and even successfully performing vigorous physical exercise during exposure to high ambient temperature. The hypothalamic thermoregulatory center prevents elevation of core body temperature during ambient heat exposure by causing peripheral vasodilation and increased sweat production. Humans thermoregulate predominantly by sending blood to the skin; during an acute heat stress, up to 8 to 10 L/min of cardiac output may be directed to the skin (and therefore unavailable to other organs) to maintain body temperature. Especially for persons with compromised circulation, such as with heart failure (HF),4,13 heat exposure may be poorly tolerated. With ample oral hydration and electrolyte repletion, moderate physical exercise can be performed in the face of significant thermal exposure for extended periods, although safe performance requires adequate heat acclimatization. The absolute intensity and duration of work that can be performed in the heat by a given individual are dictated by numerous factors, including prior heat acclimatization, physical fitness, and ambient temperature and relative humidity.
Heatstroke occurs when the cooling system fails and core body temperature rises. Heatstroke is defined clinically as a core body temperature that rises above 42° C (107.6° F), with signs and symptoms including hot, dry skin and central nervous system abnormalities such as delirium, convulsions, or coma.9 Cardiovascular complications of heatstroke include atrial fibrillation, various electrocardiographic abnormalities (T-wave changes and prolongation of the QT interval), pulmonary edema, pericardial effusion, right ventricular dysfunction, hypotension, and ventricular arrhythmias.46
Hypothermia
Classically hypothermia is defined as a core body temperature of less than 35° C (95° F). Hypothermia may develop in numerous wilderness scenarios, including but not limited to cold-water immersion, snow burial, and exercise in cool to cold ambient temperatures. Unlike heat exposure, humans do not acclimatize to an appreciable degree to cold exposure, so survival in the cold depends on behavior (e.g., clothing, shelter). The initial response to cold exposure is increased sympathetic nervous system activity leading to peripheral vasoconstriction and tachycardia.28 This causes a rise in systemic arterial blood pressure and a tendency toward central redistribution of blood volume. Acute exercise in the cold, especially in patients with CAD, is associated with increased risk for acute coronary events.
With sustained mild hypothermia (core body temperature of 33° to 35° C [91.4° to 95° F]), this central volume shift promotes diuresis, which can lead to dehydration. As hypothermia becomes more pronounced (moderate hypothermia is 30° to 33° C [86° to 91.4° F]), heart rate and cardiac output fall and the electrocardiogram may show characteristic findings of prolonged QT interval or Osborn waves. Bradycardia, caused by direct slowing of sinoatrial function and concomitant changes in tissue pH, oxygen concentration, and electrolyte concentrations, becomes more marked as core temperature continues to drop.33 The drops in core temperature and pulse rate typically observe a linear relationship; deviations from this relationship, particularly tachycardia in the moderately hypothermic patient, should raise suspicion of a secondary process, such as toxin ingestion or hypovolemia. Premature atrial and/or ventricular beats and atrial fibrillation commonly occur in the moderately hypothermic person.
In severe hypothermia (<30° C [86° F]), there are significant reductions in stroke volume, heart rate, and blood pressure. All types of atrial and ventricular tachyarrhythmias can occur during severe hypothermia.14 It should be noted that rough handling or jostling of the severely hypothermic patient could trigger arrhythmias, so care should be taken to avoid unnecessary physical manipulation of the hypothermic patient.28 At temperatures below 25° C (77° F), spontaneous ventricular fibrillation and/or asystole may occur. As discussed in further detail below, victims of hypothermia may tolerate periods of cardiac arrest longer than do victims under normothermic conditions. Thus the appropriate window for initiating and continuing resuscitation for a hypothermic patient may exceed that in other cardiac arrest situations.
Screening and Preparation for Wilderness Travel
Asymptomatic Wilderness Adventurers
A comprehensive physical examination should be performed, beginning with a basic assessment of vital signs to exclude systemic hypertension and arrhythmia. Auscultation of the heart and lungs should be performed to exclude congenital or acquired valvular heart lesions and pulmonary vascular congestion. Peripheral pulse examination should be performed to detect the presence of atherosclerotic peripheral vascular disease and aortic coarctation. Although not specifically designed for wilderness travelers, the American College of Cardiology/American Heart Association’s athletic pre-participation medical history and physical examination template may prove to be a useful guide for clinicians in this setting.35
Established Coronary Artery Disease
Individuals with coronary artery disease should not necessarily be restricted from wilderness activity. Patients with clinically stable ACAD (i.e., patients fully revascularized or incompletely revascularized but without recent change in anginal threshold) who are on a well-tolerated medical regimen should not universally be excluded from wilderness activity. We recommend that all individuals with established ACAD undergo some form of exercise stress testing before wilderness activity, because exercise stress testing provides an accurate assessment of both exercise capacity and the efficacy of medical therapy. Stress testing using continuous 12-lead electrocardiographic monitoring can be done using a number of exercise modalities, including treadmill running/walking and upright or supine cycle ergometry. Typical electrocardiographic findings of myocardial ischemia are illustrated in Figure 32-2. The choice of exercise modality can be made based on patient and clinician preferences. Concomitant myocardial imaging may be necessary to achieve adequate diagnostic accuracy in patients with certain pretest features (Box 32-1). Imaging can be performed using nuclear perfusion tracers or echocardiography as dictated by available expertise. Individuals with clinically stable ACAD who have normal left ventricular function and no myocardial ischemia during exercise testing performed at least to a workload commensurate with the demands of the expected activity should not be restricted from wilderness activity based on cardiovascular disease.
Cardiomyopathy/Congestive Heart Failure
In addition to sudden death risk, cardiomyopathy may lead to development of heart failure (HF). HF is a syndrome defined as inability of the circulatory system to provide adequate circulation of blood to meet the demands of the body and to fill adequately at a low enough pressure to avoid congestion. HF severity can be assessed based on symptom severity and the results of an assessment of cardiac structure and function. Recently updated practice guidelines present an HF severity classification scheme that incorporates symptom severity and cardiac structural/functional parameters (Table 32-1).25 Cardinal symptoms of HF include dyspnea, fatigue, exercise intolerance, and systemic venous congestion manifesting most commonly as peripheral edema. Noninvasive imaging such as echocardiography can provide important information such as left ventricular size and ejection fraction.
TABLE 32-1 ACC/AHA Classification of the Stages of Heart Failure
Heart Failure Stage | Definition |
---|---|
At Risk for Heart Failure | |
Stage A | Patients at high risk for developing heart failure in the future but no functional or structural heart disorder |
Stage B | Structural heart disorder but no symptoms of prior heart failure at any stage |
Heart Failure Present | |
Stage C | Previous or current symptoms of heart failure in the context of an underlying structural heart disorder |
Stage D | Advanced disease requiring hospital-based support, a heart transplant, or palliative care |
ACC, American College of Cardiology; AHA, American Heart Association.
Congenital Heart Disease
Congenital heart disease (CHD) is a term that includes a wide array of cardiac abnormalities that are present at birth. CHD can include abnormalities of the heart muscle, cardiac valves, coronary arteries, and electrical conduction system and may be confined to the heart or a component of a systemic disorder. From a functional perspective, CHD can be categorized as either cyanotic or acyanotic. Cyanotic CHD is characterized by reduced arterial oxygen saturation due to right-to-left intracardiac shunting with direct venous to arterial transit of blood. Cyanotic CHD is relatively rare and involves complex and severe structural cardiac malformations, such as tetralogy of Fallot. Most individuals with cyanotic CHD present early in life because of failure to thrive or markedly reduced exercise capacity and are treated with surgical reconstruction. Individuals with complex cyanotic CHD are at high risk for decompensation during wilderness activity and should be restricted from wilderness activity for this reason. Among patients with successful surgical correction of cyanotic CHD, the risk associated with wilderness activity is highly variable. For example, patients who have undergone a surgical Fontan procedure and who do not have right-to-left shunting tolerate acute exposure to high altitude surprisingly well.21 Nevertheless, the impact of chronic exposure to high altitude in such patients has not been well defined. The decision to prohibit or restrict activity in this patient population should be made on a case-by-case basis in consultation with a cardiovascular specialist with expertise in CHD.
The second is patent foramen ovale. This condition is quite common, present in perhaps 5% of the adult population, and has been associated with acute mountain sickness and high-altitude pulmonary edema.1,31 However, there is no evidence that closing a patent foramen ovale has any effect on exercise performance at altitude or the risk for high-altitude illness, and such a procedure should be avoided unless there are other strong clinical conditions. For individuals with a known patent foramen ovale wishing to go to high altitude, consideration should be given for high-altitude pulmonary edema prophylaxis with a pulmonary vasodilator such as nifedipine39 or tadalafil.34
Implantable Cardioverter-Defibrillators and Pacemakers
Implantable cardioverter-defibrillators (ICDs) are placed for the primary and secondary prevention of SCD in patients susceptible to malignant ventricular arrhythmias. Recent expansion of ICD appropriateness criteria has lead to continually increasing numbers of patients with these devices.16 By definition, individuals with ICDs are at high risk for arrhythmia and subsequent device discharge during strenuous activity and therefore should avoid all high-intensity exercise. Device discharge may be painful and may render the recipient temporarily incapacitated. Accordingly, all wilderness activities during which transient compromises in cognitive or motor function may have grave consequences to the patient or partners are contraindicated in patients with ICDs. Examples include underwater diving, rock climbing, and hang gliding. Low- to moderate-intensity exercise may be appropriate for patients with ICDs. We encourage careful assessment of heart rate and rhythm response during formal exercise testing in ICD patients who choose to pursue such activities.
Basic Management of Cardiovascular Emergencies in the Wilderness Environment
Chest Pain
Myocardial ischemia most commonly manifests with chest discomfort. The quality of this discomfort varies among individuals and may be reported as heaviness, tightness, or a dull ache. The chest discomfort attributable to myocardial ischemia is often accompanied by other symptoms, including diaphoresis, nausea, dyspnea, or pain in adjacent anatomic structures, including the neck, jaw, or left arm. Among wilderness adventurers, new-onset chest discomfort without a clear musculoskeletal cause should be considered an ominous sign. It should be assumed that nontraumatic chest discomfort is indicative of an active coronary artery process until proved otherwise. Definitive and effective therapy for active myocardial ischemia requires access to hospital-based resources.2,3 As such, wilderness travelers who experience chest discomfort suspected to be of myocardial ischemic origin should place emphasis on gaining access to a capable medical facility with minimal delay. In some cases, assistance from search and rescue programs with the capability to provide rapid transport may be appropriate. In the field, acetylsalicylic acid (aspirin), preferably in a chewable, rapidly absorbed form, should be given immediately once myocardial ischemia is suspected. Nitroglycerin-based agents, additional anticoagulants, including low-molecular-weight heparin, and analgesic medications may be appropriate if administered in the field by a clinician trained in their use.
Arrhythmias
Management of the symptomatic patient with suspected pathologic tachyarrhythmia should focus on rapid transportation to a medical facility with advanced cardiac life support capabilities.8 When transportation to professional medical attention is not an immediate option, several therapeutic maneuvers can be attempted. Carotid sinus massage can be used to terminate several forms of tachyarrhythmia. External pressure on the carotid sinus stimulates baroreceptors that trigger increased vagus nerve activity and decreased sympathetic nervous system activity, thereby potentially terminating the arrhythmia. The patient with tachyarrhythmia should be placed in a supine or lateral decubitus position with the neck fully extended and turned away from the care provider. The carotid pulse should be palpated close to the angle of the jaw to approximate the common carotid artery bifurcation. Firm pressure should then be applied with a gentle rotating motion for 5 seconds. If there is no response, the procedure should be attempted on the patient’s other side. The procedure should never be performed on both sides simultaneously. In patients who do not respond to carotid sinus massage, alternative vagus nerve stimulation maneuvers may be attempted. Prolonged breath holding, a Valsalva maneuver, putting the face in cold water (to induce the “diving reflex”), or a strong cough against a closed glottis may also cause a vagal response adequate to terminate a tachyarrhythmia.
Syncope
Syncope is defined as transient loss of consciousness accompanied by loss of postural tone. Syncope occurs because of rapid reduction in cerebral blood flow culminating in significant central nervous system hypoperfusion. There are numerous causes of syncope. Syncope may be attributable to an underlying cardiovascular pathologic condition, including structural, valvular, or electrical heart disease. Such underlying processes need to be considered when evaluating any patient with a prior syncopal event. Most commonly, syncope occurs in healthy individuals without explanatory heart disease and is attributable to autonomic mechanisms (vasovagal or neurally mediated syncope). The physiology of neurally mediated syncope is beyond the scope of this text; the interested reader is referred to several excellent reviews of this topic.27,29,38
Neurally mediated syncope is common among physically fit individuals who may choose to engage in wilderness activity12 and has been reported to occur during the early phases of altitude acclimatization as a result of plasma volume loss, hyperventilation, and perhaps hypoxia-mediated vasodilation. Individuals with a predisposition for neurally mediated syncope may be at increased risk for syncope during wilderness activity that produces dehydration (prolonged strenuous exercise) and/or significant peripheral vascular dilation (thermal exposure). The individual with neurally mediated syncope will typically report presyncopal feelings of warmth, diaphoresis, or light-headedness, which culminate in loss of consciousness ranging from several seconds to minutes.
Cardiac Arrest
The most recent American Heart Association/American College of Cardiology consensus guidelines advocate a CAB or circulation–airway–breathing approach to basic life support.7 This strategy involves immediate initiation of chest compressions for unconscious patients without spontaneous or normal cardiorespiratory function. The logic for this paradigm shift is that there is ample oxygen present in the lungs and arterial system for several minutes following cardiac arrest to avoid ischemia if blood can reach the target organs. Thus immediate circulation of blood via chest compressions will facilitate optimal oxygenation of the brain and other key organs sooner than the conventional airway-first approach. The currently recommended sequence of adult basic life support can be summarized as follows:
The success of cardiac arrest resuscitation typically requires access to advanced cardiac life support equipment and trained personnel. This may not be a reality when cardiac arrest occurs in wilderness environments. Cardiac arrest patients who do not respond to resuscitation efforts during the first 15 to 30 minutes of basic life support are very unlikely to recover any significant degree of brain function, even if spontaneous cardiopulmonary function is restored. The decision of when to terminate resuscitation in a wilderness setting is challenging. Recommendations for this task have been developed.22 In general, it is reasonable to terminate resuscitation efforts after 30 minutes if a spontaneous pulse has not been restored. An extension beyond this time limit should be considered for the hypothermic patient because hypothermia may extend the window of potential central nervous system survival. Current resuscitation guidelines provide recommendations for specific wilderness situations (Table 32-2).44
TABLE 32-2 Considerations for Resuscitation of Cardiac Arrest Victims in Specific Wilderness Situations
Wilderness Situation | Special Considerations |
---|---|
Trauma |
Finally, there are certain situations in which resuscitation may be deferred in the wilderness environment. These include the following: (1) Any situation in which resuscitation efforts would put rescuers at risk, (2) a victim with a core body temperature of less than 15° C (59° F), (3) a victim with a frozen chest wall, (4) a victim who has been submersed in cold water for more than 60 minutes, (5) a victim with an obvious lethal injury such as decapitation, and (6) a situation in which resuscitation would significantly delay evacuation of a hypothermic patient to controlled rewarming.22
1 Allemann Y, Hutter D, Lipp E, et al. Patent foramen ovale and high-altitude pulmonary edema. JAMA. 2006;296:2954.
2 Anderson JL, Adams CD, Antman EM, et al. ACC/AHA 2007 guidelines for the management of patients with unstable angina/non-ST-elevation myocardial infarction: A report of the American College of Cardiology/American Heart Association Task Force on Practice Guidelines (Writing Committee to Revise the 2002 Guidelines for the Management of Patients With Unstable Angina/Non-ST-Elevation Myocardial Infarction) developed in collaboration with the American College of Emergency Physicians, the Society for Cardiovascular Angiography and Interventions, and the Society of Thoracic Surgeons endorsed by the American Association of Cardiovascular and Pulmonary Rehabilitation and the Society for Academic Emergency Medicine. J Am Coll Cardiol. 2007;50:e1.
3 Antman EM, Anbe DT, Armstrong PW, et al. ACC/AHA guidelines for the management of patients with ST-elevation myocardial infarction—executive summary: A report of the American College of Cardiology/American Heart Association Task Force on Practice Guidelines (Writing Committee to Revise the 1999 Guidelines for the Management of Patients With Acute Myocardial Infarction). J Am Coll Cardiol. 2004;44:671.
4 Arbab-Zadeh A, Crandall CG, Levine BD. Thermoregulation in patients with cardiac disease. J Cardiopulm Rehabil. 2002;22:38.
5 Arbab-Zadeh A, Levine BD, Trost JC, et al. The effect of acute hypoxemia on coronary arterial dimensions in patients with coronary artery disease. Cardiology. 2009;113:149.
6 Asmussen E, Kristiansson NG. The “diving bradycardia” in exercising man. Acta Physiol Scand. 1968;73:527.
7 Berg RA, Hemphill R, Abella BS, et al. Part 5: Adult basic life support: 2010 American Heart Association guidelines for cardiopulmonary resuscitation and emergency cardiovascular care. Circulation. 2010;122:S685.
8 Blomstrom-Lundqvist C, Scheinman MM, Aliot EM, et al. ACC/AHA/ESC guidelines for the management of patients with supraventricular arrhythmias—executive summary: A report of the American College of Cardiology/American Heart Association Task Force on Practice Guidelines and the European Society of Cardiology Committee for Practice Guidelines (Writing Committee to Develop Guidelines for the Management of Patients With Supraventricular Arrhythmias) developed in collaboration with NASPE-Heart Rhythm Society. J Am Coll Cardiol. 2003;42:1493.
9 Bouchama A, Knochel JP. Heat stroke. N Engl J Med. 2002;346:1978.
10 Boushel R, Calbet JA, Radegran G, et al. Parasympathetic neural activity accounts for the lowering of exercise heart rate at high altitude. Circulation. 2001;104:1785.
11 Burtscher M, Philadelphy M, Likar R. Sudden cardiac death during mountain hiking and downhill skiing. N Engl J Med. 1993;329:1738.
12 Colivicchi F, Ammirati F, Santini M. Epidemiology and prognostic implications of syncope in young competing athletes. Eur Heart J. 2004;25:1749.
13 Cui J, Arbab-Zadeh A, Prasad A, et al. Effects of heat stress on thermoregulatory responses in congestive heart failure patients. Circulation. 2005;112:2286.
14 Duguid H, Simpson RG, Stowers JM. Accidental hypothermia. Lancet. 1961;2:1213.
15 Edmonds C. Why divers die: The facts and figures. In: Edmonds C, Lowry C, Pennefather J, et al, editors. Diving and subaquatic medicine. London: Arnold; 2002:479.
16 Epstein AE, DiMarco JP, Ellenbogen KA, et al. ACC/AHA/HRS 2008 guidelines for device-based therapy of cardiac rhythm abnormalities: A report of the American College of Cardiology/American Heart Association Task Force on Practice Guidelines (Writing Committee to Revise the ACC/AHA/NASPE 2002 Guideline Update for Implantation of Cardiac Pacemakers and Antiarrhythmia Devices) developed in collaboration with the American Association for Thoracic Surgery and Society of Thoracic Surgeons. J Am Coll Cardiol. 2008;51:e1.
17 Erdmann J, Sun KT, Masar P, et al. Effects of exposure to altitude on men with coronary artery disease and impaired left ventricular function. Am J Cardiol. 1998;81:266.
18 Ferringo M, Lundgren CE. Breath hold diving. In: Brubalek A, Newman TS, editors. Physiology and medicine of diving. New York: WB Saunders; 2003:161.
19 Gabrielsen A, Johansen LB, Norsk P. Central cardiovascular pressures during graded water immersion in humans. J Appl Physiol. 1993;75:581.
20 Gabrielsen A, Warberg J, Christensen NJ, Bie P, Stadeager C, Pump B, Norsk P. Arterial pulse pressure and vasopressin release during graded water immersion in humans. Am J Physiol Regul Integr Comp Physiol. 2000;278:R1583.
21 Garcia JA, McMinn SB, Zuckerman JH, et al. The role of the right ventricle during hypobaric hypoxic exercise: Insights from patients after the Fontan operation. Med Sci Sports Exerc. 1999;31:269.
22 Goth P, Garnett G. Clinical guidelines for delayed/prolonged transport: I. Cardiorespiratory arrest: Rural affairs committee, National Association of Emergency Medical Services Physicians. Prehosp Disast Med. 1991;6:335.
23 Heistad DD, Abboud FM, Dickinson W. Richards lecture: Circulatory adjustments to hypoxia. Circulation. 1980;61:463.
24 Hoon RS, Balasubramanian V, Mathew OP, et al. Effect of high-altitude exposure for 10 days on stroke volume and cardiac output. J Appl Physiol. 1977;42:722.
25 Jessup M, Abraham WT, Casey DE, et al. 2009 Focused update: ACCF/AHA guidelines for the diagnosis and management of heart failure in adults: A report of the American College of Cardiology Foundation/American Heart Association Task Force on Practice Guidelines: Developed in collaboration with the International Society for Heart and Lung Transplantation. Circulation. 2009;119:1977.
26 Koller EA, Drechsel S, Hess T, et al. Effects of atropine and propranolol on the respiratory, circulatory, and ECG responses to high altitude in man. Eur J Appl Physiol Occup Physiol. 1988;57:163.
27 Kosinski D, Grubb BP, Karas BJ, et al. Exercise-induced neurocardiogenic syncope: Clinical data, pathophysiological aspects, and potential role of tilt table testing. Europace. 2000;2:77.
28 Lauri T, Leskinen M, Timisjarvi J, et al. Cardiac function in hypothermia. Arctic Med Res. 1991;50:63.
29 Levine BD. Regulation of central blood volume and cardiac filling in endurance athletes: The Frank-Starling mechanism as a determinant of orthostatic tolerance. Med Sci Sports Exerc. 1993;25:727.
30 Levine BD. Textbook of exercise and sports cardiology. New York: McGraw Hill; 2000.
31 Levine BD, Grayburn PA, Voyles WF, et al. Intracardiac shunting across a patent foramen ovale may exacerbate hypoxemia in high-altitude pulmonary edema. Ann Intern Med. 1991;114:569.
32 Levine BD, Zuckerman JH, deFilippi CR. Effect of high-altitude exposure in the elderly: The tenth mountain division study. Circulation. 1997;96:1224.
33 Maaravi Y, Weiss AT. The effect of prolonged hypothermia on cardiac function in a young patient with accidental hypothermia. Chest. 1990;98:1019.
34 Maggiorini M, Brunner-La Rocca HP, Peth S, et al. Both tadalafil and dexamethasone may reduce the incidence of high-altitude pulmonary edema: A randomized trial. Ann Intern Med. 2006;145:497.
35 Maron BJ, Thompson PD, Ackerman MJ, et al. Recommendations and considerations related to preparticipation screening for cardiovascular abnormalities in competitive athletes: 2007 Update: A scientific statement from the American Heart Association Council on Nutrition, Physical Activity, and Metabolism: Endorsed by the American College of Cardiology Foundation. Circulation. 2007;115:1643.
36 Maron BJ, Zipes DP. Introduction: Eligibility recommendations for competitive athletes with cardiovascular abnormalities—general considerations. J Am Coll Cardiol. 2005;45:1318.
37 Mazzeo RS, Bender PR, Brooks GA, et al. Arterial catecholamine responses during exercise with acute and chronic high-altitude exposure. Am J Physiol. 1991;261:E419.
38 Mosqueda-Garcia R, Furlan R, Tank J, et al. The elusive pathophysiology of neurally mediated syncope. Circulation. 2000;102:2898.
39 Oelz O, Noti C, Ritter M, et al. Nifedipine for high altitude pulmonary oedema. Lancet. 1991;337:556.
40 Olsen CR, Fanestil DD, Scholander PF. Some effects of breath holding and apneic underwater diving on cardiac rhythm in man. J Appl Physiol. 1962;17:461.
41 Ponchia A, Biasin R, Tempesta T, et al. Cardiovascular risk during physical activity in the mountains. J Cardiovasc Med (Hagerstown). 2006;7:129.
42 Schmid JP, Noveanu M, Gaillet R, et al. Safety and exercise tolerance of acute high altitude exposure (3454 m) among patients with coronary artery disease. Heart. 2006;92:921.
43 Thompson PD, Funk EJ, Carleton RA, et al. Incidence of death during jogging in Rhode Island from 1975 through 1980. JAMA. 1982;247:2535.
44 Vanden Hoek TL, Morrison LJ, Shuster M, et al. Part 12: Cardiac arrest in special situations: 2010 American Heart Association guidelines for cardiopulmonary resuscitation and emergency cardiovascular care. Circulation. 2010;122:S829.
45 Voelkel NF, Hegstrand L, Reeves JT, et al. Effects of hypoxia on density of beta-adrenergic receptors. J Appl Physiol. 1981;50:363.
46 Zahger D, Moses A, Weiss AT. Evidence of prolonged myocardial dysfunction in heat stroke. Chest. 1989;95:1089.