Chapter 13 Ultrasound of the liver, biliary tract, and pancreas
Ultrasound Technology
Ultrasound is a high-resolution, low-cost imaging technique that uses no ionizing radiation. Its versatility and real-time imaging capability are also major advantages. Another unique advantage is Doppler ultrasound, which allows visualization of blood flow and assessment of flow dynamics. Ultrasound units are now smaller and more portable, so they are widely used in multiple medical settings, including the bedside, operative suite, emergency room, and in diagnostic and interventional radiology suites. Miniaturized high-resolution transducers also facilitate laparoscopic and endoscopic procedures (Berber et al, 2004; Machi et al, 2004).
Principles of Ultrasound Interpretation
Gray-scale sonography employs a pulse-echo technique to generate a two-dimensional display of the backscattered signal from insonated tissue. The intensity of the received echoes is recorded as brightness in the image. The strength of the echo at a tissue boundary is determined by acoustic impedence, defined as the product of tissue density and the speed of sound in the tissue. Sonographic scanners assume a uniform velocity of sound (1540 m/s) to compute the depth from which the received echo originated. Higher frequency sound has a shorter wavelength and therefore better spatial resolution, but a high-frequency sound beam attenuates more and has less tissue penetration. For this reason, lower frequency transducers are used to image structures deep within the abdomen, and higher frequencies are used to image more superficial structures (Zagzebski, 1996).
Gray-Scale Ultrasound Terminology and Artifacts
Lesions that appear dark relative to the background tissue are termed hypoechoic; those that are brighter than background are hyperechoic or echogenic (Fig. 13.1). Spaces that contain fluid do not contain internal interfaces, so no sound is reflected from within them; they appear uniformly black or anechoic. The ultrasound scanner normally compensates for the attenuation of sound that occurs with increasing depth within the tissue by amplifying echoes that return later from the far field. This is called time-gain compensation (TGC) or depth-gain compensation. TGC results in needless amplification of echoes from areas deep to pure fluid structures, because the acoustic pulse is attenuated to a lesser extent by fluid than by tissue. Such artificially bright areas beyond the fluid represent increased through transmission, or acoustic enhancement (Fig. 13.2). Although technically an artifact, acoustic enhancement is useful to characterize structures as cystic if they also lack internal flow on color Doppler.
Several other ultrasound artifacts also are used for diagnosis. At boundaries with large acoustic impedance mismatches, such as between tissue and air or tissue and bone, nearly all the energy in the incident beam is reflected; because none is transmitted, areas beyond the interface will not be visualized. A black acoustic shadow is thereby produced behind the object in the image (Fig. 13.3). Shadowing is characteristic of virtually all gallstones and greatly aids in distinction of gallstones from gallbladder polyps.
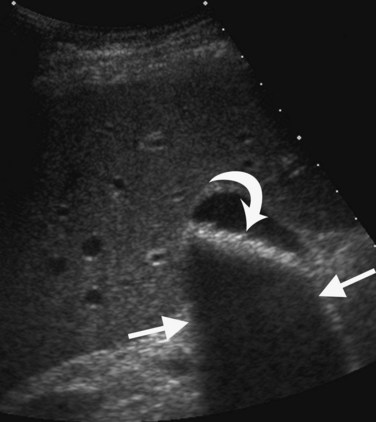
FIGURE 13.3 Gallstones (curved arrow) layering in the gallbladder produce an acoustic shadow (straight arrows).
Within fluid-filled structures such as the gallbladder, faint echoes are often seen paralleling the anterior surface. These echoes represent reverberation artifact, which occurs as the sound is repeatedly reflected between the highly echogenic anterior gallbladder wall and the transducer. Reverberation also accounts for comet tail artifact, a streak of tiny, bright, parallel bands tapering behind a punctate crystalline reflector, generated by sound echoing within the crystal itself. Comet tail artifact is a feature of adenomyomatosis of the gallbladder and emanates from cholesterol crystals trapped in dilated Rokitansky-Aschoff sinuses in the gallbladder wall (Fig. 13.4). Fluid-filled structures may also have artifactual echoes along the dependent wall that originate from off-axis portions of the ultrasound beam. This is termed side lobe artifact and may be distinguished from true debris by changing the angle of insonation or repositioning the patient (Feldman et al, 2009; Zagzebski, 1996).
Doppler Ultrasound
Three different Doppler displays are available (Fig. 13.5). Color Doppler displays show flow velocity and direction of flow toward or away from the transducer. A graphic or bar on the color Doppler image indicates the colors designated for flow toward and away from the transducer and the range of displayed velocities. Focal areas of high velocity that exceed the highest value on the Doppler setting produce a disorganized color signal; this is an artifact termed color aliasing that is useful to identify high-velocity flow, which may indicate vascular stenosis or encasement. Power Doppler displays amplitude of flow but not flow direction, and the signal is not dependent on the angle of insonation. Power Doppler is most useful to show areas of low flow and to demonstrate intralesional vascular patterns.
Spectral Doppler displays a graph of the entire range of velocities over time, rather than the mean velocity, as in a color Doppler image. When combined with gray-scale and/or color Doppler imaging in real time, it is possible to sample flow in specific blood vessels. For arterial flow, the peak systolic velocity and end-diastolic velocity indicate the strength of the inflow pressure and the downstream resistance. A useful metric for arterial waveform evaluation is the resistive index, defined as the difference between the peak systolic and end-diastolic velocities divided by the peak systolic velocity. A resistive index close to 1 implies nearly absent diastolic flow and high-resistance downstream. A lower than normal resistive index generally results from decreased systolic velocity and indicates impaired inflow to the region of interest. Hepatic venous waveform is usually phasic, with transmitted cardiac pulsation (see Fig. 13.5). When the hepatic veins or inferior vena cava (IVC) are narrowed, the waveform becomes flattened; this is a sensitive sign for venous compromise. Normal portal venous flow also has a phasic pattern that is decreased in conditions such cirrhosis and fatty infiltration (Colli et al, 1994).
Recent Technologic Advances
New techniques have been developed to coregister ultrasound, CT, and MRI images to facilitate lesion localization and to guide intervention or biopsy. Electromagnetic sensors placed near the scanning area and sensors attached to the ultrasound probe allow tracking of transducer position and orientation. Anatomic landmarks are identified on initial scanning and with navigation software, and these landmarks are coregistered with CT or MR images that have been downloaded into the ultrasound unit (Ewertsen et al, 2008). As the ultrasound scan is being performed in real time, the CT or MR images are reconstructed into a similar orientation plane (Fig. 13.6). Images may be displayed side by side or in a blended, overlapped format. In a study of contrast-enhanced ultrasound fused real time with CT, Jung and colleagues found that more lesions were identified with image fusion than with CT alone (Jung et al, 2009). Similar instrumentation allows global positioning system (GPS) navigation for needle tracking and volume acquisition. Acoustic force imaging to assess liver stiffness is another recent advance in development (see Songraphy of Diffuse Liver Disease below), and contrast-enhanced ultrasound is discussed in various sections on liver masses, detection, and characterization that follow.
Liver Ultrasound
Normal Liver Anatomy
The normal liver (see Chapter 1B) has a smooth surface and is uniform in echogenicity. Compared to adjacent organs, the liver is similar to or more echogenic than the kidneys and less echogenic than the spleen and pancreas. The superior aspect of the liver may have thin, linear indentations from diaphragmatic leaflets. Hepatic shape is variable. For example, a Riedel lobe may cause tonguelike inferior extension of the right lobe that may present as a palpable mass. Estimates of hepatic volume may be done with volumetric analysis (Treece et al, 2001; Wilson et al, 2009), but liver size is most commonly determined by a longitudinal image in the right midaxillary line. Normal liver length is reported to be less than 15.5 cm (Gosink et al, 1981). Others have used the right midclavicular longitudinal measurement with normal mean length of 10 cm (Niederau et al, 1983).
The Couinaud segmental anatomy of the liver is shown in Figures 13.7 and 13.8 (Couinaud, 1957; see Chapter 1B). Ultrasound images are obtained through available acoustic windows that avoid bone and air; this may vary the appearance of the liver compared with the standard axial, sagittal, and coronal planes of CT and MR, but the vascular landmarks that define the Couinaud segmental anatomy are well seen by ultrasound (Lafortune et al, 1991; Soyer et al, 1994). The hepatic veins and portal vein bifurcation are visualized with a subcostal transverse view; longitudinal images of the main portal vein, portal bifurcation, and hepatic artery are obtained best by intercostal approach, with the patient in the left lateral decubitus position. Transverse images of the portal confluence show the right portal vein and its bifurcation. The left portal vein in this plane courses transversely in the hilar plate for 3 to 4 cm before it extends into the umbilical fissure and gives off branches to the left hepatic segments. It is important to note that the horizontal portion of the left portal vein is extrahepatic and more readily available to the surgeon than the right portal vein.
Detection and Characterization of Focal Liver Masses
Gray-Scale Ultrasound of Focal Liver Masses
Conspicuity of liver lesions by gray-scale ultrasound is determined by lesion echogenicity relative to the liver. Small, markedly hypoechoic or hyperechoic lesions may be readily detected and characterized by ultrasound (Eberhardt et al, 2003), but masses that have echogenicity similar to adjacent liver may be more difficult to appreciate. On gray-scale ultrasound, liver masses are differentiated by internal architecture and are described as cystic, hypoechoic, and hyperechoic relative to the liver. Table 13.1 shows the common differential diagnoses within each of these categories (Charboneau, 2002; Middleton, 2002). The majority of cystic masses are benign, whereas most hypoechoic masses, with the exception of focal fatty sparing, are suspicious for neoplasm and require further evaluation, as they are clinically significant. Focal sparing often has geographic margins, typically is located in segment IV anterior to the portal bifurcation or adjacent to the gallbladder, and vessels are undisturbed. These distinctive features allow confident diagnosis (Fig. 13.9).
Hyperechoic masses include benign hemangioma, focal fat, and hepatic neoplasms such as hepatocellular carcinoma (HCC), adenoma, and metastases. Hemangiomas are brightly echogenic in the majority of cases and are sharply circumscribed. This appearance differs from malignant hyperechoic masses, which often have a hypoechoic halo. Any liver mass with a hypoechoic halo is suspicious for malignancy, and this sign has 86% positive predictive value and 89% negative predictive value for diagnosis of malignancy (Wernecke et al, 1992b).
Ultrasound Microbubble Contrast Agents for Evaluation of Liver Masses
Ultrasound microbubble contrast agents improve both detection and characterization of focal liver masses (Albrecht et al, 2003, 2004; Blomley et al, 1999; Bryant et al, 2004; Harvey et al, 2000; Quaia et al, 2004). First-generation contrast agents, such as SHU 508A (Levovist; Schering, Berlin), remain within the Kupffer cells after the vascular phase; when high mechanical index is applied to burst the bubbles, the liver enhances brightly, but metastases and other lesions without Kupffer cells appear dark. A multicenter study showed improved detection of individual metastases from 71% to 87%, and in seven of 80 patients, contrast-enhanced ultrasound showed more metastases than CT (Albrecht et al, 2003).
More recently, second-generation contrast agents and low mechanical index (LMI) scanning techniques have become available. At LMI settings, the microbubbles emit acoustic signals as they expand and contract in response to the ultrasound pulse, but they do not burst. Because the microbubbles stay in circulation, it is possible to image throughout arterial and portal phases. With LMI scanning, hypervascular tumors that enhance in the arterial phase are more easily detected. Lesion detection is also improved in the portal phase, because hepatic malignancies are hypoechoic during this phase (Wilson et al, 2006).
Contrast-enhanced ultrasound with second-generation contrast agents and LMI techniques allow assessment of vascular morphology, arterial enhancement relative to the liver, and duration of enhancement or “washout” during the portal venous phase (Wilson et al, 2006). These patterns of enhancement can be used to differentiate benign from malignant focal hepatic lesions as shown in the algorithm presented in Figure 13.10 (Brannigan et al, 2004; Ding et al, 2005; Wilson et al, 2006). Washout during the portal venous phase is highly correlated with malignant histology (von Herbay et al, 2009). In the portal phase, metastases and HCC are unenhanced relative to the liver, whereas benign hemangiomas and focal nodular hyperplasia (FNH) have sustained enhancement equal to or greater than the liver (Wilson et al, 2009; Quaia et al, 2004; Brannigan et al, 2004).
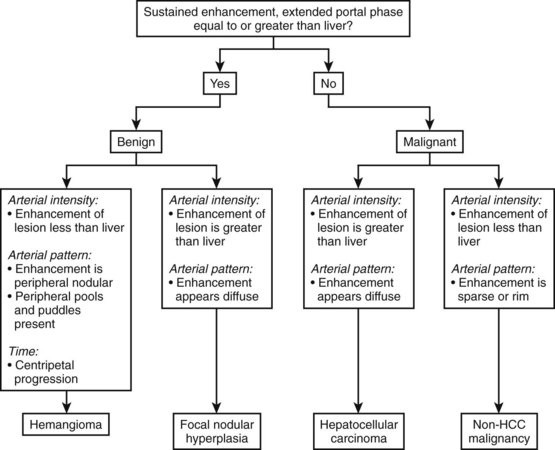
(Modified from Wilson SR, et al, 2006: An algorithm for the diagnosis of focal liver masses using microbubble contrast-enhanced pulse-inversion sonography. Am J Roentgenol 186:1401-1412.)
In a study of 452 liver lesions, contrast-enhanced ultrasound improved diagnostic accuracy for two readers from 49% to 85% and from 51% to 88%, and diagnostic confidence was increased from 0.82 to 0.97 and from 0.83 to 0.98, respectively (Quaia et al, 2004). A multiinstitutional study of 1349 patients with hepatic masses found that contrast-enhanced ultrasound had a diagnostic accuracy of 83% for all benign lesions, 82% for hemangiomas, and 87% for FNH. Diagnostic accuracy for malignant lesions was 96% overall, 91% for hepatic metastases, and 85% for HCC (Strobel et al, 2009).
Diagnostic performance of contrast-enhanced ultrasound has been compared to that of CT and MRI in several studies. There is high concordance for appearance in arterial phase imaging but less so in the portal phase, probably due to the fact the ultrasound contrast remains intravascular, but CT and MR contrast agents diffuse into the interstitium (Wilson et al, 2006). A multicenter study of 134 patients found that in comparison to CT and MRI, contrast-enhanced ultrasound was 30% more sensitive in recognition of malignancy, 16% more specific in exclusion of malignancy, and 23% more accurate (Trillaud et al, 2009).
Benign Liver Lesions
Cystic Masses
Benign hepatic cysts have smooth, thin walls, posterior acoustic enhancement, and no internal echoes (see Fig. 13.2). A partial, thin septum or puckered wall may be present. A confident diagnosis of simple hepatic cyst can be made on gray-scale ultrasound, and no further evaluation is required. Blood vessels and arteriovenous fistulas or aneurysms may appear cystic on gray-scale ultrasound, but these can be differentiated from simple cysts, because they will have internal flow on Doppler imaging. Lymphoma, which is very hypoechoic, may mimic a cyst rarely, but internal vascularity will be present on Doppler imaging. When cysts are innumerable and also present in the kidneys or pancreas, polycystic disease should be considered (see Chapter 69B).
Complex masses that contain fluid have internal echoes within the fluid, irregular or thickened walls, mural nodularity, and solid areas or calcification (Middleton, 2002). Hemorrhage, infection, and neoplasm should be considered in the differential diagnosis. Hemorrhagic cysts may have internal solid components, septations, and or debris layering in the dependent portion of the cyst, but on Doppler imaging, no internal vascularity is evident. Hepatic hematomas from trauma have variable appearance in the acute phase but become hypoechoic and cystic as they evolve (Korner et al, 2008; McGahan et al, 2006).
Abscess may be solid in appearance initially, but it then becomes cystic with debris; the wall is usually vascular (Fig. 13.11). Echinococcal cysts have variable appearance; they may be simple fluid-filled cysts, they may have undulated membranes from a rupture and detached endocyst, they may contain daughter cysts and/or echogenic material, and they may show calcification (Lewall et al, 1985). When hydatid cysts are infected, they lose their characteristic sonographic appearance and become diffusely hyperechoic.
Cavernous Hemangioma
Typical cavernous hemangioma, seen in 80% of cases, is a brightly echogenic homogeneous mass with a sharp margin (Fig. 13.12). Approximately 10% to 20% of hemangiomas are multiple (Charboneau, 2002). Multiple vascular interfaces within hemangiomas cause the increased echogenicity; the margins are well demarcated because histopathologically, only one cell layer is present at the junction with adjacent liver (Charboneau, 2002). Although hemangiomas are vascular, flow is extremely slow, and usually no Doppler signals are evident. Atypical hemangiomas, seen in 20% of cases, may have a thin echogenic rim and a lacy or granular central appearance from foci of collagen (see Fig. 13.12). Hemangiomas are often smaller than 3 cm, but some are giant hemangiomas that become symptomatic as a result of mass effect. Giant hemangiomas, those greater than 5 cm, often lack the characteristic ultrasound features because of central fibrosis, necrosis, and myxomatous degeneration. When the background liver becomes hyperechoic as a result of fatty infiltration, hemangiomas also may appear atypical and less echogenic than the liver. In such cases, contrast-enhanced ultrasound or CT may be useful (Bartolotta et al, 2007; Liu et al, 2009; see Chapter 79A).
With ultrasound contrast imaging at LMI settings, hemangiomas have sustained enhancement in the portal phase and on the arterial phase, enhancement intensity is usually less than the liver, and the pattern of enhancement is peripheral and nodular with puddling of contrast and centripetal progression, similar to CT (Wilson et al, 2006; Quaia et al, 2004; Brannigan et al, 2004; Fig. 13.13).
If the ultrasound shows the classic appearance of hemangioma, and the patient has no history of underlying liver disease or malignancy, then no further investigation is required (Leifer et al, 2000). A study of 213 patients with ultrasounds that showed a typical hemangioma appearance and without risk for hepatic malignancy found only one patient with malignancy on long-term follow-up and concluded that typical hemangiomas in low-risk patients do not require follow-up. This rule does not apply to patients with cirrhosis, hepatitis, or chronic liver disease who are at increased risk for HCC, nor does it apply to patients who already have malignancies. In a study of 2000 patients with cirrhosis, 44 had hemangioma-like lesions; on follow-up, half of these proved to be HCCs, and half were hemangiomas (Caturelli et al, 2001). Thus, in patients at risk for HCC, any echogenic lesion merits further evaluation and follow-up.
Focal Nodular Hyperplasia
The second most common benign hepatic neoplasm, FNH may be difficult to detect on gray-scale ultrasound, because it often has similar echogenicity to the liver, but subtle vascular displacement or contour abnormalities may draw attention to the lesion (Buetow et al, 1996; Hussain et al, 2004; Wilson et al, 2005; Fig. 13.14). FNH typically occurs in asymptomatic patients and is more common in women. Approximately 20% are multiple, and they may occur in association with hemangiomas (Nguyen et al, 1999; see Chapter 79A). FNH contains Kupffer cells, hepatocytes, and biliary structures but without portal triads and central veins found in normal hepatic lobules (Hussain et al, 2004). FNH is considered to be secondary to vascular malformation; fibrous septa radiate from a central scar, and tortuous feeding arteries are present.
On gray-scale ultrasound, FNH has variable echogenicity and lobulated smooth contour (see Fig. 13.14). A central scar may be evident, but it is usually better depicted on CT, MRI, or contrast-enhanced ultrasound. A key to ultrasound diagnosis of FNH is the characteristic power Doppler appearance of the tortuous feeding artery and spoke-wheel vascularity within the lesion. With ultrasound contrast imaging, the central scar is better seen, and the vascular pattern can be diagnostic. FNH is hypervascular on early arterial phase imaging with diffuse enhancement that is sustained into the early portal phase (Wilson et al, 2006; Kamaya et al, 2009; Dietrich et al, 2005; Ungermann et al, 2007; Brannigan et al, 2004; Quaia et al, 2004). Persistent portal phase enhancement differentiates FNH from malignant liver lesions (Kim et al, 2009; von Herbay et al, 2009; Fig. 13.15)
Hepatic Adenoma
Hepatic adenomas are rare benign tumors that usually occur in young women with a history of oral contraceptive use, but they can also be seen in men using anabolic steroids (see Chapter 79A). They are usually solitary (80%) but can be multiple in patients with type 1 glycogen storage disease or liver adenomatosis. Adenomas lack portal venous supply and bile ductules; they have disorganized plates of cells separated by dilated sinusoids that are perfused by arterial pressure, which predisposes to hemorrhage (Grazioli et al, 2001). On gray-scale ultrasound, adenomas appear echogenic, because adenoma cells have a high lipid content. Internal hemorrhage within adenomas may produce cystic areas, and calcification may also be present (Grazioli et al, 2001). Spectral Doppler flow may have a continuous waveform (Golli et al, 1994; Bartolozzi et al, 1997). Contrast ultrasound of hepatic adenomas more often shows centripetal or mixed filling during the arterial phase; this pattern differs from FNH, which has spoke-wheel vascularity. On portal phase imaging, adenomas are variable, but most commonly they are isoechoic or slightly hypoechoic relative to the liver (Bartolotta et al, 2007; Kim et al, 2006, 2008; Ricci et al, 2008).
Malignant Liver Neoplasms
Hepatocellular Carcinoma
HCC (see Chapter 80), one of the most common malignancies worldwide, usually arises in the background of cirrhosis and has poor survival (Collier et al, 1998; El-Serag et al, 1999). Chronic hepatitis B and C infection accounts for the high prevalence of HCC in Southeast Asia, sub-Saharan Africa, and the Mediterranean, and the increased prevalence of these viral infections has contributed to rising incidence of HCC in the United States (Caturelli et al, 2003; El-Serag et al, 1999).
Ultrasound in combination with serum α-fetoprotein (AFP) is used to screen for HCC in high-risk patients, and ultrasound-guided biopsy can be used for diagnosis with 90% accuracy (Caturelli et al, 2004; Collier et al, 1998; Sato et al, 2009; Tong et al, 2001). In patients with elevated AFP, ultrasound was found to have 86% sensitivity, 82% specificity, 55% positive predictive value, and a false-negative rate of only 4% (Mok et al, 2004). A study comparing screened versus unscreened patients with cirrhosis showed improved median survival (17 months vs. 12 months) and improved survival rates at 1, 3, and 5 years for HCC arising in the screened Child-Pugh class B patients, but not in the class C patients (Trevisani et al, 2007). Chan and colleagues (2008) compared survival of HCC arising in screened versus unscreened groups during two different 7-year time periods ending in 1997 and 2004. Long-term survival was better in the screened group and, as a result of improved surgical treatment, the proportion of patients undergoing curative resection increased from 51% to 68% in those two time periods.
On gray-scale ultrasound, HCC may be solitary, multiple, or diffuse, and it has a propensity for venous and bile duct involvement (Wilson et al, 2005). Small HCC tumors (<5 cm) are often hypoechoic but may be hyperechoic from fatty metamorphosis (Choi et al, 1993; Caturelli et al, 2001). Larger HCC tumors are often inhomogeneous because of liquefaction necrosis and fibrosis (Tanaka et al, 1983), and sonographic depiction of HCC may be limited in the setting of severe cirrhosis (Bennett et al, 2002). Thrombosis of portal and/or hepatic veins is frequent, seen in 30% to 60% of patients (Wilson et al, 2005; Fig. 13.16). Tumor thrombus usually expands the vein, and Doppler interrogation may reveal hepatofugal arterial flow within the thrombus, a pathognomonic finding (Shah et al, 2007; Lencioni et al, 1995; Giorgio et al, 2004; Tanaka et al, 1993). HCC may also extend into the bile ducts and cause biliary obstruction or hemobilia (Kojiro et al, 1982).
HCC is hypervascular with dysmorphic arteries and high-velocity flow on Doppler imaging (Koito et al, 1998; Tanaka et al, 1990). Microbubble ultrasound contrast imaging improves HCC characterization and is highly concordant with CT findings, but contrast-enhanced ultrasound is best at identifying lesions greater than 2 cm (Quaia et al, 2007; Giorgio et al, 2004; Inoue et al, 2009; Jang et al, 2008). Moderately differentiated HCC is hypervascular in the arterial phase, whereas well-differentiated and poorly differentiated HCC may have a more variable appearance. Observation during the portal phase should be extended, as many HCCs have delayed washout for up to 300 seconds (Jang et al, 2007; Fig. 13.17)
Fibrolamellar Carcinoma
Fibrolamellar HCC, a variant of HCC with better prognosis, characteristically presents as a large solitary mass in an adolescent or young adult without liver disease, and AFP levels are usually normal. On ultrasound, fibrolamellar HCC is well defined, lobulated, and has variable echogenicity. Calcification may also be present (Chung et al, 2009). A central scar may be visible on gray-scale ultrasound in a third to two thirds of patients, but scars are better seen with contrast-enhanced ultrasound (Smith et al, 2008; McLarney et al, 1999). Regional lymphadenopathy is common.
Liver Metastases
Liver metastases (see Chapter 81C) are multiple in 98% of patients and are found in up to 50% of cancer patients at autopsy (Wilson et al, 2005; Middleton, 2002). Liver metastases are frequently detected by gray-scale ultrasound, but CT is the preferred modality to determine extent of disease, number of hepatic lesions, and extrahepatic disease. Response to therapy is also better evaluated by CT, because treatment-related fatty infiltration may obscure lesions that have diminished in size or changed echogenicity as a result of chemotherapy.
On ultrasound, hepatic metastases frequently have a hypoechoic halo that corresponds to fibrosis, compressed sinusoids, and tumor neovascularity surrounding the metastatic deposit (Wernecke et al, 1992a; Kruskal et al, 2000; Fig. 13.18). Uniformly hypoechoic lesions are the second most frequent sonographic appearance of metastases (Middleton, 2002; Fig. 13.19