2 What cancer is
How cells should work
A cell (Fig. 2.1) is the basic building unit of life. All animal cells are similar in their components, although they may have different functions. Similar cells are grouped together to create tissues which carry out specific functions. For example, there are four main types of tissue that make up the human body: muscle, epithelial, nervous and connective tissues.
Each cell contains a complete copy of our genome in the form of 23 separate pairs of chromosomes (one set from each of our parents) (Fig. 2.2). For each chromosome and each gene, we have two slightly different copies.
DNA is made up of individual molecules called nucleotides, which are in turn made up of a sugar (deoxyribose), a phosphate and a nitrogenous base. The DNA molecule is comprised of two chains of nucleotide bases, arranged in a double helix (Fig. 2.3). There are four bases which are grouped into two types: purines (adenine (A) and guanine (G)) and pyrimidines (thymine (T) and cytosine (C)). Each base is paired up with another base: A pairs with T and C pairs with G. Each base is a slightly different length which gives the double helix its twisted shape. The bases can occur in any sequence. It is the sequence of the bases that makes up the instruction, a bit like the words of an instruction manual. Depending on the sequence of the bases, a particular protein will be produced. The proteins in turn will enable the cell to function in a particular way, including cell replication.
Cell cycle
From the time of conception, all of our cells continue to multiply in order for us to grow into an adult. Once we reach adulthood our cells only divide when there is need to repair and replace old damaged cells and to reproduce. To do this, cells go through a process called the cell cycle (Fig. 2.4). The phases of the cell cycle are:
• G0: resting phase, not in cell division.
• G1 and G2: the cell builds up energy and prepares for the next stage.
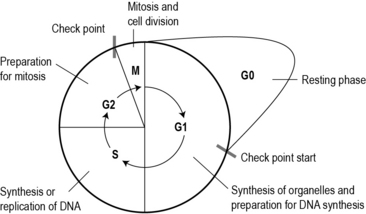
Fig 2.4 The cell cycle.
(Reproduced with permission from Kearney N, Richardson A (2005) Nursing patients with cancer: principles and practice. Churchill Livingstone (Fig. 5.4, page 78))
Normal cells only divide when they are needed, such as during growth (from conception to adulthood) and to replace old and damaged cells. This replication is carefully controlled by a number of checkpoints (see Fig. 2.4), which ensure that cell division only occurs when really necessary and occurs accurately. Certain genes control cell division, known as proto-oncogenes. These genes code for proteins and growth factors which signal to the nucleus of the cell to turn the cell cycle on, a bit like an accelerator of a car. There are four main groups of proteins produced by the proto-oncogenes: growth factors, growth factor receptors, signal transducers and nuclear proto-oncogenes and transcription factors.