CHAPTER 61 “Very” Future Directions in Minimally Invasive Spinal Surgery
It’s even more difficult to call what I’m doing now surgery. When they opened the Techno-Suite 2 years ago, I took over the remote console pretty much full time (Fig. 61–1), and the residents worked the “Pit.” That’s the macho nickname for the sterile area. Compared with the old spinal surgery operating rooms I used to work in, the so-called Pit is pretty tame. Getting blood on the floor nowadays almost calls for an incident report, unlike back in the day, when you always saw the mop and bucket come out after a spinal fusion. Probably the biggest culture shift is the cast of characters needed to pull off a spinal case; the Pit is no longer the dominion of the solitary alpha male, perpetually irritated spinal surgeon, but rather looks like the clean room at NASA, where they assemble the Mars modules. Actually looking through the glass into the Pit now, I can’t even see a surgeon.
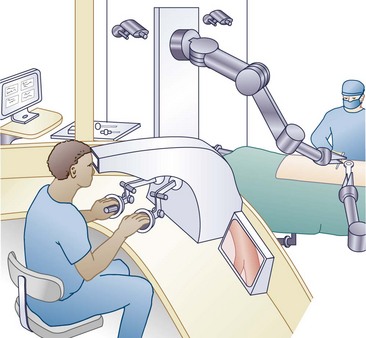
FIGURE 61–1 The attending surgeon operates the remote console while viewing the resident in the surgical area.