Chapter 12 Visual Disturbances
This chapter describes several common visual disturbances likely to occur in psychiatric patients, including decreased visual acuity, glaucoma, visual field loss, and visual hallucinations (Box 12-1). It includes several causes of visual impairment in the elderly (Box 12-2). Whatever the cause of a visual disturbance, it will probably carry psychiatric comorbidity.
Box 12-1
Common Neurologic Causes of Visual Hallucinations
*Although visual hallucinations are likely to complicate almost any form of dementia, they are characteristic of dementia with Lewy bodies disease.
†Antiparkinson medications, such as levodopa-carbidopa (Sinemet), are more likely than Parkinson disease itself to produce hallucinations.
Evaluating Visual Disturbances
After determining the patient’s specific visual symptom, the physician’s initial examination typically includes inspecting the globe or “eyeball” (Fig. 12-1) and eyelids; assessing visual acuity, visual fields, and optic fundi; and testing pupil reflexes and ocular movement. Physicians must perform additional examinations for psychogenic blindness, visual agnosia, and other perceptual disturbances.
Physicians routinely measure visual acuity by having the patient read from either a Snellen wall chart or a hand-held card (Fig. 12-2). A person with “normal” visual acuity can read 3/8-inch (0.6-cm) letters at a distance of 20 feet (6.1 meters). This acuity, the conventional reference point, is designated 20/20. People with 20/40 acuity must be as close as 20 feet (6.1 meters) to see what a person with normal acuity can see at a distance of 40 feet (12.2 meters).
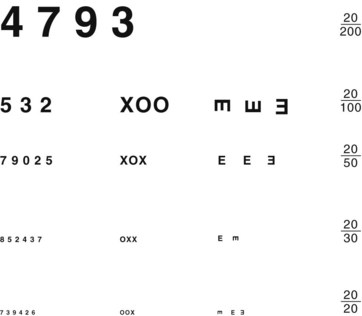
FIGURE 12-2 Physicians should hold this hand-held visual acuity chart 14 inches (36 cm) from patients and test each individual eye. The smallest line that they read without a mistake determines their visual acuity. For neurologic evaluations, patients should wear their glasses or contact lenses.
Optical Disturbances
The usual causes of myopia are optical rather than neurologic, such as a lens that is too “thick” or a globe that is too “long” (Fig. 12-3). Occasionally medicines cause myopia. For example, topiramate (Topamax), a widely prescribed antimigraine and antiepileptic drug (AED), may produce an acutely occurring but transient myopia. (Topiramate can also lead to angle closure glaucoma [see later].)
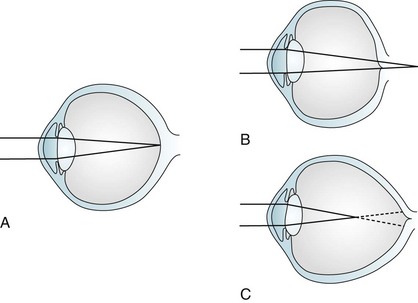
FIGURE 12-3 Image focusing in hyperopic (farsighted) and myopic (nearsighted) eyes. A, In normal eyes, the lens focuses the image on to the retina. B, In hyperopic eyes, the shorter globe or improperly focusing lens causes the image to fall behind the retina. C, In myopic eyes, the longer globe or improperly focusing lens causes the image to fall in front of the retina. Lenses can compensate for the refractive errors of hyperopia and myopia. Alternatively, laser or surgical “flattening” of the lens may correct myopia.
Because the parasympathetic nervous system mediates the accommodation reflex, many medications with anticholinergic side effects impair visual acuity for closely held objects (Fig. 12-4). These medicines – selective serotonin reuptake inhibitors (SSRIs), selective norepinephrine reuptake inhibitors (SNRIs), tricyclic antidepressants, and clozapine – produce blurred vision mostly because their substantial anticholinergic properties impair patients’ accommodation reflex. For example, duloxetine (Cymbalta) causes blurred vision in approximately 3% of patients; sertraline (Zoloft) and paroxetine (Paxil), 4%; and venlafaxine (Effexor) at 75 mg, 9%. This side effect may be unsuspected because these medicines can impair accommodation without producing other anticholinergic effects, such as dry mouth, constipation, and urinary hesitancy.
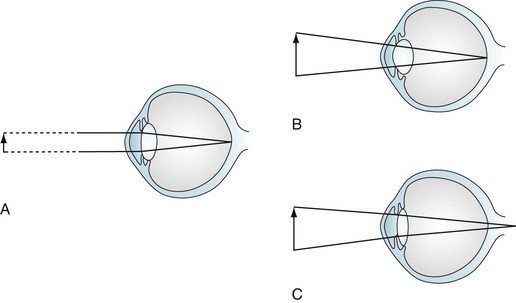
FIGURE 12-4 Accommodation and accommodation paresis. A, When looking at a distant object, parallel light rays are refracted little by a relatively flat lens on to the retina. B, Accommodation: when looking at a closely held object, ciliary muscle contraction increases the curvature of the lens, greatly refracting the light rays. C, Accommodation paresis: If the ciliary muscles are paretic, the lens cannot form a rounded shape. Its weakened refractive power focuses light rays from closely held objects behind the retina; however, parallel light rays from distant objects still focus on the retina. Therefore, accommodation paralysis blurs closely held objects but leaves distant ones distinct.
Abnormalities of the Lens, Retina, and Optic Nerve
Cataracts (loss of lens transparency) result from complications of old age (senile cataract), trauma, diabetes, myotonic dystrophy (see Chapter 6), and chronic use of certain medicines, such as steroids. In prolonged, high doses, phenothiazines and some second-generation neuroleptics may produce minute lens opacities, but ones rarely dense enough to impair vision.
Pigmentary changes in the retina can be a manifestation of injuries, degenerative diseases, diabetes, infection, or the use of massive doses of phenothiazines (Fig. 12-5). Among infants and children, nonaccidental head injury (child abuse), particularly violent head shaking or direct trauma, creates retinal hemorrhages. Other stigmata of repeated trauma – spiral fractures of the long bones, multiple skull fractures, and burns (see Chapter 22) – frequently accompany these retinal hemorrhages.
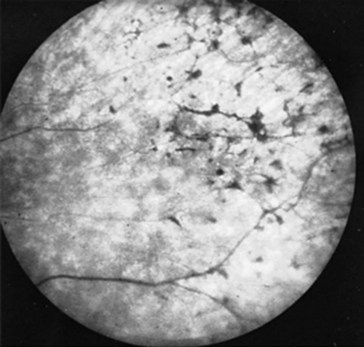
FIGURE 12-5 Massive doses of thioridazine (Mellaril) may induce retinal hyperpigmentation – described as “black bone spicules” or “salt and pepper.” Before these retinal pigmentary changes are visible on fundoscopic examination, patients may complain of blurred vision or impaired nighttime vision.
In 25% or more of Americans older than 65 years, the cells of the retina’s pigment epithelium, mostly in the macula, degenerate through a variety of mechanisms, including proliferation of the underlying blood vessels. When degeneration involves cells in the macula, a condition known as macular degeneration, it distorts patients’ critical central vision. Patients characteristically lose their reading ability. With their remaining peripheral vision, patients negotiate around their living areas. However, progressive deterioration deprives patients of all their eyesight. As with individuals who develop blindness from any cause, those beset by macular degeneration are at risk of losing their self-sufficiency, appearing to have cognitive dysfunction, and experiencing visual hallucinations (especially if they also have hearing or cognitive impairments [Box 12-1]).
Optic Nerve
Injuries of the optic nerves, which are projections of the central nervous system (CNS), result in visual loss that may be limited to a scotoma (an area of blindness [see Fig. 15-2]), but may encompass the entire visual field. In addition, because the optic nerves serve as the afferent limb of the light reflex, optic nerve injury also causes an afferent pupillary defect: When the examiner shines light into an eye with optic neuritis, both pupils fail to constrict; however, when the same light shines into the unaffected eye, both pupils normally constrict (see Fig. 4-2). With time, optic nerve injuries usually cause atrophy, revealed by the fundoscopic examination showing pallor of the optic head.
Optic nerve injuries may occur either as isolated conditions or ones accompanied by injuries of the cerebrum or other part of the CNS. One of the most common, inflammation of one or both optic nerves, optic or retrobulbar neuritis, causes sudden, painful visual loss (Fig. 12-6), as well as an afferent pupillary defect. In addition, they see “desaturated” colors with their remaining vision. For example, patients cannot appreciate the difference between fire engine red and brick red. In severe cases, they cannot distinguish red from green.
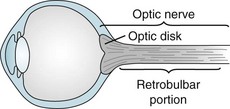
FIGURE 12-6 Neurologists consider the optic disk, which they can see on fundoscopic examination, to be the bulbar portion of the optic nerve. They consider the long segment of the optic nerve behind the eye its retrobulbar portion. Depending on where an inflammatory condition, such as multiple sclerosis, attacks the optic nerve, patients develop optic or retrobulbar neuritis. Visual loss, pain on eye movement, and color desaturation characterize optic and retrobulbar neuritis.
Of the many conditions that cause optic neuritis, demyelinating illnesses, particularly multiple sclerosis (MS) and its close relative neuromyelitis optica (NMO), are the most common (see Chapter 15). Most importantly, optic neuritis frequently precedes the appearance of other manifestations of MS and complicates the course of most cases. If an otherwise asymptomatic patient develops optic neuritis and the magnetic resonance imaging (MRI) shows two or more hyperintense lesions in the brain, that patient has greater than a 70% risk of developing MS. On the other hand, an otherwise asymptomatic optic neuritis patient who has no MRI lesions has only a 25% risk of developing MS.
An inflammatory condition of the arteries that supply the optic nerve, temporal or giant cell arteritis, often leads to ischemia of the optic nerves. Moreover, the arteritis tends to spread to the cerebral arteries (see Chapter 9). Typically affecting only people older than 65 years, temporal arteritis often first causes a mild to moderate, prolonged (weeks to months) illness featuring headache accompanied by systemic symptoms, such as malaise, prolonged aches, and pains. The number and variety of these initial nonspecific symptoms understandably lend the appearance of depression or a somatoform disorder. However, physicians should avoid missing this diagnosis because, unless they promptly treat the patient with high-dose steroids, it can result in blindness and strokes. Finding giant cells and other signs of inflammation on a temporal artery biopsy will confirm the diagnosis.
Leber hereditary optic atrophy, an illness attributable to a mitochondrial DNA mutation, also involves the optic nerves, but no other part of the CNS or the musculature (see Chapter 6). Most commonly affecting young males, it causes visual loss culminating in blindness in one and then, within months, the other eye.
Another classic example of simultaneous injury of both the optic nerve and cerebrum is an olfactory groove or sphenoid wing meningioma. This tumor compresses the near-by optic nerve (see Chapters 19 and 20) and burrows into the overlying frontal or temporal lobe. The cerebral damage can trigger complex partial seizures and cause cognitive decline and personality changes. At the same time, optic nerve damage causes optic atrophy and blindness in one eye.
Similarly, tumors of the pituitary region, such as adenomas or craniopharyngiomas, may also produce visual loss accompanied by psychologic changes. Unless detected and removed early, these tumors grow slowly upward to compress the optic chiasm and hypothalamus and downward to infiltrate the pituitary gland (see Fig. 19-4). Compression of the optic chiasm causes the pathognomonic bitemporal hemianopsia. Compression of the hypothalamus and pituitary causes headache and panhypopituitarism: decreased libido, diabetes insipidus, loss of secondary sexual characteristics, and sleep disturbances.
Glaucoma
In most cases, glaucoma consists of elevated intraocular pressure resulting from obstructed outflow of aqueous humor through the filtration angle of the anterior chamber of the eye (Fig. 12-7) – not from increased production of aqueous humor. Psychiatrists should recognize two common varieties – open-angle and angle closure – although only certain psychotropic medications occasionally produce the angle closure variety. If glaucoma remains untreated, it damages the optic nerve, causes visual field impairments, and eventually leads to blindness.
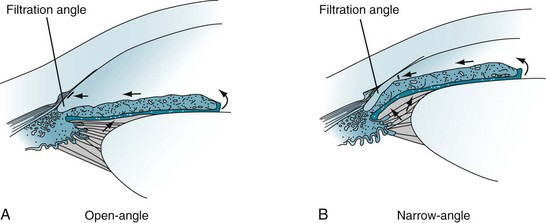
FIGURE 12-7 A, Open-angle glaucoma: The aqueous humor does not drain despite access to the absorptive surface of the angle. Impaired flow from the eye leads to gradually increased intraocular pressure (glaucoma). B, Narrow-angle glaucoma: When the iris moves forward, as may occur during pupil dilation, the angle is narrowed or even closed. Obstruction of aqueous humor flow leads to angle closure glaucoma.
Angle Closure Glaucoma
In angle closure glaucoma, which is also called closed-angle or narrow-angle glaucoma, intraocular pressure is usually elevated by impaired aqueous humor outflow at the filtration angle (see Fig. 12-7). In one variety, the fluid becomes trapped behind the iris. Patients with narrow-angle glaucoma usually are older than 40 years and often have a family history of the disorder, but they also frequently have a history of hyperopia and long-standing narrow angles. Few have had symptoms, such as seeing halos around lights, preceding an attack of angle closure glaucoma. In contrast to the relatively normal appearance of the eye in open-angle glaucoma, in acute angle closure glaucoma the eye is red, the pupil dilated and unreactive, and the cornea hazy. Moreover, the eye and forehead are painful, and vision is impaired.
Angle closure glaucoma is sometimes iatrogenic. For example, when pupils are dilated for ocular examinations, the “bunched-up” iris can block the angle (see Fig. 12-7, B). Likewise, medicines with anticholinergic properties, probably because they dilate the pupil, can precipitate angle closure glaucoma.
Cortical Blindness
Bilateral occipital cortex injuries can produce severe visual impairment, called cortical blindness. The underlying cause may be damage limited to the occipital lobes from bilateral posterior cerebral artery occlusions or trauma. Alternatively, extensive brain injury from anoxia, multiple strokes, or MS may cause cortical blindness along with other impairments. Reflecting occipital lobe damage, electroencephalograms (EEGs) characteristically lose their normal, posterior 8–12-Hz (alpha) rhythm. Whether the cortical blindness results from limited or generalized cortex injury, the pupils are normal in size and reactivity to light because all elements of the pupillary light reflex remain intact: the midbrain and optic and oculomotor nerves (see Fig. 4-2).
Anton Syndrome
The dramatic neuropsychologic phenomenon of Anton syndrome – blind patients explicitly or implicitly denying that they have lost all vision – characteristically complicates the sudden onset of blindness. Whether the blindness stems from a blast injury of both eyes, bilateral occipital lobe strokes, or other cause, the irrational response to blindness, rather than blindness itself, constitutes Anton syndrome. These patients typically respond, as those with anosognosia (see Chapter 8), by using denial as a defense. Sometimes they simply refuse to say that they have lost vision. Others blame external factors, like dim light, for their problem. Some may, if pressed, acknowledge visual loss but confabulate by “describing” their room, clothing, and various other objects. Anton syndrome allows blind patients to behave as though they still had normal vision and proceed to stumble about their room.
Visual Perceptual Disturbances
Agnosia
Visual agnosia is also a major component of the infamous, although uncommon, Klüver–Bucy syndrome. Neurosurgeons have produced this behavioral disorder in monkeys by resection of both anterior temporal lobes, which contain the amygdalae and components of the limbic system. The resulting limbic system damage produces visual agnosia so severe that the monkeys not only touch all objects, but they compulsively identify all objects by putting them into their mouth (“psychic blindness”). Their behavior can be repetitive, compulsive, and indiscriminate. When the Klüver–Bucy syndrome occurs in humans (see Chapter 16), they display a muted variation of psychic blindness, oral exploration, which consists of their placing inedible objects in their mouth, although only briefly, partly, and absent-mindedly.
Psychogenic Blindness
Similarly, tubular or tunnel vision defies the laws of optics that dictate that the visual area should expand with increasing distance (Fig. 12-8). Important exceptions to this general rule, however, sometimes occur when patients with migraine with aura have constriction of their peripheral vision and in some patients taking vigabatrin.
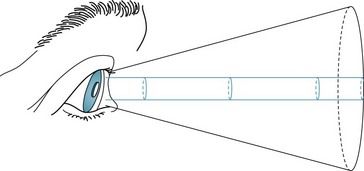
FIGURE 12-8 The area seen by a person normally increases conically in proportion to the distance from the object. In tubular or tunnel vision, which defies the laws of optics, the “visible” area remains constant despite increasing distance.
If clinical tests are inconclusive, EEG and other electrophysiologic testing may help. Alpha rhythm overlying the occipital lobes of patients at rest with their eyes closed, and loss of that rhythm when they open their eyes, indicates an intact visual system. However, because patients’ anxiety or concentration suppresses alpha activity, its absence is not as meaningful as its presence. In visual-evoked response testing, another noninvasive electrophysiologic test, visual system injuries produce abnormal potentials (see Chapter 15).
Visual Hallucinations
Visual hallucinations can originate in dysfunction of the frontal, temporal, or occipital cortex. Problems as diverse as toxic-metabolic encephalopathy, dementia-producing diseases, medication side effects, and structural lesions can produce them (see Box 12-1).
Seizures
Elementary partial, complex partial, or frontal lobe seizures (see Chapter 10) can produce visual hallucinations. Seizure-induced visual hallucinations tend to be stereotyped and brief, can be “seen” in both eyes, and may even appear in a hemianopic area. They range from simple geometric forms in elementary partial seizures to detailed visions accompanied by sounds, smells, thoughts, emotions, and, characteristically, impairment of consciousness, in complex partial seizures.
Migraine Aura
The “aura” in migraine with aura (previous termed “classic migraine”) consists of sensory disturbances – olfactory, sensory, or visual. In almost all cases, auras include stereotyped visual hallucinations (see Fig. 9-2). The majority consist of distinctive crescent scotomata or scintillating, patterned zig-zag lines (fortification spectra) that move slowly across the visual field for 1–20 minutes before yielding to a hemicranial headache. In a potentially confusing situation, visual auras sometimes represent the sole manifestation of migraine. In rare individuals, migraine aura consists of elaborate visual distortions, such as metamorphopsia, in which individuals and objects appear, to the patient, to change size or shape, as in the celebrated Alice in Wonderland syndrome.
Narcolepsy
As an element of the narcoleptic triad (see Chapter 17), visual hallucinations intrude into a patient’s partial consciousness. Narcoleptic-induced visual hallucinations are essentially dreams composed of variable, unpredictable – not stereotyped – intricate visions accompanied by rich thoughts and strong emotions. They tend to occur while patients fall asleep (hypnagogic hallucinations) or awaken (hypnopompic hallucinations). As with normal dreams, these hallucinations are associated with flaccid, areflexic paresis and rapid eye movements (REMs).
Neurodegenerative Illnesses
Visual hallucinations are also a hallmark of neurodegenerative diseases that cause dementia, particularly Alzheimer, Lewy bodies, and Parkinson diseases or their treatment (see Chapters 7 and 18). When they are manifestations of these disorders, hallucinations tend to be visually complex, have a paranoid aspect, and occur predominantly at night. As a clue to dementia with Lewy bodies disease, visual hallucinations occur frequently and begin early in its course. In contrast, when visual hallucinations complicate Alzheimer disease, they occur in its late stages. Hallucinations in Parkinson disease are usually partly medication-induced.
Sensory Deprivation
With seizures, migraine, or narcolepsy, appropriate treatment of the underlying condition will usually eliminate visual hallucinations. Some techniques override the sensory deprivation. Antipsychotics and occasionally AEDs may suppress hallucinations that complicate neurodegenerative illnesses, intoxications, and acute visual loss; however, physicians should avoid administering dopamine-blocking agents to patients with dementia with Lewy bodies (see Chapter 7).
Visual Field Loss
As in so much of neurology where anatomy determines destiny, the pattern of visual loss determines localization (Fig. 12-9). However, before reading further, nonneurologists must accept that the clinical presentation of cases is potentially confusing. Neurologists create drawings of visual fields viewed from the patient’s rather than from the physician’s perspective. Neurologists show the left eye’s visual field on the left side of the drawing, and the right eye’s on the right side. In contrast, radiologists persist in generating CTs and MRIs in a horizontally reversed pattern, “right is left.” With that frame of reference, neurologists follow several guidelines, as follows.
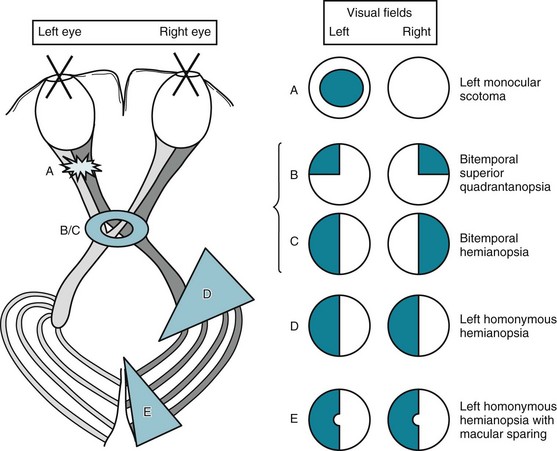
FIGURE 12-9 The visual pathway (see Fig. 4-1), which extends from the retina to the occipital cortex, is exquisitely vulnerable throughout its entire course. Lesions produce characteristic visual field defects. The defects, in turn, indicate the lesion’s location and give hints to its etiology and expectable associated findings. A, Optic nerve lesions typically give ipsilateral scotoma. B, Optic chiasm lesions, from below, when small (smaller ring), give superior bitemporal quadrantanopsia. C, When large (larger ring), optic chiasm lesions give bitemporal hemianopsia. D, Cerebral lesions that interfere with the anterior optic tract give contralateral homonymous hemianopsia. E, Lesions that interfere with the occipital cortex give contralateral homonymous hemianopsia, sometimes with macular sparing. Although the determination of visual fields serves as a highly reliable sign of localized neurologic disease, their display is one of clinical neurology’s most confusing exercises. In the standard manner, these sketches portray visual field defects as crosshatched areas from the patient’s perspective. For example, the sketch of the left homonymous hemianopsia (D) portrays the abnormal areas on the left side of each circle – as when the patient looks at the paper. The sketch of cerebral optic tract pathways portrays the tracts as though a picture had been taken from above the patient’s brain (see Fig. 8-4). In contrast, computed tomography (CT) and magnetic resonance imaging (MRI) scans traditionally show the brain in right-to-left reversal. A CT, for example, will show a left cerebral lesion on the right side of the study. Medical illustrations should include a notation to orient the reader.
Strokes in the frontal or parietal lobes usually cause physical and neuropsychological deficits in addition to a homonymous hemianopsia. In those strokes, ocular deviation, hemiparesis, hemisensory loss, and certain neuropsychological deficits typically accompany homonymous hemianopsia. For example, right-sided homonymous hemianopsia is often associated with conjugate ocular deviation to the left, right-sided hemiparesis and hemisensory loss, and nonfluent aphasia. Likewise, left-sided homonymous hemianopsia is often associated with conjugate ocular deviation to the right, left-sided hemiparesis and hemisensory loss, and left-sided anosognosia and hemi-inattention (see Chapter 8).
A rare but important situation is the homonymous superior quadrantanopia (Fig. 12-10). This visual field deficit may be the only physical manifestation of a contralateral temporal lobe lesion. Thus, it may represent the only interictal physical finding in patients who have complex partial seizures or the residua of a temporal lobectomy for trauma or intractable epilepsy.
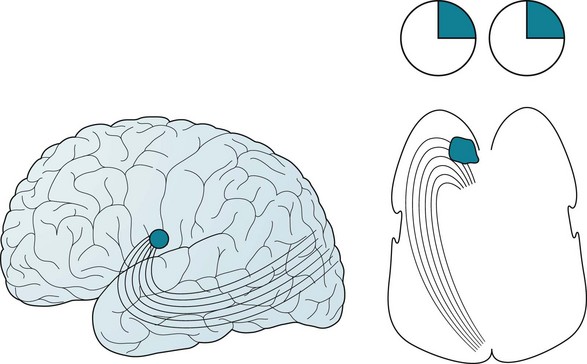
FIGURE 12-10 Left, Optic tract fibers sweep from the brainstem’s lateral geniculate body to the occipital lobe’s visual cortex. The tract’s inferior fibers first sweep anteriorly – through the temporal lobe’s anterior tip – before heading posteriorly. These fibers convey visual information from the contralateral superior temporal quadrant. Right, A large lesion or a surgical resection of the left temporal lobe has damaged the inferior optic tracts to produce a contralateral (right) superior quadrantanopsia.
Conjugate Eye Movement
In the normal, awake state, cerebral cortex (supranuclear) gaze centers (Fig. 12-11) innervate pontine (nuclear) gaze centers, which, in turn, innervate the nearby oculomotor, trochlear, and abducens cranial nerve nuclei (Fig. 12-12). Both eyes thus normally move together in a coordinated, paired (conjugate) manner. This system allows individuals to look (gaze) laterally, follow (pursue) moving objects, or deliberately shift their attention from one to another object (perform a saccade).
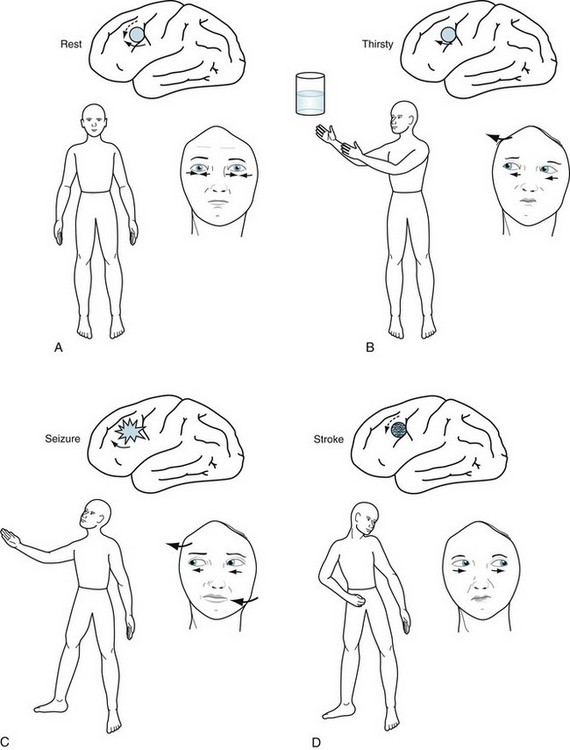
FIGURE 12-11 A, At rest, the eyes are midline because the impulses of each frontal lobe conjugate gaze center are balanced, each “pushing” the eyes contralaterally. Maintaining eyes in one position is an active process. B, Voluntarily increased activity of the left cerebral gaze center drives the eyes to the right (contralateral). C, Similarly, involuntarily increased cerebral activity, as occurs during a seizure, drives the eyes contralaterally. The seizure may also cause right arm and leg tonic-clonic activity. D, A stroke destroys the left cerebral gaze center, permitting the right center to push the eyes toward the lesion. It also destroys the cerebral motor strip, causing contralateral paresis. The eyes “looking” away from the hemiparesis characterize this common stroke.
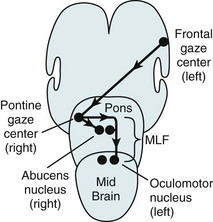
FIGURE 12-12 When looking to the right, the left frontal conjugate gaze center stimulates the right (contralateral) pontine gaze center, which neurologists also call the pontine paramedian reticular formation (PPRF). The pontine gaze center, in turn, stimulates the right (adjacent) abducens nerve nucleus and, upwards through the left medial longitudinal fasciculus (MLF), the left (contralateral) oculomotor nerve nucleus, which sits in the midbrain (see Fig. 15-3).
When individuals are conscious and looking about, their cortical, supranuclear gaze centers determine their gaze through their conjugate eye movements. When they are unconscious, tested in various ways, or beset by a variety of conditions that injure their cerebral cortex, their pontine, nuclear gaze centers assume control of their conjugate eye movements. In particular, when people dream, pontine centers generate REMs (see REM sleep, Chapter 17). Also, during both oculocephalic testing, in which the head is rocked back and forth, and cold caloric testing, in which neurologists irrigate the otic canals with cold water, labyrinthine stimulation of the pontine centers overrides any supranuclear innervation, and the nuclear stimulation drives the eye movement. In brain death, of course, neither cortical nor brainstem stimulation produces any eye movement.
Conjugate Eye Deviation From Cerebral Lesions
In an alert person, each cerebral conjugate gaze center continuously emits impulses that go through a complicated pathway to “push” the eyes contralaterally. With the counterbalancing activity of each cerebral gaze center, the eyes remain midline (see Fig. 12-11). When a person wants to look to one side, the contralateral cerebral gaze center increases activity. For example, when someone wants to look toward a water glass on the right, the left cerebral gaze center activity increases and, as if pushing the eyes away, both eyes turn to the right. If this person wished to reach for the glass, the left cerebral motor strip, which is situated posterior to the gaze center, would mobilize the right arm.
Partial seizures also increase activity of the cerebral conjugate gaze center. They push the eyes contralaterally and, because the seizures usually encompass the adjacent corticospinal tract, they push the head and neck contralaterally and produce tonic-clonic activity of the contralateral arm and leg (see Fig. 10-7).
Damage of the corticobulbar tracts, which innervate the extraocular muscle nuclei, abolishes voluntary conjugate gaze. As the most famous example, progressive supranuclear palsy (PSP) (see Chapter 7) consists of cerebral neurodegeneration that causes parkinsonism, dementia, and the illness’ pathognomonic finding – impairment of vertical and then lateral gaze. Within 3 years of the onset of the illness, PSP patients cannot voluntarily look upward or downward and then later to either side; however, neurologists can overcome the ocular paresis by performing oculocephalic testing (see Fig. 7-10).
Conjugate Eye Deviation From Brainstem Lesions
Each cerebral gaze center normally produces conjugate eye movements by stimulating a contralateral pontine gaze center, which neurologists also call the pontine paramedian reticular formation (PPRF). In contrast to the movement generated by the cerebral center, each pontine center pulls the eyes toward its own side (see Fig. 12-12). A stroke on one side of the pons thus allows the eyes to be pulled toward the opposite side. For example, if a stroke damaged the right pontine gaze center, the eyes would deviate to the left. Also, because this stroke would damage corticospinal tract in the right pons, the left arm and leg would be paralyzed. With a pontine lesion, neurologists say, “The eyes look toward the paralysis.”
When the pontine gaze center receives impulses from the contralateral cerebral conjugate gaze center, it activates one abducens nucleus and the contralateral oculomotor nucleus to produce conjugate lateral eye movement: the adjacent abducens (sixth cranial nerve) nucleus and, through the medial longitudinal fasciculus (MLF), the contralateral oculomotor (third cranial nerve) nucleus (see Figs 15-3 and 15-4). If the brain were to activate both abducens nuclei at the same time, both eyes would turn outward. If it were to stimulate both oculomotor nuclei, both eyes would turn inward.
MLF injury, as often occurs in MS and brainstem strokes, produces the MLF syndrome or internuclear ophthalmoplegia (INO). This condition, which spares the cranial nuclei and nerves, causes a classic pattern of ocular movement impairment identifiable primarily by inability of the eye ipsilateral to the lesion to adduct past the midline (see Chapter 15).
Saccades and Pursuit Movement
Neurologists examine saccades by asking patients to stare at an object 45° to one side and then suddenly shift their gaze to an object 45° to the other side. At the bedside, the primary abnormality is slowness. Other abnormalities are overshooting or undershooting (hypermetria and hypometria), irregular or jerky movements, and – a subtle one – initiating the saccade by blinking or a head jerk (Fig. 12-13).
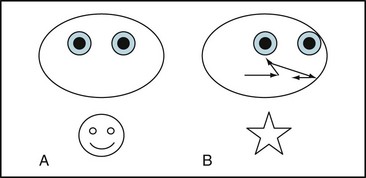
FIGURE 12-13 In testing for saccades, an examiner asks a patient with Huntington disease to shift his gaze from the examiner’s nose (A) to an object 3 feet (90 cm) to the left (B); however, after the patient’s eyes shift conjugately to the left, they jerk momentarily to the right, then resume their leftward movement, but overshoot the target. They finally come to rest on the object. Another characteristic of abnormal saccades is that they may force patients to initiate them by blinking, widely opening their eyelids, or tossing their head in the direction of the desired gaze.
While saccades are responsible for rapid shift of gaze from one object to another, pursuit or smooth pursuit is the slow continual ocular tracking of a single moving object, such as a bird in flight just above the horizon. In other words, unlike saccades, pursuits follow and maintain gaze on an object always in the visual field. The bedside test consists of asking the patient to follow the examiner’s finger as it moves horizontally at about 30°/second. The eyes should remain on the target and smoothly track the finger through a 6-second path. The primary abnormalities would be an irregular rather than a smooth path and jerky velocity. Numerous lesions and illnesses – even fatigue and inattention – impair smooth pursuit (Box 12-3).
Many conditions, including strokes, MS, tumors, neurodegenerative illnesses, and schizophrenia, can damage the intricate mechanisms that generate these movements. The responsible lesions may be located in the cerebral cortex, cerebellum, pons, or occasionally elsewhere in the CNS. Abnormal saccades are a signature of Huntington disease (see Chapter 18).
Diplopia
Oculomotor (third cranial) nerve injury results in diplopia that is greatest when the patient looks laterally. The three findings that neurologists rely on when attributing diplopia on lateral gaze to a third nerve injury are ptosis, lateral deviation of the eye, and a dilated pupil (Fig. 12-14). With third nerve palsy, diplopia will be most pronounced when the patient attempts to adduct the eye, i.e., bring it medially. For example, a patient with damage to the left oculomotor nerve will have difficulty adducting the left eye, and diplopia will be greatest upon looking to the right. Physicians should know that third nerve infarction from diabetes represents an important variation. While having all the other deficits of a third nerve palsy, a third nerve infarction from diabetes “spares the pupil,” i.e., the pupil remains reactive to light and the same size as its counterpart. Diabetic infarctions of the third nerve are also often painful.
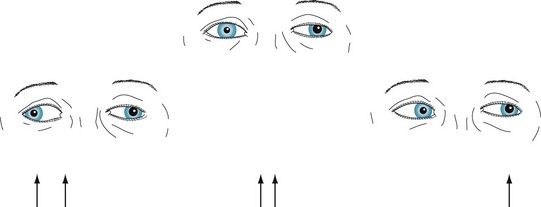
FIGURE 12-14 In the center picture, a patient with a left oculomotor (third cranial) nerve palsy looks straight ahead. The left upper lid is lower, the pupil larger, and the eye deviated slightly laterally. Because the eyes are dysconjugate, the patient sees two arrows (diplopia) when looking ahead. In the picture on the left, the patient looks to the right. Because the paretic left eye fails to cross medially beyond the midline (i.e., it fails to adduct), the eyes are more dysconjugate and there is greater diplopia. In the picture on the right, the patient looks to the left. The eyes are almost conjugate and there is little or no diplopia.
Abducens (sixth cranial) nerve injury also causes diplopia on looking laterally. In contrast to a third nerve palsy, the examination finds medial deviation of the affected eye at rest and inability of that eye to abduct. Also in contrast to a third nerve palsy, the examination shows neither ptosis nor pupil dilation (Fig. 12-15).
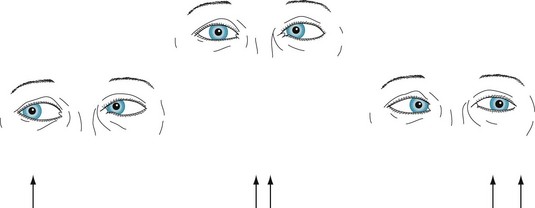
FIGURE 12-15 In the center picture, a patient with a left abducens (sixth cranial) nerve palsy looks straight ahead. The patient’s left eye is deviated medially. The eyes are dysconjugate, and the patient sees two arrows when looking ahead. In the picture on the left, the patient looks to the right. The eyes are conjugate, and the patient sees only a single arrow. In the picture on the right, the patient looks to the left. The paretic left eye fails to cross the midline laterally, that is, it fails to abduct. The exaggeration of the dysconjugate gaze increases the diplopia.
When myasthenia gravis, the classic neuromuscular junction disorder, causes diplopia, patients have fluctuating, variable, and asymmetric combinations of ptosis and paresis of various ocular muscles. However, no matter how severe the diplopia and ptosis, patients’ pupils are characteristically round, equal, and reactive to light (see Chapter 6).
Mitochondrial myopathies, such as progressive external ophthalmoplegia, may particularly affect the extraocular muscles because of their delicate nature and high metabolic demands (see Chapter 6). These disorders cause extraocular muscle paresis, including prominent ptosis, and sometimes diplopia. Although the paresis may mimic myasthenia gravis, mitochondrial myopathies progress insidiously, do not fluctuate, symmetrically weaken muscles, and usually spare facial, vocal, and limb muscles.
Horner Syndrome and Argyll Robertson Pupils
Contrary to a reasonable expectation that the brain would innervate eye muscles through short and direct pathways, the sympathetic tract follows a remarkably long and circuitous route (Fig. 12-16, A). Injury to the sympathetic tract leads to Horner syndrome: ptosis, miosis (a small pupil), and anhidrosis (lack of sweating) (Fig. 12-16, B). Given the vulnerability of injury during its long route, sympathetic tract damage leading to Horner syndrome can result from any of several widely separate injuries, including lateral medullary infarction (see Wallenberg syndrome; Fig. 2-10); cervical spinal cord injury; apical lung (Pancoast) tumor; and, because of a carotid artery dysfunction, cluster headache (see Fig. 9-4).
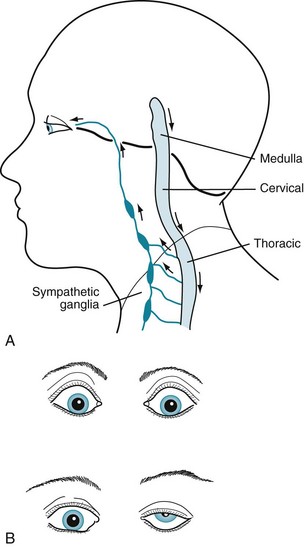
FIGURE 12-16 A The sympathetic nervous system originates in the hypothalamus, passes caudally through the brainstem, and descends further – into the cervical and then the uppermost thoracic spinal cord. Some sympathetic system neurons leave the thoracic spinal cord and, after making a hairpin turn, ascend to form ganglia adjacent to the cervical vertebrae. These postsynaptic neurons ascend further by successively wrapping themselves around the common carotid and then internal carotid arteries until they enter the orbit with the ophthalmic division of the trigeminal nerve (V1). Through these postsynaptic neurons, the sympathetic nervous system innervates the pupil dilator muscles, upper eyelid muscles, and facial sweat glands. B Top, Sympathetic nervous system activity retracts the eyelid, dilates the pupil, and causes sweating. These cardinal signs of the fight or flight response may also be induced by states of excitement, including amphetamine use. Bottom, Interruption of the sympathetic tract injury causes Horner syndrome – ptosis, miosis, and anhidrosis – on this patient’s left side. A subtle clue to Horner syndrome ptosis is the eyebrow elevation, which is an unconscious contraction of upper facial muscles to uncover the pupil.
In a different situation, the astute physician confronting a small pupil must also bear in mind that the real problem may be that the contralateral one is abnormally large. Causes of a dilated pupil include lesions that damage the parasympathetic supply of the pupil sphincter muscles, as well as an oculomotor nerve injury, because lack of parasympathetic innervation leaves the sympathetic innervation unopposed. An acquired benign variation, Adie pupil, exemplifies the pupil-dilating effect of depriving a pupil of its normal parasympathetic innervation (Fig. 12-17).
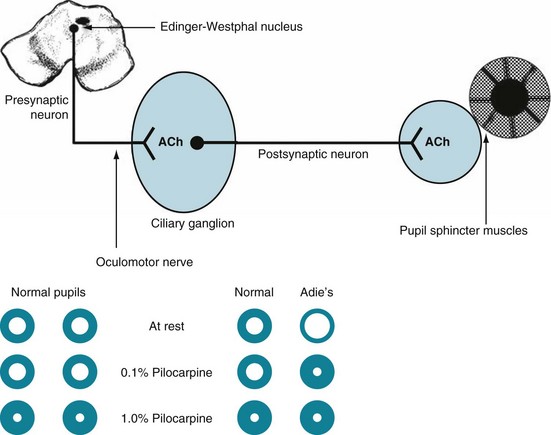
FIGURE 12-17 Top, The parasympathetic innervation of the pupil sphincter muscle, which constricts the pupil, originates in the Edinger–Westphal nucleus (see Fig. 4-2). That nucleus, adjacent to the third cranial (oculomotor) nerve nucleus, sends its parasympathetic fibers along with the oculomotor nerve. The parasympathetic fibers then separate and synapse in the parasympathetic ciliary ganglion. From there, postsynaptic fibers form a neuromuscular junction with the sphincter muscles. Acetylcholine (ACh) is the neurotransmitter in both synapses. Through this pathway, light and various other stimuli cause pupil constriction. Bottom, In the Adie pupil, which is typically a unilateral condition, the ciliary ganglion degenerates and the pupil, deprived of parasympathetic innervation, dilates. The loss of the ciliary ganglia also subjects the pupil to denervation hypersensitivity. In this case, the left (Adie) pupil suffers from a congenital impairment of the ciliary ganglion. Without the parasympathetic innervation, the left pupil dilates at rest in room light. In response to bright light, it would constrict only weakly, incompletely, and slowly. Diluted cholinergic eyedrops (0.1% pilocarpine), which have no effect on normal pupils, including this patient’s unaffected right eye, produce a brisk and long-lasting constriction of the Adie pupil. The reaction is exaggerated in the Adie pupil because its denervated postsynaptic neurons are overly sensitive, i.e., have denervation hypersensitivity. Normal pupils as well as the Adie pupil will constrict when physicians apply a more concentrated solution of pilocarpine (1%). As a surprising, seemingly unrelated, comorbidity of Adie pupil, patients often lose their quadriceps and Achilles deep tendon reflexes.
Balcer LJ. Optic neuritis. N Engl J Med. 2006;354:1273–1280.
Bengtzen R, Woodward M, Lynn MJ, et al. The “sunglasses sign” predicts nonorganic visual loss in neuroophthalmologic practice. Neurology. 2008;70:218–221.
Craig JE, Ong TJ, Louis DL, et al. Mechanisms of topiramate-induced acute-onset myopia and angle closure glaucoma. Am J Ophthalmol. 2004;137:193–195.
Giussani C, Roux FE, Bello L, et al. Who is who: Areas of the brain associated with recognizing and naming famous faces. J Neurosurg. 2009;110:289–299.
Holbrook JT, Jabs DA, Weinberg DV, et al. Visual loss in patients with cytomegalovirus retinitis and acquired immunodeficiency syndrome before widespread availability of highly active antiretroviral therapy. Arch Ophthalmol. 2003;121:99–107.
Kang SS, Dionisio DP, Sponheim SR. Abnormal mechanisms of antisaccade generation in schizophrenia patients and unaffected biological relatives of schizophrenia patients. Psychophysiology. 2011;48:350–361.
Pomeranz HD, Lessell S. Palinopsia and polyopia in the absence of drugs or cerebral disease. Neurology. 2000;54:855–859.
Schadlu AP, Schadlu R, Shepherd JB. Charles Bonnet syndrome: A review. Curr Opin Ophthalmology. 2009;20:219–222.
4. A 20-year-old soldier develops loss of vision in the right eye accompanied by retro-orbital pain, especially when looking from side to side. An ophthalmologist finds no ocular or neurologic abnormalities except for a decreased direct light reaction in the right pupil. The right pupil, however, constricts to light shone in the left eye. After 1 week, vision returns, except for a small central scotoma. Which is the most likely diagnosis?
7. A 79-year-old woman who has been blind since cataract surgery 5 years ago discloses that she has “seen” her children as babies, her long-deceased parents, and picturesque scenes. The visions last several minutes to an hour and occur at any time of the day. She is not frightened or inclined to act on them. She appreciates that the visions are hallucinations. An examination discloses no cognitive impairment or physical neurologic signs. What should be the next step?
9. Which one of the following statements is false regarding glaucoma?
10-c. The patient may have complex partial seizures and a right superior quadrantanopia as the result of a left temporal lobe lesion. Or f. Alternatively, the patient may have a left temporal lobe lesion, giving him aphasia and a contralateral superior quadrantanopia.
11-a. The patient has spinal cord, cerebellar, and right optic nerve injury, probably as the result of MS.
12-c. The patient may have complex partial seizures and a left superior quadrantanopia as the result of a right temporal lobe lesion.
13-b. The patient and her mother have preservation only of the central vision during daytime. If examination of her fundi showed clumping of retinal pigment, the diagnosis of retinitis pigmentosa would be certain. These visual fields might also be obtained from someone having tunnel vision.
14-e. The patient probably has a dominant hemisphere lesion, such as a stroke or tumor, giving a right homonymous hemianopsia.
15-d. The patient has a large pituitary tumor causing panhypopituitarism and bitemporal hemianopsia.
16. Associated musical hallucinations
17. Flashes of bright lights in the contralateral visual field
18. Associated olfactory hallucinations
19. Rotating blotches of color
20. Formed hallucinations with impaired consciousness
22. Throbbing unilateral headache
24. Simple blocks and stars of color
16-b; 17-a, c; 18-b, rarely c; 19-a; 20-b; 21-b, rarely c; 22-c; 23-c; 24-a, c; 25-b, c; 26-b, c.
37. A 70-year-old man suddenly sustained a right-sided homonymous hemianopsia, hemisensory loss, and mild hemiparesis. In addition, although he can both say and write the names of objects that he feels, he is unable to name objects that he only sees, even when they are presented to his left visual field. What is the name of this condition?
c. The patient has visual agnosia, a condition in which patients cannot process visually acquired information. Additional testing might reveal Gerstmann syndrome or alexia without agraphia (see Chapter 8) – conditions also resulting from posterior dominant hemisphere lesions. His problem is not aphasia: Once the examiner circumvents vision, by testing the patient’s writing and speaking, language function is shown to be normal. The lesion causing visual agnosia is usually in the left parietal and occipital region.
39–46. Match the patient’s condition (39–46) with the neuropsychologic disorder (a–j).
39. Cannot recognize familiar faces
40. Despite visual loss, willfully but erroneously describes hospital room and physician
41. After cardiac arrest, blindness with intact pupil light reflex
42. Cannot identify a red card, although able to match it to another red card and read a red-colored number on the Ishihara plates
43. Despite only a right homonymous hemianopsia, inability to read. Writing ability is intact
44. Congenital inability to read Ishihara plates
45. Inability to name common objects under any circumstances
46. Cannot name objects when seen, but can name them when described or grasped
a, d, e. Complex partial seizures, focal seizures that originate in the visual cortex, and migraine with aura cause the patient to experience the same – stereotyped – visual hallucination with each attack. In contrast, primary generalized – absences (petit mal) and tonic-clonic – seizures do not cause an aura or visual symptoms. Intoxications and withdrawal cause varied hallucinations – often tactile as well as visual – among other symptoms of delirium. Hypnagogic and hypnopompic hallucinations, which are essentially dreams that intrude into wakefulness (see Chapter 17), are variable.
49. A 35-year-old woman sought neurologic consultation because she has developed “droopy eyelids” and inability to look in any direction. Her symptoms persist evenly throughout the day. In lay terms, she explains that her sister, younger by 5 years, has begun to have the same facial appearance and paralysis of gaze. The patient has bilateral, symmetric ptosis and inability to move her eyes in any direction, but her pupils are round, equal, and reactive to light. She shows normal mentation, lower cranial nerves, muscle, and reflexes. Which is the most likely disorder?
d. Progressive external ophthalmoplegia, a mitochondrial myopathy, causes paresis of all the extraocular muscles, which produces ptosis and immobility of eye movement. Although the paresis mimics myasthenia gravis in that ptosis is prominent and pupil reaction to light are preserved in both conditions, progressive external ophthalmoplegia progresses insidiously and does not fluctuate, affects muscles symmetrically, and usually spares facial, vocal, and limb muscles. Neurologists confirm a clinical diagnosis of progressive external ophthalmoplegia by tests of mitochondrial DNA and myasthenia by tests for antiacetylcholine receptor antibodies, electromyograms, and Tensilon tests. Leber hereditary optic atrophy, which causes blindness, is another disorder attributable to a mitochondrial DNA mutation; most others involve muscles (see Chapter 6).
51. A 68-year-old retired well-known actor sought neurologic consultation because he has frequently fallen while descending stairs or walking off a curb. His friends and ex-wife thought that he was showing the effects of his well-known alcoholism. The neurologist found a paucity of facial and limb movements, rigidity of limbs, and loss of postural reflexes, but no tremor. He was unable to look in either vertical direction in response to requests, but when the neurologist flexed and extended his head and neck (rocked his head up and down), his eyes looked fully upward and then downward. Which is the most likely diagnosis?
c, e, f, g. Horner syndrome may result from lesions in the medulla, upper portion of the spinal cord (the cervical spinal cord), or autonomic nervous system in the chest – anywhere along the circuitous route of the sympathetic innervation of the pupil (see Fig. 12-16). In a related matter, neurologists no longer include “ophthalmoplegic migraine,” a condition in which paresis of the extraocular muscles accompanies a headache, as a variety of migraine. They now consider it a mononeuropathy.
c. The posterior cerebral arteries, which are the terminal branches of the basilar artery, perfuse the occipital lobes. Because the occipital lobes contain the visual cortex, occlusion of both posterior cerebral arteries causes cortical blindness. An occlusion of the left posterior cerebral artery that causes an infarction of the left occipital lobe and posterior corpus callosum (the splenium) may cause alexia without agraphia as well as a right homonymous hemianopsia (see Chapter 8).
55. A 33-year-old man claims to have double vision in his right eye after a motor vehicle accident. When he covers the right eye, the diplopia disappears, but when he covers the left eye, he has persistent diplopia. The visual acuity in the left eye is 20/20 and in the right eye 20/400. Visual fields are normal in the left eye but cannot be determined in the right eye because of the diplopia. Which three statements regarding his situation are true?
59. A 75-year-old man was shown a picture of his anniversary party that had been held when he was 50 years old. He recognized most friends and family members but could not identify the relationships between them. He could not recall the reason for the party despite a “Happy 25th Anniversary” banner in the background. He looked from one person to another, but he failed to survey the scene and was unable to direct his gaze. He was oriented and had good memory and judgment. His visual acuity and visual fields were within normal limits. Which is the best term describing his inability to comprehend the picture?
61. During her recovery from a lumbar laminectomy, a 35-year-old nurse complains of the sudden onset of poor vision in her right eye. That pupil is dilated and does not respond to light in either eye. Pilocarpine eye drops (1%) also fail to constrict the pupil. Her extraocular movements are full and the fundoscopic examination reveals no abnormalities. The remainder of the neurologic examination is normal. A CT, MRI, and lumbar puncture all produce normal results. The intraocular pressure is normal. A similar problem followed her hysterectomy the previous year. What is the most likely cause of her visual impairment?
64. A 72-year-old retired psychiatrist reported that suddenly and painlessly a gray curtain obscured vision in her left eye for 3 minutes. She assured herself that the vision in her right eye was normal by performing a visual field examination and reading newsprint. Which term most appropriately describes her symptom?
a. She experienced transient (fugax) loss of vision (amaurosis). By her testing each eye, she excluded a visual field cut (homonymous hemianopsia). Amaurosis fugax episodes are often caused by emboli that originate in the carotid artery, pass through the ophthalmic artery, and temporarily lodge in the retinal artery. When patients have this symptom, neurologists often evaluate them for carotid stenosis and prescribe antiplatelet agents, such as aspirin. Sometimes carotid artery surgery or stenting is warranted. Scotoma, which may appear to originate in one or both eyes, pertains to a loss of a portion of a visual field that usually has an oval or star shape and overlaps visual quadrants. Migraine with aura, optic neuritis, methanol intoxication, and retinal injuries are common causes of scotoma (see Figs 9-2, 12-9, and 15-2).
65. A 68-year-old man, a well-respected successful lawyer, remains personable, humorous, and a good ballroom dancer. Years ago he began to forget the names of acquaintances and some colleagues. He consulted an ophthalmologist because he has begun to fail to recognize the faces of friends, acquaintances, and historic figures, such as George Washington. However, his visual acuity is 20/20 with glasses. Which is the most likely condition?
66. A 43-year-old man, who was well known to the emergency room staff as a chronic alcoholic and “down on his luck,” seemed to say that he was seeing “snow” in both his eyes. His symptom was difficult to understand because of confusion, disorientation, and dysarthria, but, as best as the staff could determine, his vision was 20/200 even with his glasses. His visual fields were full to confrontation. His pupils were slow to react to light. He had nystagmus with no paresis of extraocular muscles. His gait was ataxic. The staff administered 100 mg intravenous thiamine. Blood tests showed a severe anion gap with profound metabolic acidosis and no ethanol. Which treatments should they administer in addition to the thiamine?
68. With the onset of her menopause, a 59-year-old woman begins to suffer from an increase in the frequency of her migraines from once a month to twice weekly. Her neurologist initiates preventive treatment with topiramate. Several weeks later, the woman reports bilateral visual impairment and eye pain. A consulting ophthalmologist finds that she has redness of the eyes, large pupils, and shallow anterior chambers. Which of the following conditions has most likely developed?