Chapter 14 What Is the Best Method of Rehabilitation after Flexor Tendon Repair in Zone II: Passive Mobilization or Early Active Motion? What Is the Best Suture Configuration for Repair of Flexor Tendon Lacerations?
Restoration of satisfactory digital function after flexor tendon laceration and repair continues to be one of the most difficult problems in hand surgery. Early efforts to improve the performance of flexor tendon repairs are largely based on individual anecdotal experience, historical precedence, and clinical experimentation with little or no scientific support. Methods to repair flexor tendons have undergone a notable evolution since the 1950s. Early primary repair of flexor tendons in zone II, once called “no man’s land,” has replaced tendon grafting as the standard of care. Rehabilitation after repair of flexor tendon injuries has also evolved from complete immobilization to early passive motion and now early active motion. Nonetheless, the optimal treatment of a flexor tendon laceration in zone II remains an unresolved challenge for hand surgeons to define.1 The basic tenet of current and historical investigative efforts has been to improve the strength of tendon repair to allow for earlier motion, thereby preventing adhesion formation. Recent studies have contributed to a better understanding of the biology of flexor tendon injuries, improved methods of tendon repair, a greater emphasis on flexor sheath and pulley management, and the development of early controlled motion rehabilitation protocols leading to better clinical results.2 The purpose of this chapter is to provide a concise review of Level I and II studies on flexor tendon injury repair techniques and postoperative rehabilitation protocols.
TENDON REPAIR CONSIDERATIONS
During early phases of tendon healing, repair site strength is primarily dependent on the strength of the suture repair method. Strickland2 describes six principles of an ideal repair: (1) easy placement of sutures in the tendon, (2) secure suture knots, (3) smooth juncture of tendon ends, (4) minimal gapping at the repair site, (5) minimal interference with tendon vascularity, and (6) sufficient strength throughout healing to permit the application of early motion stress to the tendon.2 To satisfy these characteristics and therefore permit earlier active tendon mobilization, various suture techniques have been described that reportedly provide increased strength. Initial repair-site strength is roughly proportional to the intrinsic properties of the type of suture used, the number of suture strands traversing the repair site, and the number of grasping loops incorporated into the repair. Currently, hand surgeons agree that flexor tendon repairs should include a grasping or locking suture within the tendon, the “core” suture, and a continuous circumferential or “epitendinous” suture around the laceration site (Level IV). Addition of an epitendinous finishing suture has been shown to be of benefit in providing added tensile strength and gap resistance, as well as preventing triggering from uneven suture lines (Level IV).
CORE SUTURE CONFIGURATION
Early reports of active motion of tendons repaired with conventional two-strand repair documented rupture rates of up to 10%. Traditional two-strand suture methods are not sufficiently strong to consistently allow for early active digital motion. For this reason, several multistrand tendon suture techniques have been described. These techniques include the Kessler, Tajima, Savage, Lee, Tsuge, Tang, Sandow, and cruciate repair of Wolfe.3–8 Recent in vitro studies have evaluated the biomechanical properties of various suture methods for flexor tendon repair in the canine model. Increased ultimate strength of repair was reported for the multistrand and multiple-grasping methods of Lee (38 N), Savage (53 N), and an eight-strand technique.9 In vivo analysis demonstrated significant increases in strength for multistrand repair methods compared with traditional two-strand repairs at both 3 and 6 weeks in canine models. Reported ultimate strength values for an eight-strand repair was 52.6 and 70.9 N at 3 and 6 weeks, respectively.10 Ex vivo and in vivo studies in human and canine models suggest that core suture configurations with the greatest tensile strength are those in which there are multiple sites of tendon suture interaction. The addition of a circumferential suture may increase the strength of core repairs by 10% to 50%, reduce gapping between tendon ends, and smooth the repair site.11 Other variables shown to have a positive effect on the repair strength include a dorsovolar location of the core suture, adding locking or grasping stitches, and increasing the cross-sectional area of tendon that is grasped or locked by the redirecting loop of suture.12 Ex vivo human model studies have demonstrated that greater strength is achieved with more dorsal rather than volar placement of the core suture within the tendon.13–15 Positioning the redirecting loop of the core suture to “lock” rather than “grasp” the tendon stumps and increasing the number of suture locks or grasps further provides greater tensile strength of the repair site.16,17
Schuind and colleagues18 report forces across flexor tendons of 0.9 kilogram force (kgf; 8.9 N) and 3.5 kgf (34.4 N) for passive and active digital motion, respectively, in an in vivo study of patients undergoing carpal tunnel surgery (Table 14-1). These values increased significantly to 12 kgf (117.5 N) with fingertip pinch. Urbaniak and coauthors19 report an average tension in a human profundus tendon to be 14.7 N and found that tensile strength of tendon repairs decreases to approximately one fifth of its initial strength at 1 week. Taking into account increased resistance from edema after surgery and a decrease in suture strength during the initial weeks after repair, Urbaniak and coauthors19 and Savage20 therefore suggest that initial repair strength equal or exceed five times the average tension, 73.5 N, to withstand gentle or moderate active finger motion. To this end, numerous published investigations base treatment recommendations on Urbaniak19 and Schuind’s18 reported in vivo force values.
TYPE | KILOGRAMS FORCE | NEWTONS |
---|---|---|
Passive mobilization of wrist | 0.6 | 5.9 |
Passive mobilization of fingers | 0.9 | 8.9 |
Unresisted active mobilization of wrist | 0.4 | 3.9 |
Unresisted active mobilization of fingers | 3.5 | 34.4 |
Grasp (flexor digitorum profundus) | 6.4 | 62.9 |
Tip pinch | 12.0 | 117.5 |
Data from Schuind F, Garcia-Elias M, Cooney WP 3rd, An KN: Flexor tendon forces: In vivo measurements. J Hand Surg [Am] 17:291–298, 1992.
Tang and coworkers21 demonstrate in a human cadaver study that four newly developed suture methods (Fig. 14-1), the Tang, Silfverskiöld, Robertson, and cruciate, were biomechanically superior to the modified Kessler suture method when subjected to mechanical loads using the Instron tensile machine.The Tang method possessed the greatest ultimate strength (53.6 N), 2-mm gap formation force (43.0 N), energy to failure, and capacity to resist tendon deformation among the five tested techniques. The cruciate method showed statistically higher tensile strength and energy to failure compared with the Robertson, Silfverskiöld, and modified Kessler methods. The gap formation force, ultimate strength, elastic modulus, and energy to failure were lowest for the modified Kessler method (Table 14-2).
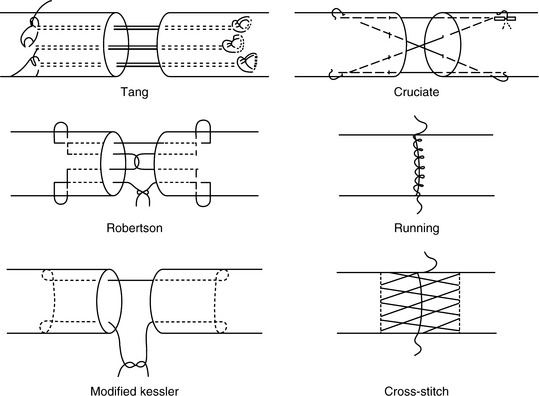
FIGURE 14-1 Schematic illustration of tendon suture techniques.
(Adapted from Tang JB, Gu YT, Rice K, et al: Evaluation of four methods of flexor tendon repair for postoperative active mobilization. Plast Reconstr Surg 107:742–749, 2001, by permission.)
TABLE 14-2 Gap Formation Force, Ultimate Strength, and Energy to Failure of Repaired Tendons (Mean ± Standard Deviation)
Labana and investigators22 similarly evaluated the biomechanical properties of three types of repairs—the standard Kessler–Tajima, double-loop (four-strand) modified Tsuge, and triple-loop (six-strand) modified Tsuge—in a human cadaver study. After subjecting the various repairs to mechanical testing using the Instron machine, the authors showed that the six-strand Tsuge suture was significantly stronger than both repairs, and that the four-strand Tsuge was significantly stronger than the Kessler–Tajima suture in terms of force to failure and initial gapping. Supramid 4–0 was used for core stitches in the modified Tsuge repairs and braided 4–0 nylon in the Kessler–Tajima repairs. 6–0 Prolene was used for epitenon repair in all cases. Labana and investigators22 conclude that an ultimate tensile strength of 64 N for the six-strand modified Tsuge repair and 48 N for the four-strand modified Tsuge repair exceeds the forces measured by Schuind and colleagues18 and, therefore, should withstand early range-ofmotion rehabilitation protocols (Table 14-3).
TABLE 14-3 Mean Force at Failure and Force to Initial Gapping for Three Repairs
REPAIR TYPE | MEAN ULTIMATE FORCE TO FAILURE (SD), N | MEAN INITIAL GAPPING (SD), N |
---|---|---|
Kessler-Tajima | 31.8 (8.8) | 29.6 (9.2) |
Four-strand Tsuge | 48.4 (10.7) | 40.7 (12.3) |
Six-strand Tsuge | 64.2 (11.0) | 56.1 (9.7) |
SD, standard deviation.
Data from Labana N, Messer T, Lautenschlager E, et al: A biomechanical analysis of the modified Tsuge suture technique for repair of flexor tendon lacerations. J Hand Surg [Br] 26:297–300, 2001.
Xie and researchers23 compared the biomechanical properties of three six-strand tendon repair techniques with different configurations of core sutures: the modified Savage (Sandow’s method), Tang, and Lim (Fig. 14-2; Table 14-4). 4–0 Ethilon was used in the modified Savage repairs and 4–0 Supramid in the Tang and Lim repairs in addition to a 6–0 Ethilon running peripheral suture in all cases. Statistically, ultimate strength of the modified Savage (57.8 N) and Tang (60.2 N) methods were similar and significantly higher than that of the Lim method (51.3 N). Gap formation force was also greater in the Tang method compared with the modified Savage and Lim methods. In addition, results indicate a significant difference between mode of failure between the modified Savage and the Tang or Lim methods. Tendons repaired with the modified Savage method failed predominantly by suture breakage, which suggests that this repair may be strengthened by a larger caliber suture, whereas tendons repaired with the Tang and Lim methods failed mostly by suture pullout. The results of this study demonstrate that repair strength significantly varies with different configurations of six-strand repairs, and that location, number of locking junctions, and orientation of core sutures play an important role in repair strength despite an equal number of strands crossing the repair site.
TABLE 14-4 Characteristics in Locking Junctions with the Tendon and Orientations of Core Sutures in Three Six-Strand Repair Techniques
Although in vitro studies can provide basic biomechanical data concerning specific repair techniques, it is impossible to reproduce in vitro the in vivo physiologic environment in which repaired human tendons heal. The weakness of in vitro studies is that actual healing does not take place, and mechanical testing in tendons without prior trauma may not accurately account for increases in work of flexion from postsurgical edema, stiffness, and adhesion formation. Until recently, most in vitro studies used a simple linear testing model to evaluate tensile strength in terms of extra-anatomic longitudinally applied loads. Komanduri and colleagues14 propose using a dynamic “curvilinear” human cadaver model to test the strength of tendon repairs to more accurately simulate repair strength in vivo and account for biomechanical factors such as angulation at the repair site, differential loading, and frictional interference.
Komanduri and colleagues14 show that dorsal tendon repairs using Kessler or Bunnell core suture techniques were stronger than the standard volar repair.14 Suture material was limited to 4–0 nylon core sutures and 6–0 nylon circumferential sutures. In all cases with and without epitenon repair, dorsally placed sutures provided significantly more tensile strength than palmarly placed sutures (Table 14-5).
Historically, concern has existed that crossing or cruciate sutures may not be as desirable as linear suture techniques because they may interfere with the blood supply of the tendon. In addition, the blood supply to the tendon is located in the dorsal portion of the tendon, which happens to be the stronger part of the tendon to anchor sutures. Because tendon nutrition is predominantly synovial, the effect compromising the blood supply by suture is theoretical and has not been evaluated experimentally or clinically. Expanding on Komanduri’s work and using the same curvilinear model, Stein and colleagues15 tested newer suture techniques: the Strickland, Robertson, and modified Becker. All core sutures were performed with 4–0 Ethibond and 6–0 nylon for epitenon repair. Stein and colleagues15 demonstrate statistically significant increases in dorsal versus volar grasping strength with Kessler and Robertson repairs. No differences were found with the locking Strickland and modified Becker repairs. The four-strand techniques (Robertson and modified Becker) were also significantly stronger than the two-strand techniques (Kessler and Strickland). Wasserman and coworkers24 further demonstrated the modified Becker repair to be significantly stronger and tougher than the modified Kessler repair whereas allowing equally efficient glide in a dynamic human cadaveric model (Table 14-6).
SUTURE MATERIAL AND SIZE
Choice of suture material is an important factor in flexor tendon repair, which is reflected in efforts to determine the best tendon suture material. Few studies have investigated the range of suture materials available to the surgeon.25