CHAPTER 17 Vascular supply and drainage of the brain
The brain is a highly vascular organ, its profuse blood supply characterized by a densely branching arterial network. It has a high metabolic rate that reflects the energy requirements of constant neural activity. It receives about 15% of the cardiac output and utilizes 25% of the total oxygen consumption of the body. The brain is supplied by two internal carotid arteries and two vertebral arteries that form a complex anastomosis (circulus arteriosus, circle of Willis) on the base of the brain. Vessels diverge from this anastomosis to supply the various cerebral regions. In general, the internal carotid arteries and the vessels arising from them supply the forebrain, with the exception of the occipital lobe of the cerebral hemisphere, and the vertebral arteries and their branches supply the occipital lobe, the brain stem and the cerebellum. Venous blood from the brain drains into sinuses within the dura mater (see Ch. 27). Acute interruption of the blood supply to the brain for more than a few minutes causes permanent neurological damage. Such ischaemic strokes along with intracranial haemorrhage are major contemporary sources of morbidity and mortality.
ARTERIAL SUPPLY OF THE BRAIN
INTERNAL CAROTID ARTERY
The internal carotid arteries (Fig. 17.1) and their major branches (the internal carotid system or ‘anterior’ circulation) supply blood to the majority of the forebrain. Some parts of the occipital and temporal lobes are supplied by branches of the vertebrobasilar system (see Fig. 17.5).
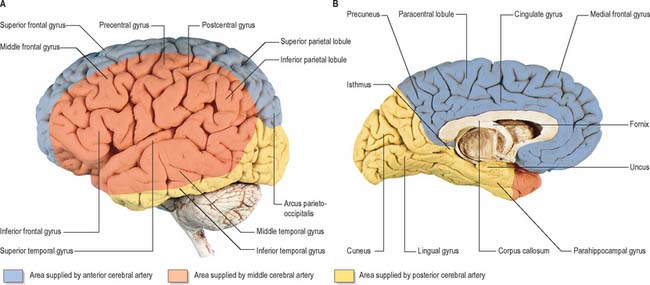
Fig. 17.5 The arteries supplying the left cerebral hemispheres. A, Lateral surface. B, Medial surface.
Cavernous part
The cavernous part of the internal carotid artery ascends to the posterior clinoid process. It turns anteriorly to the side of the sphenoid within the cavernous sinus and then curves up medial to the anterior clinoid process, to emerge through the dural roof of the sinus. The oculomotor, trochlear, ophthalmic and abducens nerves are lateral to it within the cavernous sinus (see Fig. 27.9). Occasionally, the two clinoid processes form a bony ring round the artery.
This part of the artery gives off a number of small vessels. Cavernous branches supply the trigeminal ganglion, the walls of the cavernous and inferior petrosal sinuses and the nerves contained therein. A minute meningeal branch passes over the lesser wing of the sphenoid to supply the dura mater and bone in the anterior cranial fossa and also anastomoses with a meningeal branch of the posterior ethmoidal artery. Numerous small hypophysial branches supply the neurohypophysis, and are of particular importance because they form the pituitary portal system (Fig. 21.11).
Intracranial part
Several preterminal vessels leave the cerebral portion of the internal carotid. The ophthalmic artery arises from the anterior part of the internal carotid as it leaves the cavernous sinus, often at the point of piercing the dura, and enters the orbit through the optic canal. The posterior communicating artery (Fig. 17.2, Fig. 17.3) runs back from the internal carotid above the oculomotor nerve, and anastomoses with the posterior cerebral artery (a terminal branch of the basilar artery), thereby contributing to the circulus arteriosus around the interpeduncular fossa. The posterior communicating artery is usually very small. However, sometimes it is so large that the posterior cerebral artery is supplied via the posterior communicating artery rather than from the basilar artery (‘fetal posterior communicating artery’); it is often larger on one side only. Small branches from its posterior half pierce the posterior perforated substance together with branches from the posterior cerebral artery. Collectively they supply the medial thalamic surface and the walls of the third ventricle. The anterior choroidal artery leaves the internal carotid near its posterior communicating branch and passes back above the medial part of the uncus. It crosses the optic tract to reach and supply the crus cerebri of the midbrain, then turns laterally, recrosses the optic tract, and gains the lateral side of the lateral geniculate body, which it supplies with several branches. It finally enters the inferior horn of the lateral ventricle via the choroidal fissure and ends in the choroid plexus. This small, but important, vessel also contributes to the blood supply of the globus pallidus, caudate nucleus, amygdala, hypothalamus, tuber cinereum, red nucleus, substantia nigra, posterior limb of the internal capsule, optic radiation, optic tract, hippocampus and the fimbria of the fornix.
ANTERIOR CEREBRAL ARTERY
The anterior cerebral artery is the smaller of the two terminal branches of the internal carotid (Fig. 17.3).
The two anterior cerebral arteries travel together in the great longitudinal fissure. They pass around the curve of the genu of the corpus callosum and then along its upper surface to its posterior end, where they anastomose with posterior cerebral arteries (Fig. 17.4). They give off cortical and central branches.
The cortical branches of the anterior cerebral artery are named according to their distribution. Two or three orbital branches ramify on the orbital surface of the frontal lobe and supply the olfactory cortex, gyrus rectus and medial orbital gyrus. Frontal branches supply the corpus callosum, cingulate gyrus, medial frontal gyrus and paracentral lobule. Parietal branches supply the precuneus, while the frontal and parietal branches both send twigs over the superomedial border of the hemisphere to supply a strip of territory on the superolateral surface (Fig. 17.5). Cortical branches of the anterior cerebral artery therefore supply the areas of the motor and somatosensory cortices that represent the lower limb.
Central branches of the anterior cerebral artery arise from its proximal portion and enter the anterior perforated substance (Fig. 17.3) and lamina terminalis. Collectively, they supply the rostrum of the corpus callosum, the septum pellucidum, the anterior part of the putamen, the head of the caudate nucleus and adjacent parts of the internal capsule. Immediately proximal or distal to its junction with the anterior communicating artery, the anterior cerebral artery gives rise to the medial striate artery which supplies the anterior part of the head of the caudate nucleus and adjacent regions of the putamen and internal capsule.
MIDDLE CEREBRAL ARTERY
The middle cerebral artery is the larger terminal branch of the internal carotid.
The middle cerebral artery runs at first in the lateral fissure, then posterosuperiorly on the insula, and divides into branches distributed to the insula and the adjacent lateral cerebral surface (Fig. 17.3, Fig. 17.4, Fig. 17.5). Like the anterior cerebral artery, it has cortical and central branches.
VERTEBRAL ARTERY
The vertebral arteries and their major branches (sometimes referred to as the ‘vertebrobasilar system’) essentially supply blood to the upper spinal cord, the brain stem and cerebellum and a significant but variable part of the posterior cerebral hemispheres (Fig. 17.6).
The vertebral arteries are derived from the subclavian arteries (see Ch. 30). They ascend through the neck in the foramina transversaria of the upper six cervical vertebrae and enter the cranial cavity through the foramen magnum, close to the anterolateral aspect of the medulla (Fig. 17.2). They converge medially as they ascend the medulla and unite to form the midline basilar artery at approximately the level of the junction between the medulla and pons.
The largest branch of the vertebral artery is the posterior inferior cerebellar artery (Fig. 17.6A). It arises near the lower end of the olive and then ascends behind the roots of the glossopharyngeal and vagus nerves to reach the inferior border of the pons. Here it curves and descends along the inferolateral border of the fourth ventricle before it turns laterally into the cerebellar vallecula between the hemispheres, and divides into medial and lateral branches. The medial branch runs back between the cerebellar hemisphere and inferior vermis, and supplies both. The lateral branch supplies the inferior cerebellar surface as far as its lateral border and anastomoses with the anterior inferior and superior cerebellar arteries (from the basilar artery). The trunk of the posterior inferior cerebellar artery supplies the medulla oblongata dorsal to the olivary nucleus and lateral to the hypoglossal nucleus and its emerging nerve roots. It also supplies the choroid plexus of the fourth ventricle and sends a branch lateral to the cerebellar tonsil to supply the dentate nucleus. The posterior inferior cerebellar artery is sometimes absent.
BASILAR ARTERY
The basilar artery is a large median vessel formed by the union of the vertebral arteries at the mid-medullary level (Figs 17.2, 17.3, 17.6). It lies in the pontine cistern, and follows a shallow median groove on the ventral pontine surface, extending to the upper border of the pons. It ends by dividing into two posterior cerebral arteries at a variable level behind the dorsum sellae, usually in the interpeduncular cistern.
The anterior inferior cerebellar artery (Fig. 17.3